ABSTRACT
Silicate rock powder (SRP) restores the fertility of weathered soils. However, its slow nutrient release is a disadvantage for short-duration crops. Humic-like acids (HLAs) are plant biostimulants that enhance root development and nutrient uptake. This work evaluates the effects of the co-application of HLA extracted from a vermicompost and SRP on the nutrient uptake and growth of maize cultivated in weathered soil in Brazil. The chemical composition of HLA was assessed using Fourier-transform infrared spectroscopy and 13Carbon-nuclear magnetic resonance, revealing an overall characteristic of hydrophobicity. A preliminary trial with different HLA concentrations (0, 20, 40, 80, and 160 mg L−1) revealed that 40 mg L−1 HLA resulted in the highest increase in the root area, dry root weight, H+ efflux, and the number of lateral roots, compared with other concentrations. The main experiment using soil treated with SRP at different rates (0, 600, 1200, 1800, and 2400 kg ha−1) showed that the co-application of SRP and HLA caused a significant difference in the root and total plant weights, compared with the sole SRP application. Furthermore, it increased the nutrient content of the plants. These effects are mainly because of increased proton pump activity and the hydrophobicity of HLA.
Introduction
The excessive use of chemical fertilizers in agriculture has recently raised concerns regarding its environmental impacts. Over-fertilized soils are the primary anthropogenic source of nitrous oxide (N2O) – one of the greenhouse gases that causes global warming (Houghton et al. Citation2016). In addition, fertilizers are the major constituents of agricultural drainage waters, resulting in the eutrophication of receiving surface waters (Norse Citation2005). The agricultural economy in developing countries still depends on the international market to meet its domestic demand for fertilizers, making them expensive to farmers with economic constraints. In addition, some farming systems (e.g. organic agriculture) do not allow the use of soluble fertilizers and have raised the demand for other sources of plant nutrients. The use of silicate rock powder (SRP) is a sustainable approach to increase the fertility level of weathered soils (Harley and Gilkes Citation2000; Manning et al. Citation2017). Compared with soluble fertilizers, SRP causes slow nutrient release (Tamfuh et al. Citation2019), allowing a gradual supply that reduces nutrient leaching. While the industry focuses almost exclusively on producing fertilizers containing N, P, and K, SRP presents a combination of different nutrients such as SiO2, Al2O3, K2O, and Fe2O3 (Swoboda et al. Citation2022). Moreover, compared with chemical synthetic fertilizers which contribute to green house gas emission during its manufacturing process, using SRP from local sources is not only more sustainable due to slow-release fertilizer which minimizes nutrient leaching and, but also more economical to farmers as the proximity of the source helps eliminate transportation costs (Van Straaten Citation2006). For these reasons, many scientific works on SRP have been conducted worldwide, both in greenhouses and open fields (Van Straaten Citation2006; Silva et al. Citation2012; Tamfuh et al. Citation2019; Krahl et al. Citation2020).
Despite the high capacity of SRP to improve the fertility of weathered soils, part of the nutrients present in the SRP can remain trapped in the solid matrix of rock particles, taking years to naturally solubilize in the soil (Marchi et al. Citation2020). The slow release of the nutrients is advantageous in long-term agricultural systems or perennial crop production (Lubkowski Citation2016) but criticized as a disadvantage for short-cycle crop production (Mohammed et al. Citation2014). Some chemical modification processes, such as microcrystallization, can increase nutrient solubilization (Zhang et al. Citation2019). However, this modification involves high costs and fossil energy (Ciceri et al. Citation2017). Thus, an alternative strategy with a more eco-friendly and cost-effective approach needs to be explored.
Plant biostimulants are substances or microorganisms that, regardless of their nutrient content, enhance the nutrition efficiency, abiotic stress tolerance, and crop quality traits of plants (Du Jardim Citation2015). Humic-like acids (HLAs), defined as the extracts derived from different organic materials that have not undergone the natural process of humification (Bento et al. Citation2019), are a well-known category of plant biostimulants that have improved the growth and yield of many crops (Balmori et al. Citation2019). HLAs are formed mainly by associations of hydrophobic structures stabilized by weak dispersive forces (Piccolo Citation2002). HLAs are considered plant biostimulants because of their capacity to promote plant growth by releasing bioactive molecules with hormonal-like activities (Canellas et al. Citation2002; Nardi et al. Citation2018; Zandonadi et al. Citation2019). HLAs stimulate enzymatic activities and the expression of genes involved in nutrient acquisition by triggering modifications in the content of chemicals involved in different physiological processes, such as the Krebs cycle and photosynthesis (Vujinovic et al. Citation2020). It has also been reported that HLAs can alter the root exudation profile of organic acids in plants; they increase the efflux of oxalic and citric acids in maize (Zea mays L.) plants (Canellas et al. Citation2008). These organic acids are strongly linked to the carbon cycle with microorganisms as part of this cycle involves the interaction between organic acids and microbes and the colonization of mineral surfaces (Adeleke et al. Citation2017). This is the first part of the weathering process, which results in mineral solubilization and the consequent release of cations for plants growth. These events also influence the ability of plants to absorb nutrients from SRP, increasing their agronomic efficiency (Adeleke et al. Citation2017). However, this strategy of co-application of SRP and HLA has not yet been deeply assessed.
Humic substance (HS) in soils and sediments, comprised of humic acid (HA), fulvic acid (FA), and humin, is the main component of soil organic matter, which is involved in many processes in soils.
In contrast, composting is a biodegradation process that creates HLA and fulvic-like acid (FLA) after decomposing raw organic materials. Compost can be a beneficial resource in regions that have weathered soils with low soil organic carbon. Unlike humic acids (HAs) originated from soils, leonardite, and peat, which have more stable forms, with a high carbon content enriched with aromatic groups (Stevenson Citation1994), HLA derived from compost has less structuralized chemical composition. The peculiar trait of HLA in terms of agronomic value is that several easily degradable compounds remain in the moiety of HLA throughout the composting process and these compounds may benefit macro- and micronutrient uptake by plants (Plaza et al. Citation2005).
The combined application of rock phosphate (RP) with other materials, such as microorganisms, biostimulants, and compost, has been studied recently (Ditta et al. Citation2018; Aliyu et al. Citation2019; Maharana et al. Citation2021). However, little is known about the optimal rate of these valuable materials, especially under low fertile soil conditions.
The purpose of this work was to verify if the co-application of HLA and SRP could enhance plant growth in the weathered soil of the tropical savanna agro-climate zone of Brazil. Firstly, treatments with different concentrations of HLA (0, 40, 60, 80 160 mg L−1) were conducted as a preliminary trial to find an optimal concentration to promote maize root growth and stimulate the proton pump activity. Then, a pot experiment using weathered soil was carried out with different SRP rates (0, 600, 1200, 1800, and 2400 kg ha−1) to evaluate the effects of varying SRP rates and the co-application of SRP and HLA on plant morphology and nutrient uptake. Furthermore, spectral analysis was conducted to identify the properties and chemical composition of the HLA used in this study.
Materials and methods
Extraction and characterization of humic-like acid derived from vermicompost of bovine manure
The vermicompost was produced in polyethylene boxes with a capacity of 310 dm3 using fresh bovine manure and earthworms (Eisenia foetida) at a rate of 80 worms per kg of bovine manure. The bovine manure and earthworms are taken from a livestock farm which belongs to the University of Brasília, Brazil. The composting was carried out over 120 days, and the moisture and temperature were checked to ensure proper biodegradation. The ranges of moisture during the composting process were between 40 and 60 percent. The temperature raised up to 60°C at thermophilic phase and reduced till 30° during the cooling phase. The HLA was obtained from the vermicompost of the bovine manure, according to the standard methods of extraction, isolation, and purification of the International Humic Substances Society (IHSS Citation2019 ). As a brief description of the procedure, a solution of 0.5 M NaOH was mixed with earthworm compost (10:1, v/v) under an N2 atmosphere, and after 12 h, the suspension was centrifuged (5000 × g). The HLA was precipitated by adding 6 M HCl until the pH was 1.5. After centrifugation (5000 × g) for 15 min, the sample was repeatedly washed with distilled water until a negative test against AgNO3 was obtained. Subsequently, the sample was dialyzed against deionized water using a 1000-Da cut-off membrane (Thomas Scientific, Swedesboro, NJ, USA) and lyophilized. After lyophilization, the elemental composition of the HLA was determined using a CHN analyzer (Perkin-Elmer 2400, Perkin-Elmer, Waltham, Massachusetts, USA). Different types of acidity in the HLA sample was measured according to Schnitzer & Gupta (Citation1965) (Supplemental information SI 1). The chemical composition of the HLA was studied using Fourier transform infrared (FTIR) spectroscopy and cross-polarization magic angle spinning 13Carbon nuclear magnetic resonance spectroscopy (CPMAS 13C-NMR). The FTIR spectra were recorded on KBr pellets in the 400–4000 cm–1 spectral range using a Varian FTIR 660 spectrometer (Varian Inc., Palo Alto, California, USA).with a resolution of 4 cm−1; 100 scans were conducted. The CPMAS 13C-NMR was performed at 150.91 MHz using a Bruker Avance III spectrometer (Bruker Corporation, Billerica, MA, USA) after packing the sample in zirconia rotors with Kel-F caps and spinning it at 10 kHz. The experiments were performed under a contact time of 2 ms, an acquisition time of 22.5 ms, and a recycle delay of 1 s. The identification of bands in the present work was carried out according to the method described by Stevenson (Citation1994). The ACD/NMR processor software was used to process and analyze the spectra. The areas of different 13C resonance signals were divided into main integrating regions and assigned to the respective functional groups: 0–45 (aliphatic C; alkyl C), 45–65 (methoxyl C; methoxyl/N-alkyl), 65–95 (O-alkyl systems), 95–110 (anomeric C), 110–140 (unsubstituted aromatic C and olefinic C), 140–160 (phenols and substituted aromatic C), and 160–190 ppm (aliphatic and aromatic carboxyl C; C in amidic groups; carboxyl/amide). The areas of alkyl (0–45 ppm) and aromatic (160–110 ppm) C were summed to represent the fraction of hydrophobic carbons (HB) in the HLA, whereas the summation of relative areas in intervals related to polar groups (190–160 and 110–145 ppm) indicated the fraction of hydrophilic carbons (HI). The ratio of HB and HI were used to calculate the hydrophobicity index (HB/HI).
Initial root growth assessment of HLA-treated seedlings
Maize seedlings were treated with increasing concentrations of HLA solutions in a hydroponic trial to determine the optimal concentration for plant root development. Firstly, maize seeds were disinfected by immersion in NaCl solution (0.5%) for 30 min, then rinsed and soaked in water for 6 h. Subsequently, the seeds were kept on wet filter paper and germinated in the dark at 28°C for 2 days to reach the root length of 1 cm and used for the hydroponic trial. Three replicates each were set up for different concentrations of HLA, and each replicate was formed from approximately ten plants. Four-day-old maize seedlings were transferred to pots containing a minimal medium solution (2 mmol L−1 CaCl2) enriched with 0, 20, 40, 80, and 160 mg L–1 of HLA and adjusted to pH 6.0 using 0.01 mol L–1 NaOH and HCl. Solutions were replaced every two days. After seven days in hydroponic culture, plants were harvested to evaluate the root area using the image processing software ImageJ® and the number of lateral roots was manually counted. The plants were dried in a forced-air circulation oven at 65°C until a constant weight was achieved and then weighed in a four-digit analytical balance to determine the root dry mass.
Proton pump activity measurements
The activity of the proton pump was assessed by measuring the changes in the minimal medium pH, according to the method described by Aguiar et al. (Citation2013). Ten four-day-old maize seedlings were transferred to pots containing the minimal medium solution (2 mmol L−1 CaCl2) with or without the optimal HLA concentration (40 mg L−1) for 48 h. Then, seedlings were transferred to new pots containing the minimal medium solution at an initial pH value of 7.0 and monitored for 120 min. The roots were cut from the plants and kept in a forced air circulation oven at 65°C until a constant weight was achieved and then weighed using an analytical balance to determine the root dry mass. The amount of H+ extruded by plants, related to the proton pump activity, was measured using a calibrated pH-meter, equipped with a glass electrode (MA 036, Marconi Equipamentos Para Laboratórios Ltda, Piracicaba, SP, Brazil). The amount was expressed as H+ extruded per gram of dry root mass.
Soil amendment with silicate rock powder
The SRP used in this study was derived from biotite deposits from Guanambi, Bahia, and Brazil. X-ray diffraction and petrographic analyses showed that the material is a biotite syenite composed of alkaline feldspar microcline (77%), biotite (11%), and clinopyroxene (11%) (Rosa Citation1999). Biotite syenite consists of SiO2 (539 mg g−1), Al2O3 (179 mg g−1), K2O (125 mg g−1), Fe2O3 (70 mg g−1), CaO (34 mg g−1), MgO (19 mg g−1), Na2O (18 mg g−1), TiO2 (7.6 mg g−1), P2O5 (6.7 mg g−1), BaO (6.0 mg g−1), ZnO (2.1 mg g−1), and MnO (0.6 mg g−1) (Santos Citation2016; Dos Santos et al. Citation2021). The rocks were crushed in a ball mill and passed through a 0.100 mesh sieve to obtain uniform SRP, incorporated into the soil samples in doses equivalent to 0, 600, 1200, 1800, and 2400 kg ha−1 on a dry-weight basis. These rates correspond to 0, 75, 150, 225, and 300 kg ha−1 of K2O, which is considered the well-known range rate of K fertilization recommended for maize production in the Cerrado region. The K content was chosen as a reference parameter due to its high concentration in the SRP and because Brazil imports about 97% of K, as muriate of potash, for use in agriculture (AMA Brasil Citation2019). The soil used in the experiment (B horizon) was a Dystrophic Red-Yellow Latosol (EMBRAPA Citation2013), corresponding to Oxisol in USDA soil taxonomy and WRB/FAO (Silva et al. Citation2016). The soil was collected from the experimental farm of Embrapa Cerrados, (Brazilian Federal District, 15° 36ʹ 25ʺ S, 47° 44ʹ 49ʺ W, 1,014 asl). The samples were air-dried, passed through a 2-mm sieve, and chemically analyzed according to the method described by EMBRAPA (Citation2009). The procedure for chemical analysis of soil property is described in Supplemental information SI 2 and SI 3. The Cerrados region in Brazil is affected by soil degradation due to human activity and the climate conditions of long dry seasons (Gomes et al. Citation2019), leading to low total organic carbon (TOC) (Supplemental information 3). The soil was mixed homogenously with SRP and transferred to 14-dm3 pots and kept in a greenhouse for 60 days before planting.
Pot experiment of HLA-treated maize seedlings in SRP-amended soil
A pot experiment was conducted under a completely randomized design under greenhouse conditions. Maize seeds were disinfected and rolled up in germination paper soaked with the optimal HLA concentration (40 mg L−1) for 48 h. The seedlings were carefully transferred to pots filled with soil amended with or without SRP. As previously mentioned, different ranges of SRP (0, 600, 1200, 1800, and 2400 kg ha−1) were used as treatments. A control treatment without HLA was also performed. Each treatment was conducted in triplicates, and every pot contained ten plants. Temperature and moisture were monitored and controlled to avoid the exposure of seedlings to abiotic stress. While the temperature was between 25 and 35°C during the experiment and the moisture range was between 60 and 70% of water holding capacity. All treatments were supplied with N at the rate of 100 kg ha−1 by adding appropriate amounts of (NH4)2SO4 during the experiment. In the treatments with HLA-treated seeds, 100 mL of 40 mg L−1 HLA solution was applied on the 15th and 25th days after planting The mode of the application of HLA was foliar spraying.
Plant analysis
After 45 days of planting, plant height and stem diameter were measured using a digital calliper. Subsequently, the plants were collected, and their total fresh weight was measured. Roots were separated from the aboveground biomass, and washed abundantly with water, using carefully small jets of water to avoid any damage before the fresh weight of the roots was determined. Then, all samples were oven-dried at 65°C to determine their dry weight. Plant tissues were ground (2-mm mesh sieve) using a knife mill and analyzed for the elemental composition according to standard methods described by the EMBRAPA (Citation2009). Briefly, N content was determined using a micro-Kjeldahl procedure (Guebel et al. Citation1991) after digestion with H2SO4-H2O2. For P, the samples were similarly digested with H2SO4-H2O2, and the concentration was determined using the molybdenum blue method (Crouch and Malmstadt Citation1967). The levels of Ca, Mg, K, Zn, Fe, Cu, Ni, and Mn were analyzed after digestion with HNO3-HCl and determined using a microwave-induced plasma atomic emission spectrometer (MP-AES 4200, Agilent, Palo Alto, CA, USA).
Data analysis
The elemental composition; total, carboxylic, and phenolic acidities; and FTIR and CPMAS 13C-NMR spectroscopies were qualitatively assessed. The concentration–response test was carried out using a completely randomized design with ten replicates. The experiment involving the addition of HLA in plants grown in soils enriched with SRP also followed a completely randomized design with three replicates, in a 5 × 2 factorial scheme: with five doses of SRP (0, 600, 1200, 1800, and 2400 kg ha−1), with or without HLA. After verifying the normality by the Shapiro–Wilks test, the concentration–response and plant analyses were subjected to analysis of variance tests. The significative mean of different treatments was assessed using the Tukey test of honestly significant difference (p < 0.05). All analyses were performed using the R software (R Core Team Citation2019). All results of plant parameters were reported as means ± SD based on the two-way analysis of variance for different treatments at different SRP rates (0, 600, 1200, 1800, and 2400 kg ha−1) and with HLA application. Data obtained from the fifteen performed measurements in study were analyzed using the methods of principal component analysis (PCA). We calculated the contribution of PCA loadings for each variable to the selected principal components. Total contribution of a variable for two dimensions is calculated as per ((C1 * E1) + (C2 * E2))/(E1 + E2), where: C1 and C2 represent the contributions of a variable to principal components 1 (PC1) and 2 (PC2). E1 and E2 represent the eigenvalues of principal components 1 and 2. The statistical test was conducted using the R packages, ‘agrocolae’, ‘car’, ‘multicomp’ and ‘princomp’.
Results
Characterization of HLA
shows the chemical composition of the HLA used in our study. The HLA derived from bovine manure vermicomposting had high amounts of carbon (583 g kg−1) and O (299 g kg−1) and a low amount of H (73 g kg−1) and N (45 g kg−1). . The atomic O/C ratio of 0.4 indicated the presence of oxygenated functional groups (e.g. carboxylic groups). Most of the total acidity determined in HLA (76%) was attributed to the presence of carboxylic groups, whereas phenolic groups represented only a small fraction (24%) ().
Table 1. Elemental composition, atomic ratio, total, carboxylic and phenolic acidities of humic-like acid isolated from vermicompost of bovine manure.
The FTIR spectrum of HLA displayed a typical profile of weak and polyprotic organic acids with seven prominent absorption bands (Supplemental Information 4a). The band next to 3500 cm−1 is commonly assigned to O–H and N–H, stretching from phenols and amines. The well-defined band at 2950 cm−1 is due to symmetric stretching of aliphatic C–H bonds, mainly belonging to methyl groups (CH3), whereas the band near 2800 cm−1 is associated with aldehydes. The symmetric stretching at 1650 cm−1 is due to C = O bonds from carbonyl groups, possibly COO, whereas the band in 1450 cm−1 can be attributed either to amide II groups or to the deformation of aliphatic C–H bonds (CH2 and CH3) in quinine, probably dominated by lignin and protein-like structures originated from bovine manure. Bands at 1280 and 1030 cm−1 can be assigned to C–O bonds in carboxyl, ester, or phenolic groups and to C–O stretching in polysaccharides, respectively.
The CPMAS 13C-NMR spectrum of HLA, shown in Supplemental Informatoin 4b, indicates an accumulation of waxes, lipids, cutin, and suberin in HLA because peaks in the region of aliphatic carbon, in particular, signals at 26 and 31 ppm are attributed to CH2 groups. The signal at 57 ppm is associated with the methoxy lignin substituent or C–N bonds in amino acids (Hatcher Citation1987). The signal at 73 ppm is related to cellulose and hemicellulose molecules (Aguiar et al. Citation2013). The peak at 106 ppm is attributed to the anomeric C of cellulose, whereas those at 117, 130, and 154 ppm represent structural C from lignin (Wilson Citation1987). The signals at 176 and 197 ppm are associated with carboxyl (DiDonato and Hatcher Citation2017) and carbonyl (Pavia et al. 2010) groups.
The CPMAS 13C-NMR spectrum was integrated into seven chemical shift intervals, according to the method described by Balmori et al. (Citation2014), as shown in . The region referring to alkyl C, mainly CH2 and CH3 (0–45 ppm), accounted for 33.3% of the spectrum, whereas N-alkyl groups, aliphatic C (45–65 ppm), and O-alkyl systems (65–95 ppm) were responsible for 16.7%. The anomeric C (95–110 ppm) represented 5.6%, and the unsubstituted aromatic and olefinic C (110–140 ppm) accounted for 13.3%. The region of phenols and substituted aromatic C (140–160 ppm) represented 5.6% of the spectrum, whereas 8.9% was attributed to aliphatic C, aromatic carboxyl C, and C in amidic groups (160–190 ppm).
Table 2. Relative abundances of different carbon structures measured by Cross-polarization magic angle spinning 13C nuclear magnetic resonance (CPMAS 13C-NMR) in humic-like acid extracted from vermicompost of bovine manure.
The signals at 26 and 31 ppm indicate the presence of paraffinic structures such as cutin and suberin that may corroborate the observed hydrophobicity. Moreover, CPMAS 13C-NMR signals at 57, 117, 130, and 154 ppm indicate the presence of lignin, which also contributes to increasing the HLA hydrophobicity (0.98), shown in . 0.98 in HB/HI ratio is well balanced between hydrophobic and hydrophilic parts in comparison with extracted HAs from leonardite, coal and lignite, which reaches the range of 2.49–3.63 (Barone et al. Citation2019; Zhang et al. Citation2021; lVerrillo et al. Citation2022).
Effect of HLA on initial maize root growth in a preliminary trial
The results obtained from the hydroponic trial showed that HLA increased (p < 0.05) root area, dry root mass, and the number of lateral roots at concentrations up to 40 mg L−1 (). Higher concentrations, however, induced opposite responses. A 40 mg L−1 HLA concentration resulted in the highest number of lateral roots ((a)), with an increase of 49% compared with the control. Based on the results, (1) no statistical difference was observed in the increase in root area and dry root mass upon HLA treatment at concentrations of 40 and 80 mg L−1 ((b,c)), and (2)a higher number of lateral roots ((a)) was obtained using a 40 mg L−1 HLA solution. Thus, the concentration of 40 mg L−1 HLA solution was considered the most efficient and used for subsequent experiments.
Figure 1. Effect of different concentrations of humic-like acids extracted from vermicompost in the initial maize root development. The number of lateral roots ((a)); Root area ((b)); Dry root mass ((c)). Different letters indicate statistical differences (Tukey test p<0.05). Columns represent the means ± standard deviation.
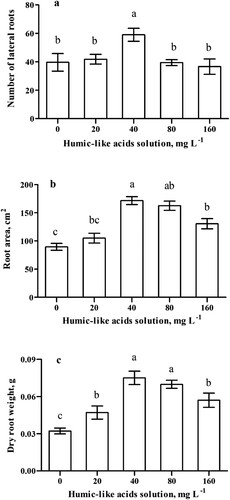
The influence of HLA on the acidification of aqueous growth medium and proton pumps of maize seedlings was examined (Supplemental information 5a). Based on the previous result in the hydroponic trial, seen in , the concentration of 40 mg L−1 HLA was used for this analysis. Compared with the control, higher acidification was observed in the extracellular H+ flux of the HLA-treated plants (Supplemental information 5a). With time, a higher proton pump activity was observed in the plants treated with HLA (Supplemental information 5b).
Biometric analysis and nutrient content in maize plants
shows the result of the pot experiment conducted to analyze the effect of different rates of SRP with/without HLA (40 mg L−1) on plant growth. The amount of SRP had a significant effect on the stem diameter, plant height, total weight, and root weight (p < 0.05), but there were also SRP levels, for which HLA addition had no or negative impacts on plant growth parameters. The treatment with 2400 kg ha−1 SRP resulted in the highest values for stem diameter, plant height, and total fresh weight, whereas that with 1200 kg ha−1 SRP resulted in the highest fresh root weight. The sample with no SRP had the lowest values in all the tested parameters. No significant difference was observed in the total dry weight from 1200 to 2400 kg ha−1.
Figure 2. Effects of humic-like acids (40 mg L−1) extracted from vermicompost on maize seedlings: (a) Stem diameter (mm); (b) Plant height (cm); (c) Root fresh weight (g); (d) Root dry weight (g); (e) Total fresh weight (g) and (f) Total dry weight (g) grown in a weathered soil under increasing rock powder doses. Asterisk indicates statistical differences (Tukey test p<0.05) between plants with or without HLA at the silica rock powder rate. The symbols of ∗ and ∗∗ indicate significant differences at the 5% and 1% levels, respectively. Bars represent standard deviation.
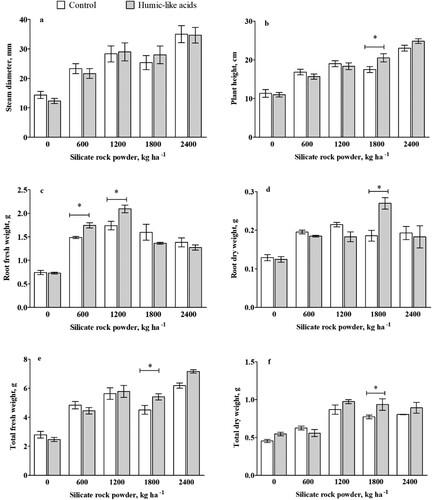
Compared with the treatments of sole SRP application, co-application of SRP and HLA showed significantly higher results in all parameters except for the stem diameter (p < 0.05). but there were also SRP levels, for which HLA addition had no or negative impacts on plant growth parameters. Plants treated with a combination of SRP (1800 kg ha−1) and HLA (40 mg L−1) had 17.1% higher plant height than those treated with only SRP ((b)). Compared to maize treated with only SRP, the addition of HLA significantly increased the fresh weight of roots by 14.6% and 16.9% in plants treated with 600 and 1200 kg SRP ha−1, respectively ((c)). Regarding the dry weight of roots, however, a significant difference is not observed in these treatments but plants treated with 1800 kg SRP ha−1 ((d)). The total fresh weight and dry weight of plants treated with a combination of HLA (40 mg L−1) and SRP (1800 kg ha−1) were significantly higher (p < 0.05) than those treated with only SRP ((e,f)). The interaction of SRP and HLA on root fresh and dry weight and total fresh weight was significant (p < 0.05). The interactive effect of SRP and HLA on plant height was significant at a p < 0.1 level of confidence.
and show the effects of different treatments on the nutrient content of plants under weathered soil conditions. Sole SRP application significantly increased all nutrients except for Mg, Cu, Fe, compared with the no SRP treatment. Treatments with SRP application at medium and high rates (1200, 1800, and 2400 kg ha−1) showed a significantly high content of macronutrients (N, K, and P) (). The additional use of HLA increased the N content by 5% in the plants grown in SRP-enriched soils at rates of 1200, 1800, and 2400 kg ha−1 (a). The plants raised under the combination treatment of 40 mg L−1 HLA and SRP at the rates of 600, 1200, 1800, and 2400 kg ha−1 showed high P content with 16.3%, 11.3%, 9.0%, and 14.4%, respectively ((b)). HLA addition provided significant increased K contents by 8.0% and 14.0% using SRP at the rates of 600 and 1200 kg ha−1, respectively ((c)). A similar trend was observed in Ca, where the co-application of 40 mg L−1 HLA and SRP at rates of 1200, 1800, and 2400 kg ha−1 resulted in increase in the Ca contents by 65.6%, 72.4%, and 32.7%, respectively ((d)). The content of Cu also increased at all rates of SRP in the HLA treatments ((a)), ranging from 24.6% (0 kg ha−1 of SRP) to 90.0% (600 kg ha−1 of SRP). Significative differences in Zn content ((b)) were detected in HLA-treated plants with SRP rates of 1200 and 1800kg ha−1, which increased Zn contents by 117.7% and 52.2%, respectively. In the case of Mn ((c)), SRP rates of 1800 and 2400 kg ha−1 combined with HLA increased Mn contents by 19.6% and 29.9%, respectively. The highest Fe content was seen at the rate of 0 kg ha−1 of SRP. The statistical analysis of the interactive effect of the two factors (SRP rates and co-application with HLA) showed that the results were statistically significant for all the tested nutrients except for Magnesium (p < 0.05), as seen in Supplemental Information 6 (SI 6).
Figure 3. The nutrient content of nitrogen, phosphorous, potassium, calcium, and magnesium in maize plants grown in a weathered soil treated silicate rock powder (0, 600, 1200, 1800, 2400 kg ha−1) with or without and humic-like acids (40 mg L−1). Asterisk indicates statistical differences (Tukey test p<0.05) between plants with or without HLA at the same silica rock powder rate. Bars represent standard deviation.
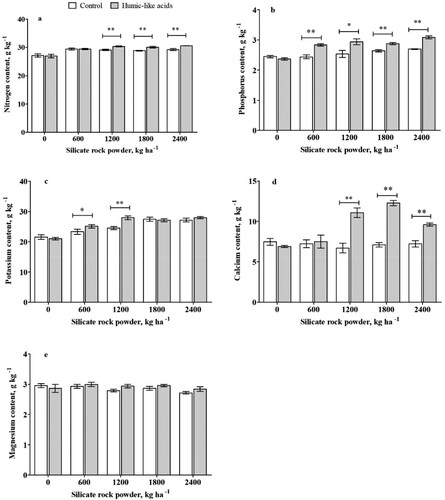
Figure 4. The nutrient content of copper, zinc, manganese, and iron in maize plants grown in a weathered soil treated silicate rock powder (0, 600, 1200, 1800, 2400 kg ha−1) with or without and humic-like acids (40 mg L−1). Asterisk indicates statistical differences (Tukey test p<0.05) between plants with or without HLA at the same silica rock powder rate. The symbols of ∗ and ∗∗ indicate significant differences at the 5% and 1% levels, respectively. Bars represent standard deviation.
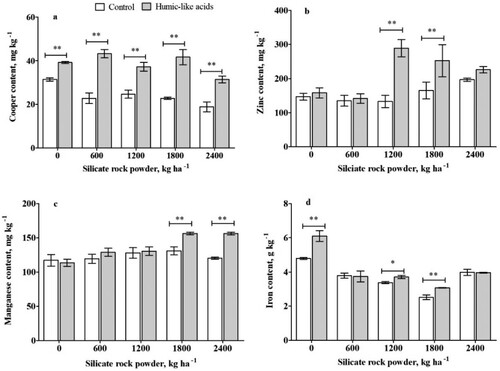
PCA is a dimensionality-reduction method of multivariate analysis which enable to discover underlying patterns in a dataset. In our study, the variance of 69.8% can be explained by dimensions 1 and 2, seen in Supplemental Information 7 (SI 7). The variables of the highest contributions in the dimension 1 are total fresh weight, N, and K. Those variables are highly associated and form a cluster, observed in SI 7. In the contrast, Cu and Mg are other variables that highly contribute the dimension 2.
Discussion
Sustainable use of rock phosphate is necessary to preserve the limited global reserves. Direct application of rock phosphate is often recommended for perennial crops and not for rapidly growing crops due to the slow dissolution of rock phosphate (Le Mare Citation1991). The present work shows the positive effect of SRP in combination with HLA on maize growth in weathered soil. A better understanding of the chemical composition and bioactivity of humic substance (HS) and humic-like substance (HLS) is needed to identify the factors underlying this effect. The stimulatory effect on plant development is closely linked with the chemical compositions of HS and HLS (Vaccaro et al. Citation2015). Therefore, information regarding the chemical structure and functional groups is necessary to evaluate HS and HLS efficiency in stimulating plant development. The molecular characteristics of the HLA used in this study, such as hydrophobicity, were revealed using 13C-NMR, and the results resemble those observed in other works in which the effect of HLA on plant growth was associated with induction of PM H+-ATPase and lateral root emergence (Aguiar et al. Citation2013). Although hydrophobic domains protect biomolecules with hormonal activity from enzymatic degradation, organic acids exuded by plants might dismantle these structures and make nutrients accessible to roots, promoting plant growth (Nardi et al. Citation2000, Citation2002; Canellas et al. Citation2008; Pizzeghello et al. Citation2020). It is noteworthy that a certain amount of aliphatic compounds were detected with peaks of 1650 and 2950 cm−1 in the FTIR spectrum, and a relatively large proportion of aliphatic and methoxyl carbons were detected in the NMR spectrum (Supplemental Information 4). Bioactive compounds in the HLA can also access the cytoplasm and lead to small changes in pH, with effects on several essential cellular processes, including the induction of enzymatic activities (Han and Burgess Citation2010).
In plants, HLAs mainly cause root elongation and lateral root emergence by activating the plasma membrane (PM) H+-ATPase and proton pump through phytohormonal modulations (Canellas et al. Citation2002; Nardi et al. Citation2018; Zandonadi et al. Citation2019). The proton pump is linked to the acidification of the apoplasts resulting in the loosening of cell walls and root growth (Frias et al. Citation1996). The activation of H+-ATPase also improves plant nutrition by increasing the electrochemical proton gradient that drives ion transport across cell membranes (Morsomme and Boutry Citation2000). Root elongation and lateral root emergence, shown in , are positive morphological impacts of HLA. This effect is supported by the acid growth theory – PM H+-ATPase is activated by small molecules present in HLA, which are endowed with auxin-like activity (Musculo et al. Citation1998, Citation1999; Canellas et al. Citation2002; Nardi et al. Citation2002). The PM H+-ATPase directs H+ extrusion through the plasma membrane via ATP hydrolysis, which in turn generates an electrochemical gradient and acidifies the apoplast, activating pH-sensitive enzymes responsible for loosening the cell wall, resulting in cell volume expansion and elongation (Zandonadi et al. Citation2019). Our work shows a decrease in pH in the minimal medium containing HLA-treated plants (Supplemental information 2), indicating an extensive efflux of H+ from the roots and higher activity of PM H+-ATPase. According to Aguiar et al. (Citation2013), two main factors may influence the acidification of the medium solution: the production of respiratory CO2 by roots and the extrusion of H+ by H+-ATPase in the plasma membrane and cell vacuoles. Therefore, we suggest that the pH decrease in the solution was due to proton pump activity and proton extrusion. It is worth mentioning that extrusion increased exponentially as pH decreased. The HLA-treated plants recorded the highest values for plant growth parameters (SI 5b), indicating a marked beneficial effect of HLA on acid growth induction, as described previously by Canellas et al. (Citation2002).
Finding an optimal HLA concentration for plant growth is one of the crucial factors for its application. In our study, it showed the reversal of the trend for higher concentrations regarding the root area and the number of the lateral roots (), which are often observed in other previous works (Canellas et al. Citation2002; Khan et al. Citation2018). This could be resulted from that (1) HLA acts as a plant hormone, which requires a low concentration and (2) the high concentration of HA would cause insolubilization of nutrient (Khan et al. Citation2018).
The co-application of SRP and HLA in our study resulted in positive nutrient uptake () and the result of PCA analysis (SI 6) confirmed that the nutrient contents such as N and K are highly associated with morphological parameters (e.g. total dry weight, plant height, dry root weight). The activation of H+ flux by HLA is also crucial for energizing secondary transporters, resulting in enhanced nutrient uptake, root growth promotion, and absorption of water (Canellas and Olivares Citation2014). Increased N content was shown in our study. The effect on the root expression of N assimilatory enzymes (Zanin et al. Citation2019) promotes the expression of the nitrate transporter ZmNRT2s (Vujinovic et al. Citation2020), which could result in increased N content in plants treated with HLA and HA.
An increase in the flux of H+ due to enhanced PM H+-ATPase activity mediated by HLA (Zandonadi et al. Citation2019) triggers P mobilization from insoluble forms. Furthermore, HLA plays an important role in stimulating the activity of solubilizing microorganisms and complexing cations bound to phosphate (Zanin et al. Citation2019). HLA also increases the activity of other microorganisms, and therefore, respiratory CO2 production, acidifying the rhizosphere, which may further solubilize P from the SRP by altering the root–soil–rock interface (Rosa et al. Citation2018). Finally, HLA influences gene expression of the high-affinity P transporter LePT2, aiding plant uptake of P from soil (Jindo et al. Citation2016). Enhanced gene expression by HLA also increases Ca2+ uptake, such as by modulating the rice putative voltage-dependent Ca2+ channel OsTPC1, which increases Ca2+ uptake upon exposure to increased acidity (Ramos et al. Citation2015).
Concerning cationic micronutrients, the decrease in soil pH of the HLA-treated plants increased nutrient availability. Under these conditions, the speciation of metal complexes such as chelating was altered, leading to higher cation availability from both SRP and the soil itself. Additionally, increased microbial activity might have accelerated the dissolution of minerals from SRP via increased root exudation of organic (e.g. siderophores) and inorganic compounds (CO2/H2CO3) following HLA application.
However, it should be highlighted that our study shows only the result of the nutrient contents in the plant tissues and not the total nutrient uptake by the plants. The effect of the coapplication of HLA and SRP on nutrient transporter and total nutrient uptake should be further examined. In addition to the plant uptake, it would be also interesting to explore the study of the change of available P and K in soil after the HLA application.
Clearly, differentiating between HA and HLA in chemical structure and functionality for soil ecosystems and plant growth is challenging. Based on recent findings (Bento et al. Citation2020; Dos Santos et al. Citation2020), several different characteristics can be described: (1) HA derived from soil contains mostly aromatic carbons, whereas both aromatic and aliphatic carbons contribute to the chemical structure of the HLA used in this study; (2) HA originating from the soil is more hydrophilic, whereas HLA from other origins such as compost, biochar and hydrocharis more hydrophobic; and (3)regarding bioactivity, HLA has more influence on plant growth than HA.
However, it should be noted that HLA and HA have a wide range of different properties that are strongly affected by the original materials (Hiemstra et al. Citation2013). Fuentes et al. (Citation2018) proposed a new methodology to evaluate the quality and quantity of HA and HLA in different organic materials by using discriminant analyses. However, it requires specialized laboratory equipment for UV-visible, FTIR, fluorescence, and elemental analyses – a cost that is a bottleneck in emergent and developing countries. Further research into practical and affordable methods for correlating HLA/HA chemical structure and plant growth promotion must be conducted.
Conclusion
This study demonstrated that maize seeds treated with a 40 mg L−1 HLA solution have more lateral roots, root area, and dry matter. In addition, roots grown in this HLA concentration (40 mg L−1) yielded more significant acidification of the minimal medium, which is aligned with the increase in H+ efflux through the activity of the PM H+-ATPase. When grown in the weathered soil with 1800kg ha−1 of SRP, HLA application improved plant growth and nutrient content. In future, a spectroscopic analysis of the mixture of SRP and HLA should be conducted to understand the possible chemical interactions. Thus, we conclude that HLAs are a sustainable and cost-effective means for soil fertilization. They stimulate the development of maize seedlings and increase their efficiency in absorbing nutrients from a weathered soil amended with SRP. The co-application of SRP and HLA strongly benefits the plant nutrition of short-duration crops.
Supplemental Material
Download MS Word (543.1 KB)Acknowledgements
Keiji Jindo wishes to acknowledge financial support (3710473400).
Disclosure statement
No potential conflict of interest was reported by the author(s).
Additional information
Notes on contributors
Jader Galba Busato
Jader Galba Busato is an associate professor at University of Brasilia in Brazil. His main research is organic waste.
Luiz Fernando dos Santos
Luiz Fernando dos Santos is an associate professor at University of Brasilia in Brazil. Environmental management for the recovery of degraded areas and remediation of contaminated soils are his scientific topic.
Alessandra Monteiro de Paula
Alessandra Monteiro de Paula is an associate professor at University of Brazilia in Brazil and her topics are plant-microorganism interaction, soil quality, and greenhouse gas emissions.
Fernando Fabriz Sodré
Fernando Fabriz Sodré is an associate professor at University of Brazilia in Brazil and his research is related to the chemical analysis in the environmental and agricultural sciences.
Aline Lima de Oliveira
Aline Lima de Oliveira is an associate professor at University of Brazilia in Brazil and she is interested in the structure and dynamics of peptide and proteins by NMR and quantification of organic molecules by NMR
Leonardo Barros Dobbss
Leonardo Barros Dobbss is an associate professor at dos Vales do Jequitinhonha e Mucuri, Unai in Brazil. His main topics are humic substance, biostimulant and organic materials.
Éder de Souza Martins
Éder de Souza Martins is a senior researcher at Embrapa Cerrado in Brazil and is interested in the analysis of rock phosphate.
Keiji Jindo
Keiji Jindo is a senior researcher at Wageningen University & Research in The Netherlands. His main research themes are the circular economy, precision agriculture and food security.
References
- Adeleke R, Nwangburuka C, Oboirien B. 2017. Origins, roles and fate of organic acids in soils: a review. S Afr J Bot. 108:393–406.
- Aguiar NO, Olivares FL, Novotny EH, Dobbss LB, Balmori DM, Santos-Júnior LG, Chagas JG, Façanha AR, Canellas LP. 2013. Bioactivity of humic acids isolated from vermicomposts at different maturation stages. Plant Soil. 362:161–174.
- Aliyu IA, Yusuf AA, Uyovbisere EO, Masso C, Sanders IR. 2019. Effect of co-application of phosphorus fertilizer and in vitro-produced mycorrhizal fungal inoculants on yield and leaf nutrient concentration of cassava. PLoS ONE. 14(6):e0218969. doi:10.1371/journal.pone.0218969.
- AMA Brazil. 2019. Production and imports of fertilizer commodities for Brazil (2008-2018). [accessed 2020 Dec]. http://amabrasil.agr.br/web/portfolio-item/producao-e-importacao-de-fertilizantes.
- Balmori MD, Spaccini R, Aguiar N, Novotny EH, Olivares FL, Canellas LP. 2014. Molecular characteristics of humic acids isolated from vermicomposts and their relationship to bioactivity. J of Agri and Food Chem 62:11412–11419.
- Balmori DM, Domínguez CYA, Carreras CR, Rebatos SM, Farías LBP, Izquierdo FG. 2019. Foliar application of humic liquid extract from vermicompost improves garlic (Allium sativum L.) production and fruit quality. Int J Recycl Org Waste Agric. 8:103–112.
- Barone V, Bertoldo G, Magro F, Broccanello C, Puglisi I, Baglieri A, Cagnin M, Concheri G, Squartini A, Pizzeghello D, et al. 2019 Jun 18. Molecular and morphological changes induced by leonardite-based biostimulant in beta vulgaris L. Plants (Basel). 8(6):181. doi:10.3390/plants8060181.
- Bento LR, Castro AJR, Moreira AB, Ferreira OP, Bisinoti MC, Melo CA. 2019. Release of nutrients and organic carbon in different soil types from hydrochar obtained using sugarcane bagasse and vinasse. Geoderma. 334:24–32. doi:10.1016/j.geoderma.2018.07.034.
- Bento LR, Melo CA, Ferreira OP, Moreira AB, Mounier S, Piccolo A, Spaccini R, Bisinoti MC. 2020. Humic extracts of hydrochar and Amazonian dark earth: molecular characteristics and effects on maize seed germination. Sci Total Environ. 15(708):135000.
- Canellas LP, Olivares FL, Okorokova-Façanha AL, Façanha AR. 2002. Humic acids isolated from earthworm compost enhance root elongation, lateral root emergence and plasma membrane H+-ATPase activity in maize roots. Plant Physiol. 130:1951–1957.
- Canellas LP, Olivares FL. 2014. Physiological responses to humic substances as plant growth promoter. Chem and Biol Technol in Agric. 1:3.
- Canellas LP, Teixeira Junior LRL, Dobbss LB, Silva CA, Medici LO, Zandonadi DB, Façanha AR. 2008. Humic acids cross interactions with root and organic acids. Annals Applied Biol. 153(2):157–166.
- Ciceri D, Oliveira M, Stokes RM, Skorina T, Allanore A. 2017. Characterization of potassium agrominerals: correlations between petrographic features, comminution and leaching of ultrapotassic syenites. Miner Eng. 102:42–57.
- Crouch SR, Malmstadt HV. 1967. Mechanistic investigation of molybdenum blue method for determination of phosphate. Anal Chem. 39(10):1084–1089. doi:10.1021/ac60254a027.
- DiDonato N, Hatcher PG. 2017. Alicyclic carboxylic acids in soil humic acid as detected with ultrahigh resolution mass spectrometry and multi-dimensional NMR. Organic Geochem. 112:33–46. doi:10.1016/j.orggeochem.2017.06.010.
- Ditta A, Muhammad J, Imtiaz M, Mehmood S, Qian Z, Tu S. 2018. Application of rock phosphate enriched composts increased nodulation, growth and yield of chickpea. Int J Recycl Org Waste Agric. 7:33–40.
- Dos Santos JV, Fregolente LG, Moreira AB, Ferreira OP, Mounier S, Viguier B, Hajjoul H, Bisinoti MC. 2020. Humic-like acids from hydrochars: study of the metal complexation properties compared with humic acids from anthropogenic soils using PARAFAC and time-resolved fluorescence. Sci Total Environ. 722:137815.
- Dos Santos LF, Sodré FF, Martins É, Figueiredo C, Busato JG. 2021. Effects of biotite syenite on the nutrient levels and electrical charges in a Brazilian Savanna Ferralsol. Pesqui Agropecu Trop. doi:10.1590/1983-40632021v5166691.
- Du Jardim P. 2015. Plant biostimulants: definition, concept, main categories and regulation. Sci Horticul. 196:3–14. doi:10.1016/j.scienta.2015.09.021.
- Embrapa – Empresa Brasilera De Pesquisa Agropecuária. 2009. Manual de análises químicas de solo, plantas e fertilizantes. Brasília: EMBRAPA Informação Tecnológica; p. 627.
- Embrapa -Empresa Brasileira De Pesquisa Agropecuária. 2013. Sistema Brasileiro de Classificação de Solos. Brasília: Centro Nacional de Pesquisa de Solos. p. 353.
- Frias I, Caldeira MT, Perez-Castineira JR, Navarro-Avino JP, Culianez-Macia FA, Kuppinger O, Stransky H, Pages M, Hager A, Serrano R. 1996. A major isoform of the maize plasma membrane H+-ATPase: characterization and induction by auxin in coleoptiles. Plant Cell. 8(9):1533–1544. doi:10.1105/tpc.8.9.1533.
- Fuentes M, Baigorri R, González-Gaitano G, García Mina JM. 2018. New methodology to assess the quantity and quality of humic substances in organic materials and commercial products for agriculture. J Soils Sediments. 18:1389–1399.
- Gomes L, Simões SJC, Dalla Nora EL, de Sousa-Neto ER, Forti MC, Ometto JPHB. 2019. Agricultural expansion in the Brazilian Cerrado: increased soil and nutrient losses and decreased agricultural productivity. Land (Basel). 8(1):12.
- Guebel DV, Nudel BC, Giulietti AM. 1991. A simple and rapid micro-Kjeldahl method for total nitrogen analysis. Biotechnol Tech. 5:427–430. doi:10.1007/BF00155487.
- Han J, Burgess K. 2010. Fluorescent indicators for intracellular pH. Chem Rev. 110:2709–2728. doi:10.1021/cr900249z.
- Harley AD, Gilkes RJ. 2000. Factors influencing the release of plant nutrient elements from silicate rock powders: a geochemical overview. Nutr Cycling Agroecosyst. 56:11–36. doi:10.1023/A:1009859309453.
- Hatcher PG. 1987. Chemical structure studies of natural lignin by dipolar dephasing solid-state 13C nuclear magnetic resonance. Org Geochem. 11:31–39.
- Hiemstra T, Mia S, Duhaut PB, Molleman B. 2013. Natural and pyrogenic humic acids at goethite and natural oxide surfaces interacting with phosphate. Environmen Sci Technol. 47(16):9182–9189. doi:10.1021/es400997n.
- Houghton J, Ding Y, Griggs D, Noguer M, Van Der Linden P, Dai X, Maskell K, Jindo K, Soares TS, Peres LEP, et al. 2016. Phosphorus speciation and high-affinity transporters are influenced by humic substances. J Plant Nutr Soil Sci. 179:206–214.
- IHSS - International Humic Substance Society. 2019. [accessed 2019 Nov]. http://humic-substances.org/isolation-of-ihss-soil-fulvic-and-humic-acids.
- Jindo K, Soares TS, Peres LEP, Azevedo IG, Aguiar NO, Mazzei P, Spaccini R, Piccolo A, Olivares FL, Canellas LP. 2016. Phosphorus speciation and high-affinity transporters are influenced by humic substances. J Plant Nutr Soil Sci. 179:206–214. doi:10.1002/jpln.201500228.
- Khan RU, Khan MZ, Khan A, Saba S, Hussain F, Jan IU. 2018. Effect of humic acid on growth and crop nutrient status of wheat on two different soils. J Plant Nutr. 41(4):453–460. doi:10.1080/01904167.2017.1385807.
- Krahl LL, Paz SPA, Angélica RS, Valadares LF, Sousa-Silva JC, Marchi G, Martins ES. 2020. Successive off take of elements by maize grown in pure basalt powder. Afr J Agric Res. 15(2):229–239.
- Le Mare P. 1991. Rock phosphates in agriculture. Exp Agric. 27(4):413–422. doi:10.1017/S0014479700019396.
- Lubkowski K. 2016. Environmental impact of fertilizer use and slow release of mineral nutrients as a response to this challenge. Pol J Chem Technol. 18(1):72–79. doi:10.1515/pjct-2016-0012.
- Maharana R, Basu A, Dhal NK, Adak T. 2021. Biosolubilization of rock phosphate by Pleurotus ostreatus with brewery sludge and its effect on the growth of maize (Zea mays L.). J Plant Nutr. 44(3):395–410.
- Manning DAC, Baptista J, Limon MS, Brandt K. 2017. Testing the ability of plants to access potassium from framework silicate minerals. Sci Total Envir. 574:476–481.
- Marchi G, Guelfi-Silva DR, Malaquias JV, Guilherme LRG, Spehar CR, Martins ES. 2020. Solubility and availability of micronutrients extracted from silicate agrominerals. Pesqui Agropecu Bras. v.55:e00807.
- Mohammed SMO, Brandt K, Gray ND, White ML, Manning DAC. 2014. Comparison of silicate minerals as sources of potassium for plant nutrition in sandy soil. Euro J Soil Sci. 65:653–662.
- Morsomme P, Boutry M. 2000. The plant plasma membrane H+-ATPase: structure, function and regulation. Biochim Biophys Acta. 1465:1–16.
- Muscolo A, Bovalo F, Gionfriddo F, Nardi S. 1999. Earthworm humic matter produces auxin-like effects on Daucus carota cell growth and nitrate metabolism. Soil Biol Biochem. 31:1303–1311.
- Musculo A, Cutrupi S, Nardi P. 1998. IAA detection in humic substances. Soil Biol Biochem. 30(8-9):1199–1201.
- Nardi S, Concheri G, Pizzeghello D, Sturaro A, Rella R, Parvoli G. 2000. Soil organic matter mobilization by root exudates. Chemosphere. 41:653–658.
- Nardi S, Pizzeghello D, Ertani A. 2018. Hormone-like activity of the soil organic matter. Soil Biol Biochem. 30(8-9):1199–1201.
- Nardi S, Pizzeghello D, Muscolo A, Vianello A. 2002. Physiological effects of humic substances in higher plants. Soil Biol Biochem. 34:1527–1537.
- Norse D. 2005. Non-point pollution from crop production: global, regional and national issues. Pedosphere. 15:499–508.
- Piccolo A. 2002. The supramolecular structure of humic substances. A novel understanding of humus chemistry and implications in soil science. Adv Agron. 75:57–134. doi:10.1016/S0065-2113(02)75003-7.
- Pizzeghello D, Shiavon M, Francioso O, Dalla Vecchia F, Ertani A, Nardi S. 2020. Bioactivity of size-fractionated and unfractionated humic substances from two forest soils and comparative effects on N and S metabolism, nutrition, and root anatomy of Allium sativum L. Front Plant Sci. 11:1203.
- Plaza C, Senesi N, Polo A, Brunetti G. 2005. Acid−base properties of humic and fulvic acids formed during composting. Environ Sci Technol. 39:7141–7146.
- R Core Team. 2019. R: A language and environment for statistical computing. Vienna: R Foundation for Statistical Computing.
- Ramos AC, Dobbss LB, Santos LA, Fernandes MS, Olivares FL, Aguiar NO, Canellas LP. 2015. Humic matter elicits proton and calcium fluxes and signaling dependent on Ca2+-dependent protein kinase (CDPK) at early stages of lateral plant root development. Chem Biol Technol Agric. 2:3.
- Rosa MLS. 1999. Geologia, geocronologia, mineralogia e litogeoquímica do batólito monzo-sienítico Guanambi-Urandi. Salvador Bahia: Universidade Federal da Bahia; p. 186.
- Rosa SD, Silva CA, Maluf HJGM. 2018. Wheat nutrition and growth as affected by humic acid-phosphate interaction. J Plant Nutr Soil Sci. 181:870–877.
- Santos LF. 2016. Alterações de propriedades químicas do solo e nutrição do milho após adição de sienito nefelínico e ácidos húmicos. Brasilia: Universidade de Brasília, p. 118.
- Schnitzer M, Gupta UC. 1965. Determination of acidity in soil organic matter. Soil Sci Society Ame. 29(3):274–277.
- Silva DRG, Marchi G, Spehar CR, Guilherme LRG, Rein TA, Soares DA, Ávila FW. 2012. Characterization and nutrient release from silicate rocks and influence on chemical changes in soil. R. Bras Ci Solo. 36:951–962.
- Silva SHG, Poggere GC, Menezes MDD, Carvalho GS, Guilherme LRG, Curi N. 2016. Proximal Sensing and digital terrain models applied to digital soil mapping and modeling of Brazilian Latosols (Oxisols). Remote Sens (Basel). 8(8):614.
- Stevenson FJ. 1994. Humus chemistry. New Jersey: Wiley; p. 496.
- Swoboda P, Döring TF, Hamer M. 2022 Feb 10. Remineralizing soils? The agricultural usage of silicate rock powders: a review. Sci Total Environ. 807(Pt 3):150976. doi:10.1016/j.scitotenv.2021.150976.
- Tamfuh PA, Wotchoko P, Nono DGK, Ndofor CNY, Nkouathio DG, Bitom D. 2019. Comparative effects of basalt dust, NPK 20-10-10 and poultry manure on soil fertility and cucumber (Cucumis sativus) productivity in Bafut (Cameroon Volcanic Line). Earth Sciences. 8(6):323–334.
- Vaccaro S, Ertani A, Nebbioso A, Quaggiotti S, Piccolo A, Nardi S. 2015. Humic substances stimulate maize nitrogen assimilation and amino acid metabolism at physiological and molecular level. Chem Biol Technol Agric. 2:5.
- Van Straaten P. 2006. Farming with rocks and minerals: challenges and opportunities. An. Acad. Bras. Ciênc. 78(4):731–747.
- Verrillo M, Parisi M, Savy D, Caiazzo G, Di Caprio R, Luciano MA, Cacciapuoti S, Fabbrocini G, Piccolo A. 2022. Antiflammatory activity and potential dermatological applications of characterized humic acids from a lignite and a green compost. Sci Rep. 12:2152. doi:10.1038/s41598-022-06251-2.
- Vujinovic T, Zanin L, Venuti S, Contin M, Ceccon P, Tomasi N, Pinton R, Cesco S, De Nobili M. 2020. Biostimulant action of dissolved humic substances from a conventionally and an organically managed soil on nitrate acquisition in maize plants. Front Plant Sci. 10:1652. doi:10.3389/fpls.2019.01652.
- Wilson MA. 1987. NMR techniques and applications in geochemistry and soil chemistry. Oxford: Pergamon Press; p. 589.
- Zandonadi DB, Matos CRR, Castro RN, Spaccini R, Olivares FL, Canellas LP. 2019. Alkamides: a new class of plant growth regulators linked to humic acid bioactivity. Chem Biol Technol Agric. 6:23.
- Zanin L, Tomasi N, Cesco S, Varanini Z, Pinton R. 2019. Humic substances contribute to plant iron nutrition acting as chelators and biostimulants. Front Plant Sci. 10:675.
- Zhang S, Yuan L, Li Y, Zhao B. 2021. Characterization of ultrafiltration-fractionated humic acid derived from Chinese weathered coal by spectroscopic techniques. Agronomy. 11(10):2030. doi:10.3390/agronomy11102030.
- Zhang XM, Li Y, Hu C, He ZQ, Wen MX, Gai GS, Huang ZH, Yang YF, Hao XY, Li XY. 2019. Enhanced phosphorus release from phosphate rock activated with lignite by mechanical microcrystallization: effects of several typical grinding parameters. Sustainability. 11:1068. doi:10.3390/su11041068.