Abstract
Magnesium and calcium ions are important factors in making tofu. However, the molecular role of these ions remains unclear in tofu formation. We have previously shown that magnesium chloride concentration-dependent produced silken tofu-like (SP) and regular tofu-like (RP) precipitates, but was an inconsequential factor for the retention of tofu. We investigated in this present study, the effect of various metal chlorides on the metal chloride concentration-dependent changes in tofu formation. These changes occurred in a similar manner to that of the magnesium ion, in which SP formation was followed by RP formation. It is interesting that the midpoint concentration for the formation of SP and RP represented a good correlation with the stability constant of EDTA. This correlation demonstrated the possibility that metal ions would interact with the carboxyl groups of soy proteins. We consider from these results that metal ions were the initiators of protein association in tofu formation.
Tofu is roughly classified into silken tofu (kinugoshi-dofu) and regular tofu (momen-dofu). Silken tofu was developed in the Edo period, and therefore has a shorter history than regular tofu which is the fundamental type.Citation1) Regular tofu is visibly different from silken tofu by its appearance and also has a lower water content than silken tofu.Citation2) These different properties imply that regular tofu is different from silken tofu. We have shown in our previous study that silken tofu and regular tofu were produced by different processes.Citation3) The selection of these different processes was determined by the concentration of magnesium chloride.Citation3) However, the mechanism for the destination and role of magnesium chloride in tofu formation has remained unclear.
The process of making tofu has long been the subject of study by the food industry. However, details of this process remain to be elucidated at the molecular level. The addition of a coagulant to soymilk heated to a suitable temperature to make the soy proteins coagulateCitation4) with magnesium chloride, magnesium sulfate, calcium chloride, calcium sulfate, and glucono-δ-lactone is a well-known manipulation.Citation5) Although glucono-δ-lactone is only used to make silken tofu, the other coagulants are used to make both silken tofu and regular tofu.Citation5) Previous studies have shown the coagulant concentration to be an important factor in the process of making tofu.Citation3,Citation6–Citation9) We have previously shown that the concentration of magnesium chloride was an important factor to selectively separate silken tofu and regular tofu.Citation3) When tofu is produced by adding a divalent cation such as magnesium or calcium ions, the divalent cations act as a linker between soy proteins through the carboxyl groups on the proteins. However, it is a plausible assumption that divalent cations act as a linker. Although some calcium ions bind with soy proteins,Citation6,Citation10–Citation12) the role of metal ions as the linker remains to be confirmed. We have also previously shown that magnesium ion was an inconsequential factor for the retention of tofu.Citation3)
To examine the role of metal ions in the formation of silken tofu and regular tofu, the effects of various metal ions on concentration-dependent tofu formation were investigated here by the addition of calcium, ferrous, copper, manganese, and ferric chlorides.
Materials and methods
Materials
Plain soymilk (50 mg of protein/mL) was purchased from Meiraku (Nagoya, Japan). This soymilk was prepared according to the traditional method described by Nito.Citation2) The protein assay dye reagent was purchased from Bio-Rad Laboratories (CA, USA). Other reagents were purchased from Wako Pure Chemicals (Osaka, Japan) and Nacalai Tesque (Kyoto, Japan).
Preparation of the tofu-like precipitate and supernatant from soymilk
Soymilk was incubated at 80 °C for 10 min.Citation4,Citation9) Metal chlorides were dissolved in distilled water at various concentrations. A 0.11 volume of each metal chloride solution was added to the incubated soymilk at various concentrations while mixing well, and was then held at 80 °C for 60 min.Citation4,Citation9) The mixture was incubated on ice for 15 min, and then separated into a supernatant and precipitate by centrifugation at 4,100 × g for 10 min at 4 °C.
Precipitate efficiency
The wet precipitate weight (WPW) was measured with an HR-120 electronic balance (A&D Company, Tokyo, Japan). The precipitate efficiency and ratio of the WPW were expressed as the percentage of the weight of the wet precipitate to that of the initial soymilk. Precipitate efficiency and WPW are synonymous terms. Data represent the average of three independent experiments.
Protein concentration
Supernatant proteins were quantified by the method of Bradford with the protein assay dye reagent (Bio-Rad Laboratories, CA, USA). The ratio of protein concentrations was expressed as the percentage of the protein concentration of the supernatant resulting from the addition of metal chlorides to the protein concentration of the supernatant prepared by the addition of distilled water. Data represent the average of three independent experiments.
Residual water content
The weight of initial soymilk was measured. The precipitate was prepared as just described. After measuring the WPW, the wet precipitate was dried at 105 °C for 3 h, until a constant weight was reached. The dry precipitate weight was measured with the same balance. The weight of water in the precipitate was calculated by subtracting the dry weight from the wet weight. The residual water content (RWC) is expressed as the percentage of the water weight contained in the wet precipitate to the initial weight of the soymilk. Data represent the average of three independent experiments.
Results and discussion
Formation of the tofu-like precipitate by the addition of various metal chlorides
To make tofu, such divalent metal salts as magnesium chloride, calcium chloride, magnesium sulfate, and calcium sulfate salts have been used as the coagulant.Citation5) It is well known that divalent metal salts make the soy proteins precipitate during tofu formation.Citation6–Citation8,Citation10–Citation15) In addition to calcium and magnesium salts, the zinc salt has also been shown to interact with soy proteins, leading to the generation of a protein precipitate.Citation13,Citation14,Citation16) We therefore investigated the precipitate formed by the addition of other metal salts, including copper chloride, ferrous chloride, ferric chloride, and manganese chloride. Zinc chloride was not utilized as a coagulant, because it does not sufficiently dissolve in water. Each metal chloride was added to heated soymilk, and the mixture was then separated into a supernatant and precipitate as already described above previously reported.Citation3) Fig. shows that a tofu-like precipitate was generated by the addition of the metal chlorides. It is interesting that, although ferric ion is a trivalent cation, its addition also produced a tofu-like precipitate (Fig. (A)). This is the first report to show that trivalent cations can also form a soy protein precipitate and can be utilized as a coagulant to make tofu-like food. The precipitate generated by the addition of divalent metal chlorides had a similar ratio for the wet weight to the precipitate generated by the addition of magnesium and calcium chlorides (Fig. (B)). Metal chlorides were added to soymilk at a final concentration of 20 mM which is almost same as that used to make regular tofu with magnesium chloride by the food industry.Citation1) These results indicate the possibility that metal chlorides can also be used as a coagulant to make tofu-like food.
Fig. 1. Formation of a tofu-like precipitate by the addition of various metal chlorides.
Note: Various metal chlorides, magnesium, calcium, copper, ferrous, ferric, and manganese chlorides, were added to soymilk to a final concentration of 20 mM. The mixture was separated into a supernatant and precipitate by centrifugation at 4,100 × g for 10 min at 4 °C. (A) Separation was observed. (B) The precipitation efficiency is expressed as the percentage weight of the wet precipitate to that of the initial soymilk. Data are shown as the average ± standard deviation of five independent experiments.
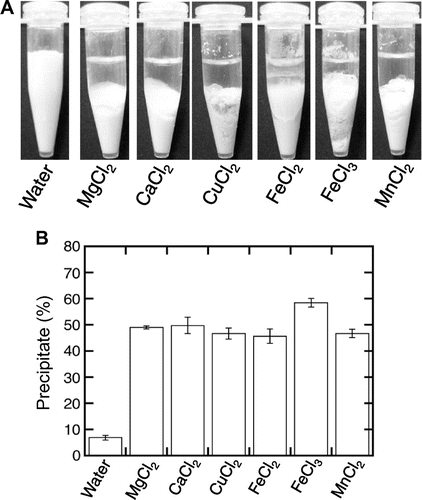
Metal chloride concentration-dependent changes in the tofu-like precipitate
To investigate the metal chloride concentration-dependent changes in the wet precipitate, various metal chlorides were added to soymilk at respective concentrations ranging from 0 to 20 mM (Fig. ). The mixture was separated into a precipitate and supernatant. The wet weight of the precipitate was investigated with the various metal chlorides (Fig. , open circles). In addition, the protein concentration in the supernatant was determined at various metal chloride concentrations (Fig. , closed circles). As shown in our previous study,Citation3) the changes in wet weight with the addition of magnesium chloride were approximately 60% higher at a lower concentration, and then decreased by approximately 50% at a higher concentration, following a short plateau phase (Fig. (A), open circles). Furthermore, the protein concentration of the supernatant was markedly decreased by the addition of magnesium chloride at a lower concentration and was not increased at the higher concentration (Fig. (A), closed circles). Similar responses were observed with the addition of calcium chloride (Fig. (B), open circles), which has been used as a coagulant by the food industry,Citation5) whereas both ferrous chloride (Fig. (D)) and manganese chloride (Fig. (F)) are not used to make tofu by the food industry. The addition of copper chloride (Fig. (C)) and ferric chloride (Fig. (E)), which are not also used to make tofu by the food industry, led to a peak instead of the short plateau phase, with a sharp increase and slight decrease, respectively, occurring in a similar manner at both lower and higher concentrations. Changes in the supernatant protein concentration also occurred in a similar manner according to the increase in metal chloride concentrations (Fig. , closed circles). Furthermore, when the wet weight was decreased at the higher concentration of each metal chloride, the protein concentration was not increased. As described in our previous study, a decrease in the wet weight was apparent with different solubilization of the precipitate.Citation3) These results show that the various metal chlorides each produced a tofu-like precipitate in a similar manner to that reported in our previous study, and support the possibility that we could selectively separate the production of food into a silken tofu-like food and a regular tofu-like food.
Fig. 2. Metal chloride concentration-dependent changes in the precipitate formation and supernatant protein concentration.
Note: Magnesium chloride (A), calcium chloride (B), copper chloride (C), ferrous chloride (D), ferric chloride (E), and manganese chloride (F) were added to soymilk at various concentrations. The mixture was separated into a supernatant and precipitate as described in the Materials and methods section. The precipitation efficiency (open circles) and supernatant protein concentration (closed circles) were determined as described in the Materials and methods section. Data are shown as the average ± standard deviation of three independent experiments.
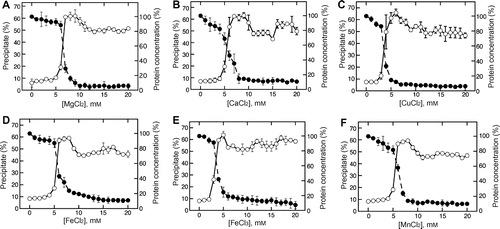
Physical appearance and water content of the tofu-like precipitate
We have demonstrated in our previous study that the physical appearance and water content of the tofu-like precipitate were destined by the concentration of magnesium chloride added.Citation3) To observe the appearance of the precipitate generated by adding other metal chlorides, a precipitate was prepared by adding each metal chloride at a lower and higher concentration to an equal volume of soymilk (Fig. ). The concentrations added were selected from the results of Fig. . The appearance of the precipitate generated by each metal chloride at the lower concentration (Fig. (B), (D), (F), (H), (J), and (L)) had a comparatively smoother surface and more compact shape than that generated at the higher concentration (Fig. (C), (E), (G), (I), (K), and (M)).
Fig. 3. Aspects of the precipitate resulting from the addition of a metal chloride.
Note: The precipitates were prepared from an equal volume (0.9 mL) of soymilk (A) as already described. Magnesium chloride was added at 9.0 mM (B) and 12.0 mM (C). Calcium chloride was added at 8.0 mM (D) and 13.0 mM (E). Copper chloride was added at 6.0 mM (F) and 12.0 mM (G). Ferrous chloride was added at 7.0 mM (H) and 12.0 mM (I). Ferric chloride was added at 5.0 mM (J) and 8.0 mM (K). Manganese chloride was added at 7.0 mM (L) and 13.0 mM (M). White bars show 1 cm.
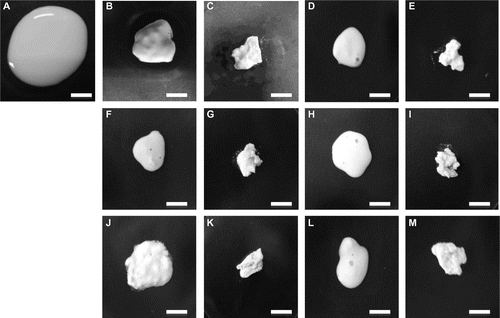
As has been previously described,Citation3) the precipitate yielded with the addition of magnesium chloride at the lower concentration exhibited physical properties similar to silken tofu. In contrast, the precipitate yielded at the higher concentration had physical properties similar to regular tofu. The silken tofu-like precipitate (SP) had higher water content than that of the regular tofu-like precipitate (RP). We therefore analyzed the ratio of the WPW and RWC of the precipitate prepared by adding various metal chlorides at the lower and higher concentrations (Table ). Differences in these values between SP and RP (Δ value) were calculated by subtracting the value of RP from that of SP. Table shows the Δ value of WPW to be similar to that of RWC with each metal chloride. These results strongly indicate the possibility that the tofu-like precipitates prepared with each metal chloride were produced by a similar mechanism and that these multivalent cations could also be utilized to make silken tofu-like and regular tofu-like foods.
Table 1. Differences in the % precipitate and % RWC between SP and RP.
Effect of metal ions on the SP formation
Proteins are soluble and partially denatured with the addition of magnesium chloride at low concentrations, and comparatively more proteins approach each other through salt bridges.Citation3) To investigate the correlation between protein precipitation and the changes in WPW with SP formation, we estimated the midpoint concentration (Cm) of the coagulant for protein reduction in the supernatant and the change in WPW with SP formation (Table ). Cm values were determined from the plots for SP formation in Fig. by a fitting analysis with a sigmoidal function using KaleidaGraph 3.6 software (Synergy Software, PA, USA). For curve fitting to determine the Cm values for the changes in WPW with SP formation, we used the data from 0 to 9 mM in MgCl2 (r2 = 0.999), from 0 to 9 mM in CaCl2 (r2 = 0.999), from 0 to 6 mM in CuCl2 (r2 = 0.998), from 0 to 8 mM in FeCl2 (r2 = 0.999), from 0 to 5 mM in FeCl3 (r2 = 0.999), and from 0 to 8 mM in MnCl2 (r2 = 0.999). The results showed that the Cm value of WPW with each metal chloride was similar to the Cm value for the reduction in protein concentration. This result implies that SP formation was correlated with the precipitation of soymilk proteins.
Table 2. Midpoint concentrations for changes in the WPW and protein concentration, and the stability constants for the EDTA-metal complexes.
The interaction between divalent cations and the carboxyl groups of soy proteins has been recognized as important when making tofu. Sakakibara and NoguchiCitation11) have shown that the calcium ion bound to the carboxyl group of the 11S protein, which is the major protein in soy proteins, in the neutral pH region (pH 6.0–8.0). However, this evidence was inadequate to explain the interaction of cations with carboxyl groups in tofu formation. To reinforce this theory, the correlation for the interaction between carboxyl groups and multivalent cations was investigated for SP formation. Since metal ions can bind to proteins through the carboxyl groups of the glutamyl and asparagyl residues, we analyzed the correlation between the midpoints and the stability constants of EDTA (log KEDTA) for the different metal ions. EDTA is one of the most popular chelators and has four carboxyl groups in its molecules. Table summarizes the respective stability constants of EDTA for Ca2+, Cu2+, Fe2+, Fe3+, Mg2+, and Mn2+ as 10.70, 18.8, 14.3, 25.7, 8.69, and 13.56.Citation17) The stability constant reflects the degree of interaction between the chelator and the metal ion.Citation17) Fig. (A) shows the value for the stability constant of EDTA for each metal ion plotted against the Cm value for the change in WPW (closed circles) and the Cm value for the reduction in protein concentration of the supernatant (open triangles). Plots for the change in WPW (solid line) and the reduction in protein concentration of the supernatant (dashed line) were fitted by an exponential function using the same software. Interestingly, the stability constants represented the correlation with both the Cm value for the change in WPW (r2 = 0.949) and the reduction in protein concentration of the supernatant (r2 = 0.839). The results showed that these changes were highly correlated with the interaction between the metal ion and carboxyl group. Furthermore, the fitting curve for the Cm value for the change in WPW almost overlapped that for the reduction in protein concentration of the supernatant. This overlap strongly suggests that the interaction between the metal ions and the carboxyl groups of soy proteins was an important factor for SP formation. However, the magnesium ion was not found to be an important factor for the retention of SP in our previous study.Citation3) These findings indicate that multivalent cations played the role of initiator for SP formation.
Fig. 4. Correlation between the changes in WPW and protein precipitation with the stability constants of EDTA for the metal ions.
Note: The respective stability constants of EDTA (log KEDTA) for Ca2+, Cu2+, Fe2+, Fe3+, Mg2+, and Mn2+ were 10.70, 18.8, 14.3, 25.7, 8.69, and 13.56.Citation17) A, The Cm value for the transition of WPW (closed circles) and protein concentration reduction (open triangles) with SP formation were calculated from Fig. by a sigmoidal function, using KaleidaGraph 3.6 software (Synergy Software, PA, USA). Plots for the precipitate efficiency (solid line) and protein concentration reduction (dashed line) were fitted by an exponential function with the same software. B, The Cm value for the decrease in WPW with RP formation (open circles) was calculated from Fig. by a sigmoidal function with, KaleidaGraph 3.6 software. The plots were fitted by a linear function with the same software (solid line).
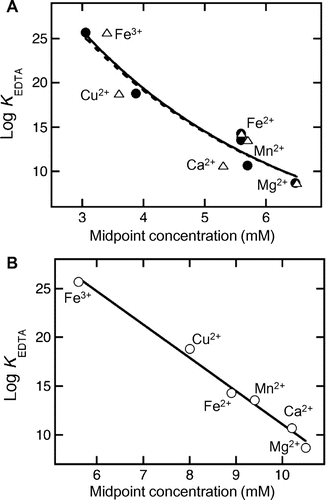
Effect of metal chlorides on formation of the RP
Our previous studyCitation3) has shown hydrophobic interactions to be important for the retention of RP in RP formation. In contrast, the interaction through the magnesium ion was not important for the retention of RP. Therefore, the role of metal ions in RP formation remains unclear. To investigate the correlation between the Cm value for the small decrease in WPW with RP formation and the stability constant for EDTA, the Cm value was estimated from the plot for RP formation in Fig. by a curve-fitting analysis with a sigmoidal function using the same software. For the curve-fitting analysis to determine the Cm value for RP formation, we used the data from 7 to 16 mM in MgCl2 (r2 = 0.922), from 7 to 14 mM in CaCl2 (r2 = 0.976), from 5 to 20 mM in CuCl2 (r2 = 0.840), from 6 to 14 mM in FeCl2 (r2 = 0.956), from 4 to 11 mM in FeCl3 (r2 = 0.945), and from 6 to 20 mM in MnCl2 (r2 = 0.970). The value for each metal ion is summarized in Table and was plotted against the stability constant for EDTA (Fig. (B)). The plot was fitted by a linear function using the same software (Fig. (B)). It is interesting that the stability constants showed very good correlation with the Cm value for the decrease in WPW with RP formation (r2 = 0.990). These results show that, although the interaction between the metal ions and carboxyl groups of soy proteins was not important for the retention of RP, this interaction played a role in RP formation. This means that metal ions also acted as the initiators of protein association with RP formation.
Our previous reportCitation3) has shown that the solubility of both SP and RP in EDTA was similar to that in water alone. EDTA had no effect on the solubilization of proteins in these precipitates, implying that the metal ion was not important for the retention of these precipitates. We make clear in the present study that the Cm values for both SP and RP formation presented very good correlation with the stability constants of EDTA for various metal ions. This evidence shows that the interaction between metal ions and the carboxyl groups of soy proteins played an important role in tofu formation, but not in the retention of tofu. In conclusion, magnesium and calcium ions act as the initiators of protein association in tofu formation. However, although the final result was determined by the differing degrees of protein denaturation, it remains unclear why the concentration of the coagulant selectively separated tofu formation into silken tofu and regular tofu. These results also show that mineral-fortified tofu-like foods may be produced by using these metal chlorides and may be utilized for improving nutrition and disease prevention.
Acknowledgments
This work was financially supported by Grant-in-Aid for Young Scientists (B) from the Ministry of Education, Culture, Sports, Science and Technology of Japan [23700894]. We also thank M. Okamura, E. Torii, A. Murakami, and H. Yasuda for their technical assistance.
Notes
Abbreviations: Cm, midpoint concentration; RP, regular tofu-like precipitate; RWC, residual water content; SP, silken tofu-like precipitate; WPW, wet precipitate weight.
References
- Nito H. Tofu. Tokyo: Rural Culture Association Japan; 2004: p. 28–29.
- Resources Council for Science and Technology, Ministry of Education, Culture, Sports, Science, and Technology, Japan. Standard tables of food composition in Japan. 5th revised and enlarged ed.; 2005.
- Arii Y, Takenaka Y. Magnesium chloride concentration-dependent formation of tofu-like precipitates with different physicochemical properties. Biosci. Biotechnol. Biochem. 2013;77:928–933.
- Watanabe T, Saio K. Tofu wo Kangaeru. Kagaku To Seibutsu (in Japanese). 1973;11:631–640.
- Taira H, Kijima H, Abe N. Proximate and mineral compositions of four types of tofu with different coagulants. Foods Food Ingredients J. Jpn. (in Japanese). 2000;188:49–56.
- Tamura S. Tofu nitaisuru Karusiumu no Sayo. Kogyokagaku Zasshi (in Japanese). 1959;62:1877–1880.
- Tamura S. Tonyu nitaisuru Karusiumu no Ketsugo to Gyoseki tono Kankei. Kogyokagaku Zasshi (in Japanese). 1959;62:1880–1883.
- Ono T, Katho S, Mothizuki K. Influences of Calcium and pH on protein solubility in soybean milk. Biosci. Biotechnol. Biochem. 1993;57:24–28.
- Toda K, Ono T, Kitamura K, Hajika M, Takahashi K, Nakamura Y. Seed protein content and consistency of tofu prepared with different magnesium chloride concentrations in six Japanese soybean varieties. Breed. Sci. 2003;53:217–223.
- Appurao AG, Narasinga Rao MS. Binding of Ca by the 11S fraction of soybean proteins. Cereal Chem. 1975;52:21–33.
- Sakakibara M, Noguchi H. Interaction of 11S fraction of soybean protein with calcium ion. Agric. Biol. Chem. 1977;41:1575–1580.
- Torikata Y, Ishihara J, Yano T. Protein coagulation through reversible and irreversible bindings of calcium. Agric. Biol. Chem. 1987;51:707–714.
- Appurao AG, Narasinga Rao MS. Binding of Ca(II), Mg(II), and Zn(II) by 7S fraction of soybean proteins. J. Agric. Food Chem. 1976;24:487–490.
- Appurao AG, Narasinga Rao MS. Binding of Zn(II) by 11S fraction of soybean proteins. J. Agric. Food Chem. 1976;24:490–494.
- Yuan YJ, Velev OD, Chen K, Campbell BE, Kaler EW, Lenhoff AM. Effect of pH and Ca2+-induced associations of soy bean proteins. J. Agric. Food Chem. 2002;50:4953–4958.
- Allred JB, Kratzer FH, Porter JW. Some factors affecting the in vitro binding of zinc by isolated soya-bean protein and by α-casein Br. J. Nutr. 1964;18:575–582.
- Furia TE. Chapter 6 of sequestrants in foods. In: Furia TE, editor. CRC handbook of food additives. 2nd ed. Vol. I. (FL). New York: CRC Press; 1981. p. 271–294.