ABSTRACT
The pathogen Erysiphe cruciferarum is an increasingly serious threat to cruciferous oilseed crops worldwide. In the present study, the severity of powdery mildew was evaluated on spring oilseed rape (Brassica napus) and five alternative oilseed crop species (B. juncea, B. nigra, Sinapis alba, Raphanus sativus, Eruca sativa). The main aim of the study was to identify plant species that are less susceptible to powdery mildew for possible application as cover crop, in trap cropping or seed production. Powdery mildew assessments were conducted in two growing seasons that were characterised by unusually warm and dry conditions for northern temperate climatic conditions. In both growing seasons, a heavy powdery mildew infection (over 80%) was recorded on B. napus and B. nigra plants, indicating that E. cruciferarum is an important pathogen for oilseed crops in northern Baltics. However, three other cruciferous species S. alba, E. sativa and R. sativus showed full resistance to powdery mildew, and thus, even under strong powdery mildew pressure, they can be cultivated without pesticide application in northern Baltic conditions. We suggest that in changing climatic conditions powdery mildew is expected to become a more serious problem in cruciferous oilseed crops in northern Europe, especially under intensive short-rotation farming practices.
Introduction
Oilseed cruciferous crops, mostly oilseed rape (Brassica napus L.) and turnip rape (Brassica rapa L. subsp. oleifera (DC.) Metzg.) have been important cash crops for Estonian farmers from the end of the 1990s. However, exploitation of short crop rotation has caused serious problems especially for spring oilseed rape and turnip rape with high pressure of difficult-to-control soilborne diseases.
Impact of simultaneously or sequentially occurring stressors on plants such as herbivory or pathogen attack accompanied by hot and dry weather or limited availability of nutrients is common (Stavrinides et al. Citation2010; Sarasketa et al. Citation2016; Velásquez et al. Citation2018; Trejo-Téllez et al. Citation2019). Thus, great emphasis is placed on the breeding of plant cultivars and genetic engineering of plants with the aim of obtaining plants resistant to hot and dry growing periods while maintaining their nutritional value (Hossain et al. Citation2013; Kissen et al. Citation2016). The stress tolerance of plants is regulated by phytochemicals and in the case of cruciferous plants, particular attention is paid to glucosinolates (Antonious et al. Citation2009). In addition to their antifungal properties, glucosinolates mediate various metabolic processes starting from stomatal closure, sulphate assimilation, heat stress – and oxidative stress response (Hossain et al. Citation2013; Kissen et al. Citation2016).
Powdery mildew (Erysiphe cruciferarum Opiz ex L. Junell) is distributed worldwide, however, the severity of disease differs markedly between regions, growing seasons and crop species (Sadowski et al. Citation2002; Desai et al. Citation2004), reflecting the cultivation practices of cruciferous crops and climatic conditions. Severe powdery mildew attacks have been also reported on oilseed rape crops in India (Desai et al. Citation2004), Poland (Sadowski et al. Citation2002), Turkey (Mert-Türk et al. Citation2008), U.S.A. (Karakaya et al. Citation1993) and these infections can cause yield losses as high as 30% (Penaud Citation1999). Powdery mildew is an emerging problem in Korea (Kim et al. Citation2013; Cho et al. Citation2016) and in Australia (Uloth et al. Citation2016). Less frequent occurrence of E. cruciferarum in cooler climates has been associated with its relatively high thermal requirements (Pandey et al. Citation2017). Furthermore, while in warmer climates the infections can occur year-round, in seasonal climates, E. cruciferarum overwinters as resting spores on plant tissues or in soil (Koike et al. Citation2006). Nevertheless, climate change has resulted in poleward moments of many major pathogens (Elad and Pertot Citation2014; Velásquez et al. Citation2018), and thus, the spread of E. cruciferarum might drastically increase in the future.
In Estonia, E. cruciferarum has been never studied and published before. The first registered in the herbarium collection of Estonian University of Life Sciences on Brassica napus in 1959 (identified in 1999), B. juncea in 2002, on B. rapa in 2003 and on several wild host species since 1959 (Phytopathogenic Fungi Collection at Institute of Agricultural and Environmental Science of the Estonian University of Life Sciences, Tartu, Estonia, https://elurikkus.ee/generic-hub/occurrences/search?q=lsid:147053&lang=et#records [Accessed 29 Aug 2019]). Recent observations suggest that E. cruciferarum has become more frequent and is currently a common disease in Estonian spring oilseed rape fields (E. Runno-Paurson personal observations), and under favourable conditions, it can cause significant damage to foliage and buds. However, the development of infection by E. cruciferarum and susceptibility of different cruciferous crops to the disease has not been published in Estonia and all Baltics, except by Bankina et al. (Citation2012), where reported that powdery mildew is a minor disease on oilseed rape in Latvia. Under intensive cultivation, powdery mildew might become under favourable conditions a more serious problem in cruciferous oilseed crops in the northern Baltics. The pathogen E. cruciferarum survives on overwintering crops and volunteers (Koike et al. Citation2006). Thus, with a short crop rotation interval it is very important that plants for cover crop, trap cropping, lodging culture, etc. are not susceptible to powdery mildew disease, and must studied comprehensively before harnessing.
Given the lack of information about powdery mildew (E. cruciferarum) susceptibility on oilseed crops in northern Baltics, a field trial experiment was carried out with six different cruciferous oilseed crops to evaluate resistance to powdery mildew. The species included were spring oilseed rape (B. napus subsp. oleifera) and five alternative oilseed crop species (B. juncea, B. nigra, Sinapis alba, Raphanus sativus, Eruca sativa). The purpose of this experimental trial was to find plants that are less susceptible to powdery mildew for possible application as cover crop, in trap cropping or in seed production. We hypothesised that alternative cruciferous crops are most resistant to powdery mildew than spring oilseed rape.
Materials and methods
Field experiment
Powdery mildew (E. cruciferarum) occurrence was evaluated in 2010 and 2011 growing seasons on the plants of six different cruciferous plant species in the experimental field of the Estonian University of Life Sciences in 2010 and 2011, Eerika, Tartu County, Estonia (58°21′N, 26°39′E). The plants were grown in a randomised complete block design with three replicates of each of the following species: spring oilseed rape (Brassica napus L. cv. ‘Maskot’), mustard green (brown mustard) (B. juncea (L.) Czern. cv. ‘Jadrjonaja’), black mustard (B. nigra (L.) W. D. J. Koch), ruccola/arugula (Eruca sativa subsp. sativa (Mill.) Thell. (=E. sativa) cv. ‘Poker’), oilseed radish (Raphanus sativus L. var. oleiformis Pers. (=R. sativus) cv. ‘Bille’)) and white mustard (Sinapis alba L. cv. ‘Branco’). In both years, seeds were obtained from the seed collection of the Estonian University of Life Sciences. The size of each plot was 1 × 5 m as recommended by Kalischuk and Dosdall (Citation2004). To minimise inter-plot interactions, there was an 1 m wide buffer zone of bare soil around each plot. The whole experimental field was surrounded by a hay meadow. The seeds were sown on 12 May in 2010 and 9 May in 2011, at 250 seeds per m2. Seed germination percentage was similar for all species and years, and no differences in plant density (on average 200 plants per m2) between plots were noticed. In both years, the same standard crop management practices were used in all trial plots. Fertilisers and pesticides were not used. The plant growth stage (BBCH) was assessed using the decimal code system of Lancashire et al. (Citation1991).
Weather conditions
In 2010, the air temperature (Rõhu Weather Station present at the field site) in May and June were similar to the long-term (48-year) average, but in July, the temperature was 4.7 °C higher than the long-term average (). The rainfall in 2010 was similar to the long-term average in June, but in July (36.0 mm) and at the beginning of August (13.4 mm for the first two weeks of August) it was much lower than the long-term average (48-year averages of 70.6 mm for July and 33.3 mm for the first two weeks of August). In 2011, the temperature in May was similar to the long-term average, but June was hotter by 1.9°C and July hotter by 2.4°C (). The rainfall in May 2011 was similar to the long-term average, but much lower in June, July and the beginning of August (). In the third ten-day period of July, the warm conditions (with temperatures over 30°C for more than 10 days) were most favourable for powdery mildew infection. The measurement times were scaled into a probability range [0%, 100%] (Roostalu Citation2008). The cumulative probability of occurrence of certain average temperature (°C) and precipitation (mm) conditions was calculated for the pertinent study periods and corresponding average temperature and precipitation values for the growing season 2010 and 2011.
Table 1. Average monthly temperatures (°C) and precipitation (mm) at the Eerika experimental field during the vegetation period and the corresponding long-term averages (1964–2019).
Powdery mildew assessment
The occurrence of powdery mildew was assessed from July 12 to August 2 in 2010 (four observations) and from July 15 to August 5 in 2011 (four observations), at GS 58–63 to GS 85–89 (). Powdery mildew assessments were made under natural infection conditions as a percentage of total foliage attacked once each week, until the culmination of the disease. In all species in each of the replications, 10 randomly selected plants per plot were assessed for powdery mildew infection. Powdery mildew infection on test plants was assessed according to the 0–100% scale (EPPO Bull Citation2003). The area under the disease progress curve (AUDPC) was calculated from the date of the first occurrence of powdery mildew until the last observation of the disease in the trial according to Shaner and Finney (Citation1977) using the following formula: ΣR = n[(Ri +1 + Ri)/2)] (ti+1 – ti), where Ri is the disease severity (percentage leaf surface blighted) for the previous (ith observation), and Ri+1 the severity for the current observation and ti and ti+1 are the corresponding times of measurements (day of year), and n is the total number of observations.
Table 2. Growth stages (GS) of studied cruciferous species at each observation date in 2010 and 2011.
Data analysis
Statistical analyses were carried out using Statistica 13 (Quest Software Inc, Aliso Viejo, CA, U.S.A.). Differences in the severity of powdery mildew infection in dependence on year, cruciferous crop species and their interaction were tested by ANOVA. Both factors ‘year’ and ‘species’ were treated as fixed categorical variables. Tukey HSD post-hoc tests (P = 0.05) were applied to separate the differences among the means between years and cruciferous crop species. The level of statistical significance for all effects was P = 0.05 except when noted.
Results
In 2010, the average air temperature was extreme for 10 days before the powdery mildew symptoms were recorded and the unusual weather conditions continued during the whole observation period ((A−D). In fact, within the long-term weather record (1964−2011), the average air temperature for the dates corresponding to the study period has exceeded that measured in 2010 in only 4 years. The temperatures were extremely high during the period from the infection and the following 14 days (12–26 July) as indicated by a very low probability of hotter weather according to the available weather record for 48 years ((B, C)). The amount of precipitation before the infection and during the observation period was low (29.6−49.1% probability of drier conditions during 48 years period; (A−D)).
Figure 1. Cumulative probability of occurrence of certain average temperature (°C) and precipitation (mm) conditions at the Eerika experimental field according to long-term (1964–2011) weather data. The cumulative probability was calculated for the pertinent study periods and corresponding average temperature and precipitation values for the growing season 2010 are also shown. The relevant time periods as: A – for 10 days before first sampling (02.07–11.07), B – for (12.07–19.07), C – for (20.07–25.07) and D – for (26.07–02.08).
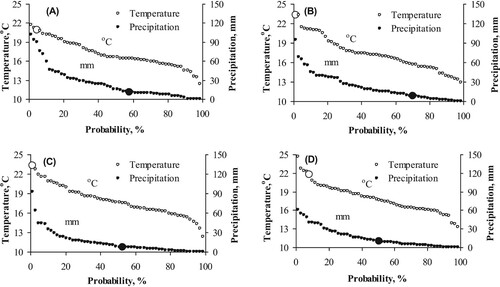
In 2011, the trend of warmer air temperature continued for the time period before infection ((A)) and later on for the period 15–22 July (within the 48-year weather record, only about 25% of years were warmer during this time period; (B)). For the period 23–29 July, air temperature was warmer in only 18% of years with available weather data ((C)). In 2011, the whole observation period except the 10-day period before the first disease symptoms were recorded (5−15 July), was dry (drier weather during the pertinent periods observed only for 24.3–33.2% of the years during 48 years period; (B–D)).
Figure 2. Cumulative probability of occurrence of certain average temperature (°C) and precipitation (mm) conditions at the Eerika experimental field according to long-term (1964–2011) weather data. The cumulative probability was calculated for the pertinent study periods and corresponding average temperature and precipitation values for the growing season 2011 are also shown. The relevant time periods as: A – for 10 days before first sampling (05.07–14.07), B – for (15.07–21.07), C – for (22.07–28.07) and D – for (29.07–04.08).
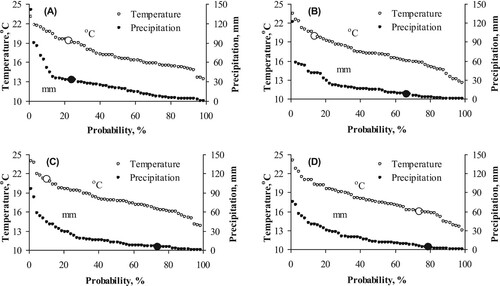
In 2010, the first occurrence of powdery mildew infection was found on 12 July on S. nigra plants. A week later, on 20 July, the infection was recorded on B. juncea plants with 1.6% and on B. napus plants with 3.0%, whereas the powdery mildew infection had increased already to 48% in S. nigra plants. Until 26 July, powdery mildew development progressed on B. nigra, B. napus and B. juncea plants significantly, being most severe on plants of B. nigra and B. napus plots (F5,12 = 71.69, P < 0.001) ((A)). By the end of the observation period, 83% of foliage was infected by powdery mildew on B. nigra plants (BBCH 81–83) and 89% of foliage on B. napus plants (BBCH 79–81; significantly higher degree of infection compared to other species, F5,12 = 788.63, P < 0.001). The infection on B. juncea plots culminated with 55% (BBCH 86–88) ((A)). Erysiphe cruciferarum infection was not found on S. alba, E. sativa or R. sativus plants.
Figure 3. Development of powdery mildew (E. cruciferarum) on six different cruciferous oilseed crops in 2010 (A) and 2011 (B). Error bars denote standard error of the means. The asterisks demonstrate the significance of the species effect. *Significant at P < 0.05; **Significant at P < 0.01; ***Significant at P < 0.001. ns Not-significant.
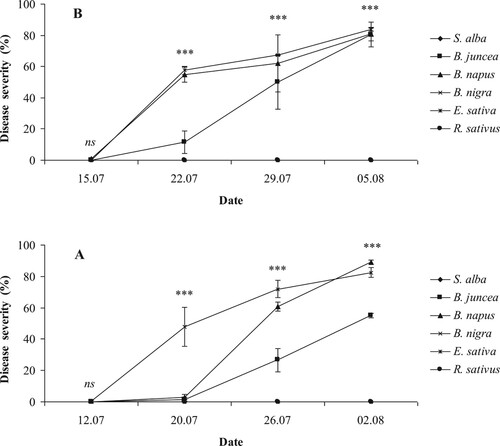
In 2011, the first low-level infection of powdery mildew was recorded on 15 July on B. napus plants ((B)). A week later, on 22 July, the disease infection on B. napus plants had increased to 54.7% and on B. juncea plants to 11.7% and extremely fast powdery mildew development was observed on B. nigra plants with 57.5% infection ((B)). Powdery mildew development further progressed at a moderate rate and culminated on B. juncea (BBCH 86–89) and on B. napus plants at 80% infection (BBCH 78–81) and on B. nigra plants at 83.7% infection (BBCH 82–85) (F5,12 = 144.39, P < 0.001).
In 2011, significant differences in AUDPC values were also found between the six studied crops (F5,12 = 32.88, P < 0.001). As in 2010, the AUDPC value was zero in three crops: E. sativa, R. sativus and S. alba (). The highest AUDPC values were 1166.7 ± 12.1 in B. nigra and 1104.7 ± 163.4 in B. napus (), showing high susceptibility to powdery mildew. Brassica juncea plants with the AUDPC value of 714.0 ± 178.2 can also be classified as susceptible to powdery mildew. Combined two-year results further emphasised the significant species effect on AUDPC value of powdery mildew infection (F5,24 = 3.48, P = 0.02).
Figure 4. Values of the mean area under the disease progress curve (AUDPC) of powdery mildew (E. cruciferarum) infection on different cruciferous oilseed crops. Error bars denote the standard error of the means. Means followed by different capital letters above each bar indicate a significant influence (P < 0.05) of cruciferous oilseed crops in different years (Tukey HSD test). Means followed by different small letters above each bar indicate a significant influence (P < 0.05) of year (Tukey HSD test).
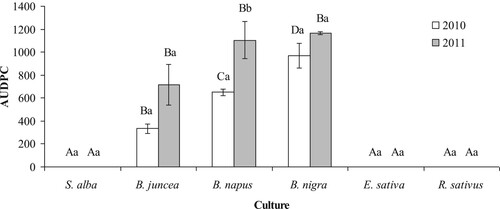
Based on AUDPC values, the powdery mildew pressure differed significantly between the two observation years (F1,24 = 14.53, P < 0.001) and test species (F5,24 = 76.69, P < 0.001). Although the overall disease pressure in 2010 was lower compared to 2011, significant differences in AUDPC values were found between the six crops (F5,12 = 69.05, P < 0.001) (). The highest average ± SE value of AUDPC of 969.9 ± 109.1 was observed in B. nigra plants followed by B. napus with a value of 650.5 ± 29.8, and B. juncea with a value of 333.8 ± 42.1 (). The three other crops, S. alba, E sativa and R. sativus, had no infection ().
Discussion
Powdery mildew infection on cruciferous crops: sporadic or epidemic disease
In this study, development of powdery mildew was assessed on oilseed rape and on five alternative cruciferous oilseed crops species. The results clearly showed that under warm and rather dry weather conditions E. cruciferarum is an important pathogen in northern Baltic conditions, particularly on some oilseed crops. An especially severe powdery mildew infection of E. cruciferarum was recorded in both growing seasons on black mustard plants. In both years, the degree of infection on black mustard and oilseed rape plants was more than 80% at the end of the observation period (). Also, on mustard green (B. juncea) plants, the disease severity at the end of the growing season reached up to 80% in 2011, however, the development rate was moderate compared to black mustard and oilseed rape plants ((B)).
So far, the pathogen E. cruciferarum is considered an important disease of oilseed rape, causing crop losses in warmer areas of Europe such as France (Penaud Citation1999), Turkey (Mert-Türk et al. Citation2008) and on alternative hosts camelina (Camelina sativa) and wild mustard (Sinapis arvensis) under Mediterranean conditions in Greece (Vellios et al. Citation2017). The powdery mildew can be a rather destructive disease on B. juncea in India (Desai et al. Citation2004) due to infestation of pods, carrying infection on seeds (Dange et al. Citation2002). Our results support the high disease severity of B. juncea in comprehensive research findings from Indian fields (Mehta et al. Citation2008; Meena et al. Citation2018). In contrast, a three-year fungal disease survey on oilseed rape in Australia classified powdery mildew as a sporadic disease (Van de Wouw et al. Citation2016). Nevertheless, severe powdery mildew infection has also been already reported on rapeseed crops in Poland (Sadowski et al. Citation2002). Based on our two-year observations, the powdery mildew on spring oilseed rape, black mustard and B. juncea cannot any longer be classified as a sporadic disease in the northern Baltic region.
How weather controls powdery mildew infection and how climate change could alter the disease severity.
The main climate factors temperature and precipitation can influence the development of powdery mildews (Erysiphe spp.) (Penaud Citation1999; Jorgensen Citation2012). Thus, the disease severity due to powdery mildews vary across habitats and growing seasons (Enright and Cipollini Citation2007; Copolovici et al. Citation2014). Powdery mildew is typically more severe in warm dry summers during periods of higher humidity (EPPO Bull Citation1996). Hot weather conditions have been shown to be positively correlated with the severity of powdery mildew infection on B. juncea (Desai et al. Citation2004), Camelina sativa and Sinapis arvensis plants (Vellios et al. Citation2017). In our study, the weather conditions in both years before and during the disease development were significantly warmer and dryer compared with 48 years average and can be considered as extreme (, 2; ). These extreme conditions were favourable for powdery mildew development, because the quantitative disease resistance assessment on powdery mildew with AUDPC showed high values on both growing seasons. Nevertheless, the climatic conditions in the two growing seasons were somewhat different. In 2010, July was 4.8°C warmer compared to long-term average, with extremely warm periods in the second decade of July that was 6.0°C (± 2.2°C) and the third decade that was 5.5°C (±2.0°C) warmer than the long-term average. The overall disease pressure was slightly lower under warmer weather conditions in 2010. However, it was enough to damage most of foliage by the end of the growing period in B. nigra, B. napus and B. juncea, and a significantly lower AUDPC value was only observed in B. napus in 2010 compared with the year 2011.
Powdery mildew on rapeseed-mustard has become an epidemic disease in India due to the rise of temperature during the past two decades (Meena et al. Citation2018). Similarly, powdery mildew has recently become more severe on commercial oilseed rape and mustard crops in northern NSW and Northern agricultural region of Western Australia (Uloth et al. Citation2016) due to warmer growing season temperatures (Barbetti et al. Citation2012). However, drought conditions in continental type of climate slowed down the development of powdery mildew on plants of garlic mustard (Alliaria petiolata), suggesting that mildew infection can be particularly detrimental in moist weather conditions (Enright and Cipollini Citation2007). Nevertheless, even under drought, the whole leaf area of A. petiolata was infested by the fungus at the end of the experiment, indicating that powdery mildew can affect plant fitness under a wide range of moisture conditions (Enright and Cipollini Citation2007). Differently from Mediterranean climates (Barbetti et al. Citation2012), hot daytime temperatures in Northern Europe are typically coupled with high humidity, implying that there is often night-time dew formation on leaves when temperatures fall. This could provide enough moisture for infection, even in periods with hot daytime conditions.
We argue that the spread of the powdery mildew disease in Northern Europe is expected to be further enhanced in future warmer climatic conditions. The frequency of hot summers has increased in Northern Europe (Kocmánková et al. Citation2010) and such conditions are predicted to increase in the future (Pulatov et al. Citation2015). The recent monitoring study shows that the climate in the Baltic Sea region, including Estonia, has warmed particularly fast comparing with the global average (Lakson et al. Citation2019). This could increase problems due to E. cruciferarum pathogen damage and powdery mildew control in northern regions. In warmer climatic conditions, new pathogens will give rise to new challenges in the future (Jönsson et al. Citation2013), e.g. similarly to E. cruciferarum, higher temperatures have enhanced the spread of potato early blight (Alternaria spp.) in northern parts of Europe (Runno-Paurson et al. Citation2015; Odilbekov et al. Citation2016). Our results confirm that powdery mildew has already become a more serious problem for northern Baltic oilseed crops. As the disease pressures on oilseed crops are already high due to soil-borne pathogens (Veromann et al. Citation2013), powdery mildew includes an additional threat to plant yield.
What factors could be responsible for almost full powdery mildew resistance of some cruciferous crops?
Some cruciferous species like S. alba, E. sativa and R. sativus exhibited full foliar resistance to powdery mildew in both study years (). Gunasinghe et al. (Citation2013) have reported powdery mildew occurrence on E. sativa leaves after inoculation at the late flowering stage in 2012 in Australia. Widespread use of one or more highly susceptible cultivars of oilseed rape and mustard crops has enhanced the risk of powdery mildew infection and has increased the severity of disease in northern Australia (Uloth et al. Citation2016). Thus, the cultivar resistance offers the first opportunity for effective management of the emerging powdery mildew on oilseed crops in Australia (Uloth et al. Citation2018). As host resistance plays an important role in disease dispersal, genetic engineering is a promising tool to reduce infection. Promising results of enhanced E. cruciferarum resistance of B. juncea transgenic lines with BjNPR1 have been observed (Ali et al. Citation2017).
The content of glucosinolates and/or emission of their volatile metabolites or other nitrogen and sulphur containing phytochemicals such as phytoalexins and glutathione, which are de novo synthesised upon pathogen attack may be one of the reasons for the varying stress resistance of cruciferous plants (Ahuja and Bones Citation2012; Czerniawski and Bednarek Citation2018; Gullner et al. Citation2018). In addition, stress tolerance is affected by daily temperature and light intensity not to mention the soil pH as the co-effect of UV-B radiation intensity and temperature was probably the reason, why B. juncea, B. napus and E. sativa grown in the field contained four times more glucosinolates than the ones grown in greenhouse (Mewis et al. Citation2012). Finally, higher resistance of S. alba, R. sativus and E. sativa to powdery mildew compared to B. juncea, B. napus and B. nigra may also be related to the plantś morphological traits such as the density of non-glandular trichomes on plants (Khalik Citation2005). For example, sparse distribution of trichomes is characteristic to B. napus leaves, while the ones of B. villosa are much more densely and uniformly covered with trichomes (Nayidu et al. Citation2014). In general, the abundance of non-glandular trichomes increases leaf surface reflectance and thus, enhances plant resistance to drought stress (Hallik et al. Citation2017). However, they also capture airborne particles including fungal spores and thus, higher density of trihomes might increase the risk of fungal infections (Roda et al. Citation2003). Non-glandular trichomes are excellent habitats for pathogens unless they contain an anti-fungal hydrolase, which inhibits or decreases the fungal infection by hydrolysing the cell walls of fungi (Kim Citation2019). In summary, based on the knowledge of glucosinolates and trichomes, efforts have been made to improve the stress tolerance of, for example, B. napus through genetic engineering by transferring a tissue-specific lipid transfer protein of B. rapa to B napus in order to increase the abundance of trichomes and glucosinolates in plant leaves (Tian et al. Citation2018).
Currently, there is a limited assortment of alternative cruciferous and non-cruciferous oilseed crops for cultivation in northern regions, and there is a strong demand for new oilseed crops by local producers (Runno-Paurson et al. Citation2019a). Based on our observations, alternative oilseed crops such as S. alba, E. sativa and R. sativus, even under favourable conditions for powdery mildew infection, possess a great potential to grow without any chemical input in northern Baltic conditions. As powdery mildew poses a considerable threat to oilseed crops, resistant species and resistant cultivars available must be harnessed, especially in organic farming systems. This knowledge is also important for cover crop and green manure production to suppress pathogens (Larkin and Halloran Citation2014; Runno-Paurson et al. Citation2015, Citation2019b) and therefore to select species and cultivars that are less prone to disease dispersal. White mustard (S. alba) could be particularly interesting because it is very suitable as a cover crop due to fast initial growth, has a high level of glucosinolates and is a low-demanding crop (Lee et al. Citation2014). Locally, white mustard has growing importance in organic farming use as an alternative lodging culture due to increasing lodging resistance of field peas (Rauber et al. Citation2001).
Acknowledgements
The study was supported by the European Regional Development Fund (Center of Excellence EcolChange: Ecology of global change: natural and managed ecosystems) and the Estonian University of Life Sciences project (base funding P190259PKTT).
Disclosure statement
No potential conflict of interest was reported by the author(s).
Additional information
Funding
Notes on contributors
Eve Runno-Paurson
Dr. Eve Runno-Paurson working as Assoc. Prof. at the Chair of Crop Science and Plant Biology, Estonian University of Life Sciences (EULS). She is phytopathologist, potato late blight key person of Baltics. Her area of expertise include changes in phenotypic and genetic structure and diversity of plant pathogens, development and optimization of sustainable plant protection strategies, molecular diagnostics of plant pathogens and stressors of new crops.
Peeter Lääniste
Peeter Lääniste is a PhD student of EULS. He is a specialist of novel oilseed crops cultivation technologies in Estonia.
Viacheslav Eremeev
Dr. Viacheslav Eremeev, is a research fellow at the Chair of Crop Science and Plant Biology, EULS. His area of expertise include field crop husbandry, potato, crop rotation, regulation humus status of soil in conventional and organic farming.
Liina Edesi
Dr. Liina Edesi is working at Estonian Crop Research Institute as senior researcher. She has good skills in soil microbial activity and community composition investigations in field experiments under the different crop rotations.
Luule Metspalu
Dr. Luule Metspalu is working at EULS as senior researcher. She is well-experienced specialist in plant protection, entomology and trap crops.
Astrid Kännaste
Dr. Astrid Kännaste is working at EULS as senior researcher. Her research interests are related to studies on biotic and abiotic plant stressors, plant chemotypes and also adaptation and survival of plants in environment under changing climate.
Ülo Niinemets
Prof. Ülo Niinemets leading the Chair of Crop Science and Plant Biology at EULS. His main research interest is what determines vegetation distribution on the globe. He focus on plant acclimation and adaptation to abiotic and biotic stresses with particular emphasis on stress-dependent volatile release.
References
- Ahuja IK, Bones AMR. 2012. Phytoalexins in defense against pathogens. Trends Plant Sci. 17:73–90.
- Ali S, Mir ZA, Tyagi A, Mehari H, Meena RP, Bhat JA, Yadav P, Papolu PL, Rawat S, Grover A. 2017. Overexpression of NPR1 in Brassica juncea confers broad spectrum resistance to fungal pathogens. Front Plant Sci. 8:1693.
- Antonious GF, Bomford M, Vincelli P. 2009. Screening Brassica species for glucosinolate content. J Environ Sci. and Health. 44:311–316.
- Bankina B, Gaile Z, Balodis O, Paura L, Kokina I. 2012. Possibilities for integrated control of winter oilseed rape diseases in Latvia. Bulletin OEPP/EPPO Bull. 42:560–567.
- Barbetti MJ, Banga SS, Salisbury PA. 2012. Challenges for crop production and management from pathogen biodiversity and diseases under current and future climate scenarios – case study with oilseed Brassicas. Field Crops Res. 127:225–240.
- Cho SE, Park JH, Choi YJ, Choi IY, Shin HD. 2016. First report of powdery mildew caused by Erysiphe cruciferarum on oilseed rape in Korea. Plant Dis. 100:1245.
- Copolovici L, Väärtnõu F, Portillo Estrada M, Niinemets Ü. 2014. Oak powdery mildew (Erysiphe alphitoides)-induced volatile emissions scale with the degree of infection in Quercus robur. Tree Physiol. 34:1399–1410.
- Czerniawski P, Bednarek P. 2018. Glutathione S-transferases in the biosynthesis of sulfur-containing secondary metabolites in Brassicaceae plants. Front Plant Sci. 9:1639.
- Dange SRS, Patel RL, Patel SI, Patel KK. 2002. Assessment of losses in yield due to powdery mildew disease in mustard under North Gujarat conditions. J Mycol Plant Pathol. 32:249–250.
- Desai AG, Chattopadhyay C, Agrawal R, Kumar A, Meena RL, Meena PD, Sharma KC, Srinivasa Rao M, Prasad YG, Ramakrishna YS. 2004. Brassica juncea powdery mildew epidemiology and weather-based forecasting models for India – a case study. J Plant Dis Protect. 111:429–438.
- Elad Y, Pertot I. 2014. Climate change impacts on plant pathogens and plant diseases. J Crop Improv. 28:99–139.
- Enright SM, Cipollini D. 2007. Infection by powdery mildew Erysiphe cruciferarum (Erysiphaceae) strongly affects growth and fitness of Alliaria petiolate (Brassicaceae). Am J Bot. 94:1813–1820.
- EPPO Bull. 1996. Guideline on good plant protection practice. Rape. EPPO Bull. 26:349–367.
- EPPO Bull. 2003. Efficacy evaluation of fungicides: root, stem, foliar and pod diseases of rape. EPPO Bull. 33:25–32.
- Gullner G, Komives T, Király L, Schröder P. 2018. Glutathione S-transferase enzymes in plant-pathogen interactions. Front Plant Sci. 9:1836.
- Gunasinghe N, You MP, Lanoiselet V, Eyres N, Barbetti MJ. 2013. First report of powdery mildew caused by Erysiphe cruciferarum on Brassica campestris var. pekinensis, B. carinata, Eruca sativa, E. vesicaria in Australia and on B. rapa and B. oleracea var. capitata in Western Australia. Plant Dis. 97:1256–1256.
- Hallik L, Kazantsev T, Kuusk A, Galmés J, Tomás M, Niinemets Ü. 2017. Generality of relationships between leaf pigment contents and spectral vegetation indices in Mallorca (Spain). Reg Environ Change. 17:2097–2109.
- Hossain MS, Ye W, Hossain MA, Okuma E, Uraji M, Nakamura Y, Mori IC, Muarata Y. 2013. Glucosinolate degradation products, isothiocyanates, nitriles, and thiocyanates, induce stomatal closure accompanied by peroxidase-mediated reactive oxygen species production in Arabidopsis thaliana. Biosci Biotechnol, Biochem. 77:977–983.
- Jönsson AM, Pulatov B, Linderson ML. 2013. Modelling as a tool for analysing the temperature-dependent future of the Colorado potato beetle in Europe. Glob Chang Biol. 19:1043–1055.
- Jorgensen TH. 2012. The effect of environmental heterogeneity on RPW8-mediated resistance to powdery mildews in Arabidopsis thaliana. Ann Bot. 109:833–842.
- Kalischuk AR, Dosdall LM. 2004. Susceptibilities of seven Brassicaceae species to infestation by the cabbage seedpod weevil (Coleoptera: Curculionidae). Can Entomol. 136:265–276.
- Karakaya A, Gray FA, Koch D. 1993. Powdery mildew of Brassica spp. in Wyoming. Plant Dis. 77:1063.
- Khalik KA. 2005. Morphological studies on trichomes of Brassicaceae in Egypt and taxonomic significance. Acta Bot Croat. 64:57–73.
- Kim JY, Kim BS, Cho SE, Shin HD. 2013. First report of powdery mildew caused by Erysiphe cruciferarum on Indian mustard (Brassica juncea) in Korea. Plant Dis. 97(10):1383–1383.
- Kim KW. 2019. Plant trichomes as microbial habitats and infection sites. Eur J Plant Pathol. 154:157–169.
- Kissen R, Øverby A, Winge P, Bones AM. 2016. Allyl-isothiocyanate treatment induces a complex transcriptional reprogramming including heat stress, oxidative stress and plant defence responses in Arabidopsis thaliana. BMC Genomics. 17:740.
- Koike ST, Gladders P, Paulus AO. 2006. Vegetable diseases: a colour handbook. London: CRC Press. 448 p.
- Kocmánková E, Trnka M, Eitzinger J, Formayer H, Dubrovský M, Semerádová D, Žalud Z, Juroch J, Možný M. 2010. Estimating the impact of climate change on the occurrence of selected pests in the Central European region. Clim Res. 44:95–105.
- Lakson M, Post P, Sepp M. 2019. The impact of atmospheric circulation on air temperature rise in Estonia. Front Earth Sci. 7:131.
- Lancashire PD, Bleiholder H, Boom TVD, Langelüddeke P, Strauss R, Weber E, Witzenberger A. 1991. A uniform decimal code for growth stages of crops and weeds. Ann App Biol. 119:561–601.
- Larkin RP, Halloran JM. 2014. Management effects of disease-suppressive rotation crops on potato yield and soilborne disease and their economic implications in potato production. Am J Potato Res. 91:429–439.
- Lee RW, Malchev IT, Rajcan I, Kott LS. 2014. Identification of putative quantitative trait loci associated with a flavonoid related to resistance to cabbage seedpod weevil (Ceutorhynchus obstrictus) in canola derived from an intergeneric cross, Sinapis alba x Brassica napus. Theor Appl Genet. 127:419–428.
- Meena PD, Mehta N, Rai PK, Saharan GS. 2018. Geographical distribution of rapeseed-mustard powdery mildew disease in India. J Mycol Pl Pathol. 48:284–302.
- Mehta N, Singh K, Sangwang MS. 2008. Assessment of yield losses and evaluation of different varieties/ genotypes of mustard against powdery mildew in Haryana. Pl Dis Res. 23:55–59.
- Mert-Türk F, Gül MK, Egesel CO. 2008. Nitrogen and fungicide applications against Erysiphe cruciferarum affect quality components of oilseed rape. Mycopathologia. 165:27–35.
- Mewis I, Schreiner M, Nguyen CN, Krumbein A, Ulrichs C, Lohse M, Zrenner R. 2012. UV-B irradiation changes specifically the secondary metabolite profile in Broccoli sprouts: induced signalling overlaps. Plant Cell Physiol. 53:1546–1560.
- Nayidu NK, Tan Y, Taheri A, Li X, Bjorndahl TC, Nowak J, Wishart DS, Hegedus D, Gruber MY. 2014. Brassica villosa, a system for studying non-glandular trichomes and genes in the Brassicas. Plant Mol Biol. 85:519–539.
- Odilbekov F, Edin E, Garkava-Gustavsson L, Persson Hovmalm H, Liljeroth E. 2016. Genetic diversity and occurrence of the F129L substitutions among isolates of Alternaria solani in south-eastern Sweden. Hereditas. 153:10.
- Pandey P, Irulappan V, Bagavathiannan MV, Senthil-Kumar M. 2017. Impact of combined abiotic and biotic stresses on plant growth and avenues for crop improvement by exploiting physio-morphological traits. Front Plant Sci. 8:537.
- Penaud A. 1999. Chemical control and yield losses caused by Erysiphe cruciferarum on oilseed rape in France. In: N. Wratten, P. A. Salibury, editors. Proceedings of the 10th international rapeseed congress. Canberra: The Regional Institute; CD-ROM Doc No. 327:1–8.
- Pulatov B, Linderson ML, Hall K, Jönsson AM. 2015. Modeling climate change impact on potato crop phenology, and risk of frost damage and heat stress in Northern Europe. Agric For Meteorol. 214–215:218–292.
- Rauber R, Schmitke K, Kimpel-Freund H. 2001. The performance of Pea (Pisum sativum L.) and its role in determining yield advantages in mixed stands of pea and oat (Avena sativa L.). J Agron Crop Sci. 187:137–144.
- Roda A, Nyrop J, English-Loeb G. 2003. Leaf pubescence mediates the abundance of non-prey food and the density of the predatory mite Typhlodromus pyri. Exp Appl Acarol. 29:193–211.
- Roostalu H. 2008. Riskid taimekasvatuses (risks in crop production). In: Roostalu H, editor. Agromajanduslikud riskid taimekasvatuses ja nende leevendamise võimalused (Agroeconomical risks in crop production and their mitigation possibilities). p. 39–105. Tartu: Tartumaa Põllumeeste Liit. [in Estonian].
- Runno-Paurson E, Loit K, Hansen M, Tein B, Williams IH, Mänd M. 2015. Early blight destroys potato foliage in the northern Baltic region. Acta Agric Scand B Soil Plant Sci. 65:422–432.
- Runno-Paurson E, Eremeev V, Tõrra T, Niinemets Ü, Lääniste P. 2019a. Õlikanepi kasvatustehnoloogiate optimeerimine. (Optimization of cultivation technologies for industriaal hemp (Cannabis sativa cv. Finola). In: I. Tupits, S. Tamm, Ü, Tamm, A. Toe, editors. Agronoomia 2019. p. 96–103. Jõgeva: Eesti Taimekasvatuse Instituut ja Eesti Maaülikool. [in Estonian].
- Runno-Paurson E, Lääniste P, Eremeev V, Tähtjärv T, Kaurilind E, Tosens T, Niinemets Ü, Williams IH. 2019b. Does winter oilseed rape as a winter cover crop influence potato late blight development in an organic crop rotation? Biological Agriculture and Horticulture. 35:1–13.
- Sarasketa A, González-Moro MB, González-Murua C, Marino D. 2016. Nitrogen source and external medium pH interaction differentially affects root and shoot metabolism in Arabidopsis. Front Plant Sci. 29:1–12.
- Sadowski C, Dakowska S, Lukanowski A, Jedryczka M. 2002. Occurrence of fungal diseases on spring rape in Poland. In: VH Paul, I Föller, editors. ‘Working group: integrated control in oilseed crops’. April 2001, Soest. Vol. 25; p. 1–12. (IOBC/WPRS Bulletin: Dijon, Cedex).
- Shaner G, Finney RE. 1977. The effect of nitrogen fertilization on the expression of slow-mildewing resistance in Knox wheat. Phytopathology. 67:1051–1056.
- Stavrinides MC, Daane KM, Lampinen BD, Mills NJ. 2010. Plant water stress, leaf temperature, and spider mite (Acari: Tetranychidae) outbreaks in California vineyards. Environ Entomol. 39:1232–1241.
- Tian NN, Liu F, Wang PD, Yan XH, Gao HF, Zeng XH, Wu G. 2018. Overexpression of BraLTP2, a lipid transfer protein of Brassica napus, results in increased trichome density and altered concentration of secondary metabolites. Int J Mol Sci. 19:1–22.
- Trejo-Téllez LI, Estrada-Ortiz E, Gómez-Merino FC, Becker C, Krumbein A, Schwarz D. 2019. Flavonoid, nitrate and glucosinolate concentrations in Brassica species are differently affected by photosynthetically active radiation, phosphate and phosphite. Front Plant Sci. 10:371.
- Uloth MB, You MP, Barbetti MJ. 2016. Cultivar resistance offers the first opportunity for effective management of the emerging powdery mildew (Erysiphe cruciferarum) threat to oilseed brassicas in Australia. Crop Pasture Sci. 67:1179–1187.
- Uloth MB, You MP, Barbetti MJ. 2018. Plant age and ambient temperature: significant drivers for powdery mildew (Erysiphe cruciferarum) epidemics on oilseed rape (Brassica napus). Plant Path. 67:445–456.
- Van de Wouw AP, Idnurm A, Davidson JA, Sprague SJ, Khangura RK, Ware AH, Lindbeck KD, Marcroft SJ. 2016. Fungal diseases of canola in Australia: identification of trends, threats and potential therapies. Australas Plant Pathol. 45:415–423.
- Velásquez AC, Castroverde CDM, He SY. 2018. Plant and pathogen warfare under changing climate conditions. Curr Biol. 28:R619–R634.
- Vellios E, Karkanis A, Bilalis D. 2017. Powdery mildew (Erysiphe cruciferarum) infection on camelina (Camelina sativa) under Mediterranean conditions and the role of wild mustard (Sinapis arvensis) as alternative host of this pathogen. Emir J Food Agric. 29:639–642.
- Veromann E, Toome M, Kännaste A, Kaasik R, Copolovici L, Flink J, Kovács G, Narits L, Luik A, Niinemets Ü. 2013. Effects of nitrogen fertilization on insect pests, their parasitoids, plant diseases and volatile organic compounds in Brassica napus. Crop Prot. 43:79–88.