ABSTRACT
Phosphorus (P) losses from clay soils can be mitigated by introducing measures for improving soil structure. These include structure liming, where a mixture of CaO or Ca(OH)2 and CaCO3 is added to the soil. In a field experiment with separately tile-drained plots on a clay loam in Sweden, we examined the effects of structure liming on leaching of total-P, phosphate P (PO4-P) and total nitrogen (N) during three years after initial application. The treatments included two application rates (8 and 16 t ha−1) of a common product in comparison with a control (no lime). Effects of structure liming emerged during the second and third year after application, with 45 and 38% lower total-P leaching than in the unlimed control. A significant effect of the application rate was found in the third year. Nitrogen leaching and crop yield were not affected. As expected, soil pH raised following structure lime addition. Measurements of aggregate stability did not confirm the reduction in P leaching, indicating that it is important to measure P concentrations in drainage water directly when assessing the effect of structure liming.
Introduction
Soil health, soil quality and, more specifically, soil fertility are all concepts used to describe the capacity of soil to function as a vital living system and provide ecosystem services (Pankhurst et al. Citation1997). These aspects of soil are shaped by biological, physical and chemical processes. Soil structure, defined as the way in which soil particles are assembled into stable aggregates, is the main physical component of a healthy and productive soil (Bünemann et al. Citation2018). Good soil structure supports productivity, but also flood prevention, climate change mitigation, soil biodiversity and reduced water pollution (The Royal Society Citation2021). Nutrient loads from agricultural fields are one of the main contributors to the eutrophication of inland and coastal waters, e.g. in the Baltic Sea region (Brandt et al. Citation2009), where phosphorus (P) loads in particular need to be reduced (Boesch et al. Citation2008). Since 2001, mitigation programmes targeted at reducing P losses from agriculture have been implemented in many European Union (EU) countries, in compliance with the EU Water Framework Directive.
Poor or deteriorated soil structure results in altered water flow pathways, characterised by reduced infiltration capacity, lower water-holding capacity and increased surface runoff. Preferential flow in cracks and macropores is typical of clay soils, although it can also occur in other homogeneous soils (Jarvis et al. Citation2016), mediating rapid transport of solutes (Bergström and Jarvis Citation1993) and potentially also of particles. Losses of P from clay soils to tile drains have been observed to occur rapidly, e.g. after fertiliser application (Ulén et al. Citation2005; Aronsson et al. Citation2014). In Sweden, on average 50% of all P found in drainage water is in particulate form (Ulén and Jakobsson Citation2005). Surface runoff and erosion are other pathways for P losses, and are affected by risk factors such as precipitation, topography, soil type and soil infiltration (Djodjic and Villa Citation2015). In soils with good structure and stable soil aggregates, both surface runoff and preferential flow tend to be lower and instead more effective and uniform infiltration and percolation of water may occur, transporting smaller amounts of particles and dissolved P. The distribution pattern of infiltration and percolation in the soil matrix affects filtration of soil particles and adsorption of P moving with water through the soil. A soil with high P sorption capacity can act as a sink, reducing P transport to subsurface drains (Andersson et al. Citation2015). However, soil pH has a strong impact on P sorption in soil (Penn and Camberato Citation2019).
Programmes aiming to mitigate P losses include measures in the field and along ditches and streams. Measures for clay soils tend to focus particularly on reduced transport of particles carrying P. This involves measures for improving soil structure and aggregate stability, such as structure liming (Bergström et al. Citation2015). Structure liming refers to application of either quicklime (calcium oxide, CaO) or hydrated (slaked) lime (Ca(OH)2) to a clay soil, with the aim of initiating structure-improving processes. These processes include fast cation exchange causing flocculation and agglomeration, and slower pozzolanic reactions and lime carbonation (Blomquist Citation2021). For reasons related to health aspects during handling and spreading (calcium oxide and hydroxide are caustic), often only about 14% of the Ca applied as structure lime in Sweden is in ionic form (CaO or Ca(OH)2), i.e. directly active in structure-improving processes, while the rest is applied as CaCO3. Hereafter, the term ‘structure lime’ is used interchangeably for products with all Ca present as Ca2+ or in mixtures with CaCO3. Improvements in structure, aggregate stability and porosity following structure liming have been observed in field studies (Choquette et al. Citation1987; Blomquist et al. Citation2018; Bölscher et al. Citation2021). The effect of structure liming on particulate-P losses has been assessed indirectly, by measurements of soil aggregate stability, in several Swedish experiments (Blomquist et al. Citation2018). In those experiments, aggregates (2−5 mm) collected from the soil were exposed to artificial irrigation, following which turbidity in water effluent was measured in order to estimate the content of dispersed clay particles. This method assumes a positive correlation between turbidity and particulate-P in water (Etana et al. Citation2009), and thus does not consider losses of dissolved P from the aggregates. According to Blomquist (Citation2021), who summarised measurements at multiple sites in Sweden, there is a knowledge gap about best management practices for structure liming, including recommended doses for different soil clay contents, the effect of dose on duration of effects, and effects on micro-nutrient availability and crop yield. Despite this, and the fact that most findings are based on this type of indirect assessment of P losses, structure liming is a prioritised measure for reducing P losses from arable land in Sweden. Farmers are provided subsidies for structure liming from different programmes, and there is also a common interest among farmers due to possible positive effects on soil fertility and crop yields.
Only few studies have performed direct field measurements of both particulate-P and dissolved P leaching to subsurface drains after treatment with structure lime in Sweden (Svanbäck et al. Citation2014; Ulén and Etana Citation2014). Both these studies revealed less P leaching after structure liming, in Svanbäck et al. (Citation2014) 40% reduction of particulate-P and in Ulén and Etana (Citation2014), 50% reduction of P leaching in dissolved form. Observed effects of structure liming on P leaching thus can be due to higher stability of the aggregates, better filtration of particles and/or less leaching due to more efficient adsorption of soluble P (Eslamian et al. Citation2020).
The main objective of this study was to gain more knowledge about effects of structure liming on P leaching, but also to investigate simultaneous effects on N leaching and crop yields. In this case, a clay loam was studied, where two doses of structure lime, 8 t ha−1 (recommended by suppliers) and 16 t ha−1, were compared with a control with no structure liming. The first hypothesis tested was that structure liming decreases the concentration of total-P in drainage water leaving the field, and thereby leaching losses. This hypothesis was based on expected lower losses of P following improvements in soil structure resulting from increased soil aggregate stability. A second hypothesis tested was that N leaching is not affected by structure liming. A third hypothesis tested was that a higher dose of structure lime (16 t ha−1) gives a greater decrease in drain water P concentrations and leaching losses of total-P than the recommended dose (8 t ha−1).
Materials and methods
A field experiment was conducted at the Lilla Böslid research farm in south-west Sweden. The soil at the site is a clay loam, with specific soil properties as summarised in . Expandable minerals, mainly quartz, K-feldspar, plagioclase and di-mica, dominate the soil clay fraction. Mean annual temperature in the region is 8.4°C and mean annual precipitation is 850 mm (Halmstad 1991–2020, SMHI). The experimental field consisted of 18 separately tile-drained plots, each measuring 16 m × 20 m. There were two plastic drainage pipes in each plot at a spacing of 7 m and at a depth of 0.9 m.
Table 1. Characteristics of the clay loam soil at the experimental field.
Experimental set-up
In September 2018, 12 of the plots were treated with structure lime in two different doses, 8 and 16 tons ha−1, with six plots per treatment, where 8 t ha−1 is the dosage recommended by suppliers and advisors and 16 t ha−1 is double that dosage. The remaining six plots were control plots without lime application. All plots were distributed evenly and randomised across the field. The product used was Nordkalk Fostop Struktur, which contains both slaked (Ca(OH)2) and burned (CaO) lime mixed with limestone (CaCO3), in unknown proportions, but with a guide value of 20% active CaO and a neutralisation value of 58%. The water content of the product was 15−25% according to the supplier’s information.
Within one day after application, the structure lime was mixed into the soil to a depth of 15−18 cm with a cultivator driven several times over the field in different directions. After 10 weeks, the field was mouldboard ploughed to 18−20 cm depth.
Spring barley was grown in the field during the growing season of 2019 and 2020, while in September 2020 winter wheat was sown. These crops were managed according to local practices, e.g. the spring barley was fertilised with approximately 110 kg N ha−1 and 20 kg P ha−1 per year (NS 27-4, PK 11-21) and the soil was mouldboard ploughed in autumn 2019 and 2020. All plots were treated in the same way. Crop grain yield was determined at harvest, to identify any residual effects of structure liming. Grain yield and total-N and total-P content in grain were determined for three replicate crop samples (each from a sub-area of approximately 20 m2) per plot, using a LECO CNS-2000 analyser. Mean values of the three replicates from each plot were used in statistical analyses.
Sampling and analyses of drainage water
Sampling of drainage water started in September 2018 and continued for three years. As described in Norberg and Aronsson (Citation2020), drainage water from the separately tile-drained plots was conducted to an underground monitoring station with prevailing temperature between 10 and 15°C during the main drainage periods. Discharge rates were recorded using tipping buckets connected to a data logger, which stored accumulated daily drainage volumes from each plot. Flow-proportional water subsamples (15 mL per occasion) were taken using a peristaltic pump after every 0.2 mm discharge and collected in individual polyethylene bottles for each plot. The bottles were emptied every two weeks during the main drainage periods for analysis of total-P, phosphate P (PO4-P) and total-N. The total-P concentration was determined in unfiltered samples and the PO4-P concentration in filtered samples (0.2 µm), according to methods issued by the International Standard Organization (ISO 15681-12003), where all P is oxidised with K2S2O8 to PO4-P, which is analysed photometrically. The difference between total-P and PO4-P was assumed to represent particulate-P, although this fraction may also contain a small fraction of dissolved organic P (e.g. Liu et al. Citation2012). However, in runoff water from Swedish clay soils this fraction is normally small (Linefur et al. Citation2022). The total-N concentration was determined on unfiltered samples by a combustion catalytic oxidation method where all N was converted to nitrous oxide before analysis (Shimadzu TOC-VCPH + TNM-1), according to the European standard (SS-EN 12260-1).
The daily leaching loads of N and P were calculated by multiplying the concentration in each sample by the daily amount of drainage during the two-week period prior to the sampling date. Annual values of leaching were obtained by the accumulation of daily values during the period 1 September–31 August, which approximately corresponded to the time from application of the lime onwards in one-year periods. Mean monthly and annual concentrations were obtained by dividing the accumulated monthly and annual load by the monthly and annual amount of discharge from each plot.
Sampling and analyses of soil
Shortly after sowing in spring 2019 and 2020, soil samples were taken for chemical analysis and for aggregate stability tests. Samples of topsoil (0−20 cm) were analysed for pH, Ca content and electric conductivity (EC). Before analysis, the samples were air-dried and milled to <2 mm size. pH was determined in a soil:water solution of 1:5. Electric conductivity was determined on the same solution, but after filtration, using conductivity apparatus MU 6100 L and conductivity cell CO 11 (pHenomenal, VWR). The Ca concentration was determined with ammonium-lactate/acetic acid solution, filtered and analysed with ICP/OES (Avio200, Perkin Elmer, U.S.A.).
Aggregate stability testing, as described in Blomquist et al. (Citation2022), was performed on soil samples from the top layer (0−5 cm), which were sieved to obtain samples of the 2−5 mm aggregate fraction. The samples were air-dried and then subjected to simulated rainfall in two events, with 24 h between events. After 24 h, the leachate from the first rainfall event was shaken for 10 min, left to stand for 4.5 h, and then analysed for EC and turbidity. This procedure was repeated after the second rain event. In total, there were four values for each analysis and soil sample, one for each rain event and one for each year (2019 and 2020).
Statistical analysis
A mixed model was used to test for differences between treatments (control, liming at 8 and 16 tonnes ha−1) in yearly and monthly means (concentration and leaching of N and P, discharge) and differences between sampling occasions (yield, N and P concentrations in grain). Treatment was set as fixed factor, block as random factor and plot as repeated structure factor. When significant effects (p < .05) were found, least significant differences (Tukey HSD) were used to compare mean values. Monthly values were log-transformed before analysis, to meet normality requirements. The data were tested for normality using the Andersson–Darling test. Soil data did not meet the normality requirement, and therefore a Kruskal–Wallis test was used to test for differences between treatments. When significant effects (p < .05) were found, the Steel Dwass method was used to compare mean values. Relationships between variables were tested with a regression model (p < .05). All statistical analyses were carried out using JMP Pro 15 (SAS Institute, Inc.).
Results and discussion
Weather conditions and discharge
The monitoring period started on 1 September 2018, just before application of structure lime. Early summer 2018 was very warm and dry, and when precipitation started in August the dry soil profile was slowly rewetted, leading to discharge from mid-October onwards. Precipitation during the first year (1 September 2018–31 August 2019) was 701 mm and discharge was 335 mm. The following year (1 September 2019–31 August 2020) received a little more rain (738 mm), distributed especially during autumn and winter, which led to higher yearly discharge (566 mm). The third year (1 September 2020–31 August 2021) had lower precipitation (687 mm) and similar discharge as in the first year. Discharge was significantly lower from plots treated with 8 t ha−1 of structure lime than from unlimed control plots in the first year (), but not in the second or third year.
Table 2. Mean annual (1 September–31 August) drain discharge (mm), concentration (mg L-1) in drainage water, and leaching losses (kg ha-1) of total-P, phosphate-P (PO4-P) and total nitrogen (N) in treatments with 8 t lime ha−1 and 16 t lime ha−1 and an unlimed control during three years (2018/2019, 2019/2020, 2020/2021).
Leaching of N
Discharge and leaching losses of N are generally interconnected, with greater leaching losses associated with greater discharge, i.e. the amount of water that flows through the soil profile affects N losses. However, this relationship was not observed during the three years of monitoring at the study site, where leaching losses of N were greatest (82 kg ha−1 in the control) during the first year, with the lowest discharge. Nitrogen concentrations in drainage water were high in that year (up to 48 mg L−1; ), which resulted in considerable leaching losses despite moderate discharge. This was most likely due to high amounts of residual fertiliser N left in the soil after a summer with poor crop growth and low yields. However, the statistically significant difference in N leaching between treatments in the first year was most likely an effect of the significant differences in discharge observed between treatments, since there were no significant differences in N concentrations ().
Figure 1. Mean monthly sum of (A) drain discharge (mm) and precipitation (mm, squares); mean monthly leaching losses (kg ha−1) of (B) total phosphorus (P), (C) phosphate-P (PO4-P) and (D) total nitrogen (N); and mean monthly concentration (mg L−1) of (E) total-P, (F) PO4-P and (G) total-N in the three treatments (no lime – black bars and circles, 8 t lime ha−1 – grey bars and circles, 16 t lime ha−1 – white bars and circles) during the period September 2018–August 2021. Mean, standard deviation as error bars (n = 6). Months marked with * showed a significant difference between treatments (see also Supplementary Material).
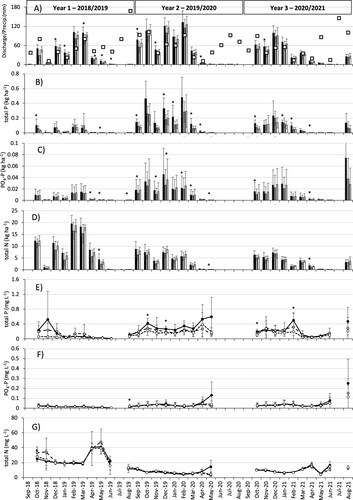
During the second and third years, leaching rate and concentration of N in drainage water were similar across the treatments, and thus structure liming did not affect the processes leading to N losses during those years ( and ).
Treatment effects on P leaching
Leaching losses of P were lowest during the first year (0.35 kg ha−1 in the control) and highest during the second year (1.9 kg ha−1 in the control). In a previous review, King et al. (Citation2015) concluded that a majority of P losses through subsurface drainage occur during periods of elevated flow. The findings in the present study are consistent with this, as the P concentration in drainage water and leaching losses of P were higher during the winter season in the second year, with higher discharge, than during the winter season in the first and third years (). King et al. (Citation2015) also concluded that the critical period for subsurface drainage discharge and P losses is during the non-growing season (late autumn, winter, early spring), which was also the case in the present study ().
The statistical analysis revealed higher leaching of total-P and PO4-P on a yearly basis from the unlimed control plots than from the plots treated with 8 or 16 t ha−1 structure lime during the second and third years, partly confirming our first hypothesis (). During the first year after application (1 September 2018–31 August 2019), no significant differences between treatments were found. During the second year (1 September 2019–31 August 2020) and third year (1 September 2020–31 August 2021), 8 t structure lime ha−1 reduced the P leaching losses by approximately 45% and 26%, respectively, compared with the control plots (). The higher load of structure lime (16 t ha−1) gave an even larger reduction during the third year, of approximately 38%. Furthermore, the P concentrations in drainage water were reduced in plots treated with structure lime, although the observed differences were not statistically significant (). In the third year, the higher load of structure lime (16 t ha−1) tended to reduce the concentration of P in drainage water by around 32%, while 8 t ha−1 did not show any reduction compared with the control plots, partly supporting our third hypothesis. This raises the question of whether the double dosage of structure lime (16 t ha−1) had a longer-lasting effect than the normal dosage on the soil at the study site. However, assessment of long-term effects of structure lime requires measurements for additional years. The lack of effect of liming during the first year was probably because the structure-improving processes in soil were slow and did not reach their full effect until after a full year. The differences between the treatments varied only slightly on a monthly basis, even though the yearly mean showed a significant difference ( and Supplementary Table S1 and ). shows how the leaching and concentrations varied across the monitoring period from September 2018 to August 2021. It can be seen from the diagram that the concentrations of N and P did not follow a similar trend. The fact that the concentration and load of N did not show any differences between treatments in the second and third year ( and ) confirms that the liming treatment had effect on P losses. Nitrogen leaching losses were not expected to be affected by the liming treatment, according to our second hypothesis.
Leaching losses of P from soils are known to display high variation, due to natural spatial variation at both large and small scale (Ulén et al. Citation2018). This was confirmed by our data, with high variation between plots within each treatment, but also between years ( and ). Mean annual P losses varied between 0.17 and 0.35 kg ha−1 during the first year and 1.1–1.9 kg ha−1 during the second year. In 2016, mean annual losses of P from arable land in Sweden (including soil erosion losses) were estimated to be 0.6 kg ha−1 (Johnsson et al. Citation2019). On a yearly basis, an average of around 17% of total-P in drainage water consists of PO4-P. Most of the remaining 83% of total-P can be assumed to consist of particulate-P, although this fraction might include a small fraction of dissolved organic P (e.g. Liu et al. Citation2012; Linefur et al. Citation2022).
In Sweden, only two previous field studies have measured actual P leaching losses from soils treated with structure lime, and both observed confirming results (Svanbäck et al. Citation2014; Ulén and Etana Citation2014). Svanbäck et al. (Citation2014) found that structure liming led to an approximately 40% reduction in total-P leaching losses from a clay soil, mainly brought about by a reduction in particulate-P losses, while Ulén and Etana (Citation2014) reported a 50% reduction in losses from a clay loam, mainly by a reduction in losses of PO4-P (there described as dissolved reactive phosphorus, DRP). In a Swedish lysimeter study, addition of lime to subsoil samples from two different clay soils reduced particulate-P leaching losses by around 50%, while PO4-P leaching was not affected (Andersson et al. Citation2016). In contrast, adding lime to subsoil samples from a sandy soil reduced PO4-P leaching, while particulate-P leaching was unaffected (Andersson et al. Citation2016). This is in line with findings by Eslamian et al. (Citation2020), in a study on liming of intact soil columns, showing a 40% and 70% decrease in PO4-P concentrations in leachate from sandy loam and loam, respectively, compared with an unlimed control. Eslamian et al. (Citation2020) attributed this reduction in losses to increased PO4-P adsorption on the soil surface after liming, due to two main mechanisms, Ca bridging and formation of calcium phosphate compounds. They also pointed out the importance of soil structure and they observed a positive correlation between Ca and P content in the soil. Their finding of a 40% reduction in PO4-P leaching from the coarser sandy loam soil indicates that calcium phosphates are concentrated in coarser fractions in the soil. Liming has been shown to improve phosphate adsorption in soil (Curtin and Syers Citation2001), and lime addition to drain trench backfill on a sandy loam in Lithuania was found to reduce PO4-P concentrations in drainage water (Povilaitis et al. Citation2018). These results indicate that structure liming affects P mitigation processes in different ways, depending on soil type. In soils with a high clay content, the main mechanism involves soil structure-forming processes capturing, preserving particulate-P, while in coarser-textured soils the main mechanism involves Ca2+ bridging, and formation of calcium phosphate.
All of these processes were possible in the present study, since the soil at the experimental site contains clay (29%), silt (43%) and sand (28%). Total-P and PO4-P losses were reduced by the structure liming in the second and third year of the study () and particulate-P (total-P minus PO4-P) in the second year (data not shown), which indicates that the reduction in losses of total-P was due to lower losses of both PO4-P and particulate-P.
Yield
Yield of spring barley increased slightly, but not significantly, in the two structure liming treatments during the first years after application, while the concentrations of N and P in grain were not affected by the treatments (). In an earlier study with four different field experiments, Blomquist et al. (Citation2018) observed varying yield response (no difference or decreases) of barley in limed plots compared with the control plots.
Aggregate stability, pH, EC and Ca2+ concentration in soil
Analysis of the topsoil samples taken in spring after application of lime showed that pH and content of Ca2+ had increased in plots with lime compared with control plots (). Additional Ca2+ ions in soil increase ionic strength and promote flocculation, which can rearrange soil particles and improve the formation and stability of aggregates. Despite this, there was no clear improvement in soil aggregate stability, measured as turbidity and EC in leachate from soil aggregates exposed to simulated rain events (). However, in the field there were visible signs of improved soil structure in the limed plots, for instance faster drying in spring and after heavy rain.
Table 3. pH, ammonium-lactate soluble calcium (Ca-AL, mg 100g−1), electric conductivity (EC, µS cm−1) in soil samples (0−20 cm depth), and turbidity (NTU) and electric conductivity (EC, µS cm−1) in soil aggregate stability samples in spring 2019 and 2020.
In the studies by Blomquist et al. (Citation2018), Blomquist and Berglund (Citation2021) and Blomquist et al. (Citation2022), soil aggregate stability was used as a proxy for the effect of structure liming in Swedish arable soils, particularly in the form of mitigation of P losses from clay soils. Blomquist et al. (Citation2022) identified four soil characteristics that determine the effect of structure liming on soil aggregate stability, namely clay content, initial soil pH, soil organic matter (SOM) content and soil mineral index (SmV). Positive results of liming were expected at our study site, since it met the requirements for three of these four factors: Clay content (29%) fell into the group of medium clay soils (25−40%), initial soil pH (6.6) fell into the medium group (6.6−7.7), and soil organic matter (SOM) content (3.2%) was in the group of moderate SOM (3−6%), all of which resulted in 8 or 16 t ha−1 of added structure lime improving soil aggregate stability in the study by Blomquist et al. (Citation2022). Regarding SmV, our study soil fitted into the group with expandable clay minerals, which was characterised by Blomquist et al. (Citation2022) as a soil type with no effect of structure liming, although the statistics were not totally clear for that group. In their study, non-expandable clay minerals, e.g. illite, kaolinite, chlorite, were found to be more affected by structure lime than expandable clay minerals, e.g. smectite and vermiculite (Blomquist et al. Citation2022). In an earlier greenhouse pot experiment by Keiblinger et al. (Citation2016), clay content and cation exchange capacity were found to be the most important soil characteristics determining the effect of quicklime on soil aggregate stability, probably due to the flocculating effect of added Ca2+. In that study, the effect on soil aggregate stability after application of quicklime was instant (within two days) and persisted for a monitoring period of three months in three soils with medium clay content (27−40%).
Blomquist and Berglund (Citation2021) found that the timing and conditions at application of structure lime affect the outcome, with an early application time (mid-August) giving better results on soil aggregate stability one year after treatment than the normally recommended application time (mid-September). However, three years after treatment in that study there were no differences between the two application dates, and unfavourable soil moisture (too wet) and rain soon after application of the structure lime reduced the effect on soil structure (Blomquist and Berglund Citation2021).
In the present study, we applied the structure lime in mid-September, in warm, dry conditions. We incorporated the lime with a tine cultivator, as is recommended practice, and no heavy rain fell in the period soon after application, but despite these favourable conditions no effect of structure liming on soil aggregate stability was detected during spring in the two following years. However, while there was no measurable effect on soil aggregate stability, P leaching losses were reduced during two of the three years of measurements (). This suggests that structure lime affects soil P processes in other ways than those measurable as soil structure improvements or those structure improvements were too small to be detectable with the method used. Leaching of total-P and also of PO4-P was reduced, indicating the involvement of other processes such as increased adsorption of dissolved P in the soil matrix, possibly due to increased soil pH (Penn and Camberato Citation2019), as suggested in other studies (Ulén and Etana Citation2014; Andersson et al. Citation2016; Povilaitis et al. Citation2018; Eslamian et al. Citation2020).
Recommendations
This three-year study on the effects of structure liming on a clay loam revealed that P leaching losses were reduced during the second and third year after structure liming, by 45% and 38%, respectively. This justifies current Swedish recommendations on prioritising structure liming as a measure for mitigating P leaching losses from clay soils.
It could not be confirmed that a higher rate of structure lime (16 t ha−1) gave improved effect on P leaching compared with the recommended rate (8 t ha−1), as an effect was seen only in the third year after application. It is important to investigate the persistence of effects over time more thoroughly, including effects of different application rates, in order to identify long-term effects of structure liming.
The results obtained in this study show the importance of direct measurements of P concentrations in drainage water in order to assess the true effect of structure liming. The positive effect seen in this study in terms of reduced P concentrations in drainage water was not reflected in improved aggregate stability, which indicates that this is not an accurate indicator of liming effects, with the method used here.
The observed reduction in total-P leaching included reduced leaching of both particulate-P and PO4-P. Thus, different mechanisms were involved, and not only those related to pathways for particulate-P losses. In Sweden, structure liming is recommended for mitigation of P losses from clay soils with poor structure. Our results suggest that the addition of Ca2+ ions by liming may not only reduce P leaching from clay soils, but also from soils with a more coarser texture where there is a risk of losses of dissolved P.
Supplemental Material
Download MS Word (64.6 KB)Acknowledgements
We wish to thank the field staff at Hushållningssällskapet Halland, Maria Blomberg for technical assistance, the water laboratory at the Department of Aquatic Sciences and Assessment and the Soil and Crop Laboratory at the Department of Soil and Environment, SLU.
Disclosure statement
No potential conflict of interest was reported by the author(s).
Additional information
Funding
Notes on contributors
Lisbet Norberg
Lisbet Norberg (PhD) is a specialist in soil science, with the focus on long-term monitoring of water quality in arable field runoff. Her research deals with the impact of agricultural management practice, soil type and climate on nutrient leaching in the agricultural landscape.
Helena Aronsson
Helena Aronsson (Associate Professor) is a senior lecturer in nutrient management with special commitment to extension duties. Her research centres on management practices for reduced nutrient leaching from arable land.
References
- Andersson H, Bergström L, Djodjic F, Ulén B, Kirchmann H. 2016. Lime placement on subsoil as a strategy to reduce phosphorus leaching from agricultural soils. Soil Use Manag. 32:381–389.
- Andersson H, Bergström L, Ulén B, Djodjic F, Kirchmann H. 2015. The role of subsoil as a source or sink for phosphorus leaching. J Environ Qual. 44:535–544.
- Aronsson H, Liu J, Ekre E, Torstensson G, Salomon E. 2014. Effects of pig and dairy slurry application on N and P leaching from crop rotations with spring cereals and forage leys. Nutr Cycling Agroecosyst. 98:281–293.
- Bergström LF, Jarvis NJ. 1993. Leaching of Dichlorprop, Bentazon, and Cl-36 in undisturbed field lysimeters of different agricultural soils. Weed Sci. 41:251–261.
- Bergström L, Kirchmann H, Djodjic F, Kyllmar K, Ulén B, Liu J, Andersson H, Aronsson H, Börjesson G, Kynkaanniemi P, et al. 2015. Turnover and losses of phosphorus in Swedish agricultural soils: long-term changes, leaching trends, and mitigation measures. J Environ Qual. 44:512–523.
- Blomquist J. 2021. Effects of structure liming on clay soil. Acta Universitatis Agriculturae Sueciae [Doctoral Thesis no. 2021:86].
- Blomquist J, Englund JE, Berglund K. 2022. Soil characteristics and tillage can predict the effect of ‘structure lime’ on soil aggregate stability. Soil Res. 60:373–384.
- Blomquist JE, Berglund K. 2021. Timing and conditions modify the effect of structure liming on clay soil. Agri Food Sci. 30:96–107.
- Blomquist J, Simonsson M, Etana A, Berglund K. 2018. Structure liming enhances aggregate stability and gives varying crop responses on clayey soils. Acta Agri Scand Sec B-Soil Plant Sci. 68:311–322.
- Boesch D, Carstensen J, Paerl HW, Skjodal HR, Voss H. 2008. Eutrophication of the seas along Sweden’s West Coast. Report 5898. Stockholm: Swedish Environmental Protection Agency.
- Bölscher T, Koestel J, Etana A, Ulén B, Berglund K, Larsbo M. 2021. Changes in pore networks and readily dispersible soil following structure liming of clay soils. Geoderma. 390:114948.
- Brandt M, Ejhed H, Rapp L. 2009. Nutrient loads to the Swedish marine environment in 2006. Report 5995. Stockholm: Swedish Environmental Protection Agency.
- Bünemann EK, Bongiorno G, Bai ZG, Creamer RE, De Deyn G, De Goede R, Fleskens L, Geissen V, Kuyper TW, Mader P, et al. 2018. Soil quality – a critical review. Soil Biol Biochem. 120:105–125.
- Choquette M, Bérubé M-A, Locat J. 1987. Mineralogical and microtextural changes associated with lime stabilization of marine clays from eastern Canada. Appl Clay Sci. 2:215–232.
- Curtin D, Syers JK. 2001. Lime-induced changes in indices of soil phosphate availability. Soil Sci Soc Am J. 65:147–152.
- Djodjic F, Villa A. 2015. Distributed, high-resolution modelling of critical source areas for erosion and phosphorus losses. Ambio. 44:S241–S251.
- Eslamian F, Qi Z, Tate MJ, Romaniuk N. 2020. Lime application to reduce phosphorus release in different textured intact and small repacked soil columns. J Soils Sediments. 20:2053–2066.
- Etana A, Rydberg T, Arvidsson J. 2009. Readily dispersible clay and particle transport in five Swedish soils under long-term shallow tillage and mouldboard ploughing. Soil Tillage Res. 106:79–84.
- Jarvis N, Koestel J, Larsbo M. 2016. Understanding preferential flow in the vadose zone: recent advances and future prospects. Vadose Zone J. 15:1–11.
- Johnsson H, Mårtensson K, Lindsjö A, Persson K, Andrist Rangel Y, Blombäck K. 2019. Läckage av näringsämnen från svensk åkermark. SMED Rapport nr 5 (In Swedish).
- Keiblinger KM, Bauer LM, Deltedesco E, Holawe F, Unterfrauner H, Zehetner F, Peticzka R. 2016. Quicklime application instantly increases soil aggregate stability. Int Agrophys. 30:123–128.
- King KW, Williams MR, Macrae ML, Fausey NR, Frankenberger J, Smith DR, Kleinman PJA, Brown LC. 2015. Phosphorus transport in agricultural subsurface drainage: a review. J Environ Qual. 44:467–485.
- Linefur H, Norberg L, Kyllmar K, Andersson S, Blomberg M. 2022. Växtnäringsförluster i små jordbruksdominerande avrinningsområden 2020/2021. Ekohydrologi. 175 (In swedish).
- Liu J, Aronsson H, Bergstrom L, Sharpley A. 2012. Phosphorus leaching from loamy sand and clay loam topsoils after application of pig slurry. Springerplus. 1:1–10.
- Norberg L, Aronsson H. 2020. Effects of cover crops sown in autumn on N and P leaching. Soil Use Manag. 36:200–211.
- Pankhurst CE, Doube BM, Gupta VVSR. 1997. Biological indicators of soil health: synthesis. In: Pankhurst C. E., Doube B.M., Gupta V.V.S.R., editor. Biological indicators of soil health. Wallingford: CAB International; p. 419–435.
- Penn CJ, Camberato JJ. 2019. A critical review on soil chemical processes that control how soil pH affects phosphorus availability to plants. Agriculture. 9:120.
- Povilaitis A, Rudzianskaite A, Miseviciene S, Gasiunas V, Miseckaite O, Živatkauskiene I. 2018. Efficiency of drainage practices for improving water quality in Lithuania. Trans ASABE. 61:179–196.
- Svanbäck A, Ulén B, Etana A. 2014. Mitigation of phosphorus leaching losses via subsurface drains from a cracking marine clay soil. Agric Ecosyst Environ. 184:124–134.
- The Royal Society. 2021. Soil structure and its benefits. [Accessed 2021 Sep 27]. http://www.royalsociety.org/soil-structure-benefits [Online].
- Ulén B, Aronsson H, Torstensson G, Mattsson L. 2005. Phosphorus and nitrogen turnover and risk of waterborne phosphorus emissions in crop rotations on a clay soil in southwest Sweden. Soil Use Manag. 21:221–230.
- Ulén B, Etana A. 2014. Phosphorus leaching from clay soils can be counteracted by structure liming. Acta Agri Scand Sec B-Soil Plant Sci. 64:425–433.
- Ulén B, Jakobsson C. 2005. Critical evaluation of measures to mitigate phosphorus losses from agricultural land to surface waters in Sweden. Sci Total Environ. 344:37–50.
- Ulén B, Larsbo M, Koestel J, Hellner Q, Blomberg M, Geranmayeh P. 2018. Assessing strategies to mitigate phosphorus leaching from drained clay soils. Ambio. 47:114–123.