Abstract
Casimiroa edulis is known as cochitzapotl, and it belongs to a species of tropical fruiting tree in the family Rutaceae, native to eastern Mexico and Central America south to Costa Rica. In this study, we isolated two furocoumarins and two polymethoxyflavones from leaves of C. edulis and evaluated the functions of glucose and lipid metabolism activity with 3T3-L1 adipocytes. We discovered that the addition of furocoumarins increased glucose uptake and lipid accumulation in 3T3-L1 adipocyte. These results suggest that furocoumarin compounds can be used as functional food-derived compounds, to regulate adipocyte functioning for the management of metabolic syndrome, which is associated with dysfunctions of glucose and lipid metabolism.
Graphical Abstract
Phenolic compounds from leaves of Casimiroa edulis showed adipogenesis activity of 3T3-L1 adipocytes
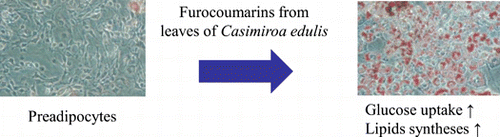
Casimiroa edulis is a species of tropical fruiting tree. Zapotin in C. edulis has been found to induce both cell differentiation and apoptosis in cultured human promyelocytic leukemia HL-60 cells.Citation1) This compound inhibits 12-O-tetradecanoylphorbol 13-acetate (TPA)-induced ornithine decarboxylase activity in human bladder carcinoma cells (T24 cells) and TPA-induced nuclear factor-kappa B activity in human hepatocellular liver carcinoma cells.Citation2) The data suggest that zapotin deserves further investigation as a potential cancer suppressive agent. Crude plant extracts of seeds and of leaves of C. edulis have been found to affect blood pressure, cardiac activity,Citation3–5) and aortic muscular tone,Citation6) and to possess anticonvulsant activity,Citation7,8) but previous study has focused mainly on seed components and leaf components that were not studied well.
Adipocytes play a major role in the maintenance of energy balance. The amount of glucose uptake into the adipocytes is significant in the maintenance of serum glucose levels, because adipocyte-specific knockout of an insulin-dependent glucose transporter (glut4) results in diabetic symptoms.Citation9) Lipid synthesis (lipogenesis) is also a vital function of adipocytes. In vivo studies have suggested that adipose tissue is responsible for more than 50% of fatty acid (FA) synthesis in mammals.Citation10) FA synthesis is a cytosolic process utilizing acetyl CoA as precursor. It is generally accepted that FA is synthesized from various substrates (glucose, pyruvate, lactate, and acetate).Citation11) It is indispensable for the management of adipocyte functions to evaluate complicated metabolisms, including lipogenesis and glucose uptake.
In this study, we tried to isolate phenolic compounds from C. edulis leaves, and investigated other effect, adipogenesis, including glucose uptake, FA synthesis activity, and lipid accumulation with 3T3-L1 adipocytes. PPARγ synthesized ligand, pioglitazone, was used as positive control, since PPARγ is abundantly expressed in 3T3-L1 adipocytes and plays a central role in metabolism and differentiation.Citation12) Pioglitazone, a major insulin sensitizer, promotes preadipocyte metabolism including glucose uptake and FA synthesis through PPARγ activationCitation13) and is widely used in the treatment of type 2 diabetes.
Materials and methods
Leaves of C. edulis were collected at the Botanical Garden of Nippon Shinyaku Co., Kyoto, Japan. The voucher specimen was deposited at Gifu Pharmaceutical University. 13C6 glucose and pioglitazone were purchased from Sigma (St. Louis, MO). [1,2,3,4,5,6,7,8,9,10,11,12,13,14,15,16-13C] palmitic acid was from Watari (Tokyo). Unless otherwise indicated, all chemicals were purchased from Nacalai Tesque (Kyoto, Japan) or Wako Pure Chemicals (Osaka, Japan) and were of guaranteed reagent grade or tissue culture grade.
General experimental procedures
1H and 13C NMR spectra were recorded on an EX400 (1H at 400 MHz and 13C at 100 MHz, JEOL) spectrometer. Chemical shifts appeared as δ values with trimethylsilane as internal reference. Peak multiplicities were quoted in Hz. EI-MS was recorded on a JMSDX-300 (JOEL) spectrometer. Kiesel gel 60 (60–230 mesh, Merck, Japan) and Fuji Silysia Chemical Chromatorex ODS (100–200 mesh) were used for column chromatography. Kiesel-gel 60 F256 (Merck, Tokyo) at 0.25 mm was used for analytical and 0.5 mm for preparative TLC.
Extraction and isolation of compounds
Dried leaves (550 g) were extracted with MeOH (1 L × 5 times) at room temperature and were evaporated in vacuo. The MeOH extract (52 g) obtained was chromatographed on Silica gel (250 mm × 30 mm) with a mixture of chloroform–MeOH by increasing the polarity from 0 to 10% methanol. The chloroform–MeOH (10:1) (3.5 g) was further chromatographed on an ODS column (250 mm × 20 mm) eluted with H2O, followed by increasing the concentration of MeOH to give four fractions. Fraction 2 (263 mg, 70% methanol) was repeatedly purified by preparative TLC (n-hexane-EtOAc 1:4) to give 1 (5 mg), 2 (7 mg), 3 (12 mg), and 4 (10 mg).
8-(3ʹ-Hydroxymethyl-but-2-enyloxy)-psoralen acetate (compound 1)
White amorphous solid. Negative ion FABMS [M-H]– m/z 327. 1H NMR(CDCl3) δ: 1.81 (3H, br s, H-5ʹ), 2.04 (3H, s, acetyl-Me), 4.66 (2H, br s, H-4ʹ), 5.09 (2H, br d, J = 7.0 Hz, H-1ʹ), 5.86 (1H, br t, J = 7.0 Hz, H-3ʹ), 6.38 (1H, d, J = 10.0 Hz, H-4), 6.82 (1H, d, J = 2.5 Hz, H-9), 7.37 (1H, s, H-5), 7.69 (1H, d, J = 2.5 Hz, H-10), 7.77 (1H, d, J = 10.0 Hz, H-3): 13C NMR (CDCl3) δ: 160.43 (C-2), 114.83 (C-4), 144.27 (C-4), 116.54 (C-4a), 113.81 (C-5), 125.95 (C-6), 148.32 (C-7), 131.39 (C-8), 143.67 (C-8a), 106.76 (C-9), 146.76 (C-10), 69.12 (C-1ʹ), 125.13 (C-2ʹ), 136.57 (C-3ʹ), 62.79 (C-4ʹ), 21.42 (C-5ʹ), 20.83 (acetyl-Me), 170.85 (acetyl C=O).
8-(3ʹ-Hydroxymethyl-but-2-enyloxy)-5-methoxypsoralen acetate (compound 2)
White amorphous solid. Negative ion FABMS [M-H]– m/z 357. 1H NMR(CDCl3) δ: 1.80 (3H, s, H-5ʹ), 2.03 (3H, s, acetyl Me), 4.18 (3H, s, C-5 OMe), 4.66 (2H, br s, H-4ʹ), 4.91 (2H, br d, J = 7.0 Hz, H-1ʹ), 5.86 (1H, br t, J = 7.0 Hz, H-2ʹ), 6.28 (1H, d, J = 10.1 Hz, H-3), 6.99 (1H, d, J = 2.5 Hz, H-9), 7.64 (1H, d, J = 2.5 Hz, H-10), 8.12 (1H, d, J = 10.1 Hz, H-4); 13C NMR (CDCl3) δ: 160.42 (C-2), 112.91 (C-3), 139.55 (C-4), 107.61 (C-4a), 144.26 (C-5), 114.61 (C-6), 150.57 (C-7), 136.51 (C-8), 125.63 (C-8a), 105.12 (C-9), 145.16 (C-10), 69.36 (C-1ʹ), 125.27 (C-2ʹ), 136.51 (C-3ʹ), 62.78 (C-4ʹ), 21.41 (C-5ʹ), 20.84 (acetyl Me), 170.84 (acetyl C=O), 60.79 (3H, s, C-5 OMe).
5,6,2ʹ,3ʹ,5ʹ,6ʹ-Hexamethoxyflavone (compound 3). White amorphous solid: exhibited spectral data (1H NMR and EIMS) comparable to published data.Citation14)
5,6,2ʹ-Trimethoxyflavone (compound 4). White amorphous solid: 1H NMR data was comparable to published dataCitation6); 13C NMR (CDCl3) δ: 159.05, 157.90 (C-2, C-2’), 111.68 (C-2), 178.41 (C-4), 119.07, 119.02 (C-4a, 8), 147.82 (C-5), 149.65 (C-6), 113.33 (C-7), 151.82 (C-8a), 120.65 (C-1ʹ), 112.96 (C-3ʹ), 132.13 (C-4ʹ), 120.62 (C-5ʹ), 129.07 (C-6ʹ).
LC/MS system
The Agilent Series 1100 LC system (Agilent Technologies, Waldbronn, Germany) consisting of a G1323B control module, a G1312A quaternary pump, a G1322 degasser, a G1329A, a G1330A autosampler, a G1316A column oven, and a G1314A detector was used. The column used was an XbridgeTM (C18 3.5 μm, 2.1 mm× 150 mm, Waters, Milfold, MA). The LC pump gradient was as follows: 85% mobile phase A (water in 5 mm ammonium acetate) and 15% mobile B (methanol in 5 mm ammonium acetate) from 0 to 20 min, 99% mobile phase A and 1% mobile phase B from 20 to 20.1 min, and 85% mobile phase A and 15% mobile B from 20.1 to 35 min. The injection volume, flow rate, and column temperature were 5 μL, 0.2 mL, and 40 °C, respectively. MS conditions were selected ion monitoring: polarity negative; 10 psi nebulizer gas, 150 V fragment ion voltage; 3,000 V Capillary voltage, and 350 °C; dwell time 50 ms. Unless otherwise specified, all reagents used were of analytical grade, and the water was deionized. Solvent: LC/MS grade methanol. [1,2,3,4,5,6-13C] glucose and [1,2,3,4,5,6,7,8,9,10,11,12,13,14,15,16-13C] palmitic acid were detected at 185 and 271, m/z, respectively.Citation15)
Spectra of furocoumarins were determined in LC/MS scan mode, polarity negative (50−400 m/z).
Gas chromatograph mass spectrometer system
Gas-chromatographic (GC) analyses were performed using an Agilent 6890 quadrupole GC equipped with an Agilent 5973B mass spectrometer (MS). The GC column was a DB-5MS + DG capillary type (30 m × 0.25 mm i.d. 0.25 μm film thickness; J&W Scientific, Folsom, CA). A 1-μL extract was injected in splitless mode at an injection temperature of 250 °C. The oven temperature was programmed to increase from an initial 60 °C (held for 1 min) to 300 °C (held for 2 min) at a rate of 20 °C/min. The temperatures of the quadrupole and ion source were 150 and 230 °C. The GC-MS system was operated in scan mode (50–500 m/z) with the electron multiplier tune value.
Cell culture
3T3-L1 preadipocytes, purchased from the American Type Culture Collection (Rockville, MD), were grown in DMEM-high glucose medium supplemented with 10% fetal bovine serum, 100 U/mL of penicillin, and 100 μg/mL streptomycin at 37 °C in 5% CO2 using a 12-well plate (5 × 104 cell/well). Post-confluent preadipocytes were differentiated into adipocytes by adding 10 μg/mL insulin, 0.1 μg/mL dexamethasone, and 112 μg/mL isobutylmethylxanthine to the medium with 5 μm pioglitazone, and 5 μm of each test compound in DMSO, as previously described.Citation12) The differentiation medium was removed after two days and replaced with DMEM-high glucose medium containing 5 μg/mL insulin with TZD or the test compound every two days. Ten days after the addition of the differentiation medium, the cells were plated in culture dishes for a given experiment. After differentiation, they were washed with warm PBS, and the medium was replaced with glucose-free DMEM containing 4.5 mg/mL [1,2,3,4,5,6-13C] glucose. They were incubated for a further 48 h. This was repeated twice.
Extract and saponification
The media were collected after incubation for specific durations and diluted with methanol. Diluents were filtered with a 0.22 μm filter membrane, and were analyzed by the LC/MS system. The cells were dissolved in 5 mL KOH 20% (ethanol:water 4:6; v/v), and hydrolyzed for 60 min at 80 °C, followed by acidification with 6 mL 5 N HCl. The FAs were extracted twice with 4 mL diethylether, dried under N2 at 40 °C, dissolved in 5 mL methanol, and analyzed by the LC/MS system.
Oil Red O staining
Cells were fixed with 10% formaldehyde/PBS and stained with Oil Red O solution (0.5% Oil Red O-isopropyl alcohol/H2O (3:2, v/v)).
Statistical analysis
Results are expressed as mean and standard error of mean. Statistical significance of differences among groups was evaluated by Student’s t-test. Differences were considered significant at p < 0.05.
Results and discussion
NMR analysis
The 1H NMR of compound 1 showed the presence of two sets of coupled protons that δ 6.38 and 7.77 (J = 10.0 Hz) and δ 6.82 and 7.69 (J = 2.5 Hz). The data indicated compound 1 was a furocoumarin. An aromatic singlet (δ 7.37) was also observed that correlated with the C-4 signal of the coumarin skeleton in the HMBC spectrum. Other HMBC correlations indicated that the furan ring was attached to C-6, 7, like psoralen. 1H and 13C NMR showed the presence of two oxymethylene (δΗ 4.66 and 5.09, and δC 62.79 and 69.12), a tri-substituted double bond (δH 5.09; δC 125.13 and 136.57), a methyl attached to a double bond (δH 1.81; δC 21.41), and an acetyl group (δH 2.04; δC 20.83 and 170.85). In the HMBC spectrum, an oxymethylene proton was correlated with C-8 of a coumarin. These data are characteristic of a prenyloxy group, in which one of methyl groups had been oxidized to a hydroxyl methyl and acetylated. The hydroxymethyl group was of the cis form with respect to the oxymethylene group (H-1ʹ) of the prenyl substituent on the basis of the NOESY spectrum (H-1ʹ/H-4ʹ and H-2ʹ/H-5ʹ). Therefore, the structure was determined to be as described in Fig. . This compound was first isolated from leaves of Casimiroa pringlei.Citation16,Citation17)
Fig. 1. Structures of compounds isolated from leaves of C. edulis. Furocoumarins (compounds 1 and 2), polymethoxyflavones (compounds 3 and 4).
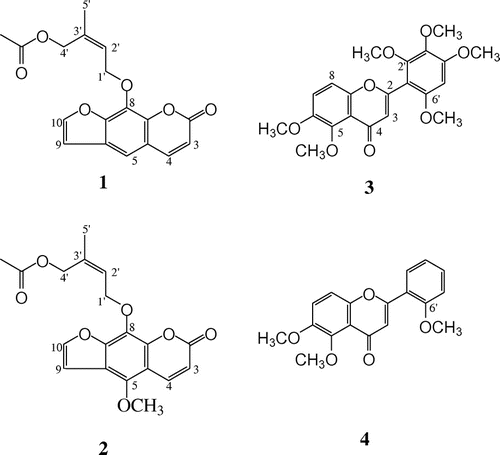
The 1H NMR spectrum of compound 2 was very similar to that of 1, but an aromatic proton disappeared and a methoxy group (δ 4.18) was observed. An NOE was observed between the methoxy group and H-4 of the coumarin skeleton. By detailed analysis of NMR by 2D methods, the structure of 2 was determined to 5-methoxylated compound of 1. A deacetylated coumarin of 2 was isolated from Casimiroa tetrameria. To the best of our knowledge, compound 2 was a new compound.
The structures of compounds 3 and 4 were determined and identified by comparison to previous data as 5,6,2ʹ,3ʹ,5ʹ,6ʹ-hexamethoxyflavone (3)Citation18) and 5.6.2’-trimethoxyflavone (4),Citation14,18) respectively.
Fig. shows spectra of furocoumarins and polymethoxyflavones. Parent ions of them as determined by NMR were obtained by LC/MS and GC/MS analysis (327, 357, 402, 312 m/z). This result confirms the NMR analysis data.
Glucose uptake and glycerol output activity
To elucidate the effects of compounds 1–4 on adipocyte functioning, we examined the insulin-dependent glucose uptake and glycerol output of differentiated 3T3-L1 adipocytes. As shown in Fig. (A), the addition of 5 μm compounds 1 and 2 enhanced the insulin-dependent uptake of glucose (1.2–1.3-fold increases as compared to control). The addition of the other compounds did not enhance insulin-dependent uptake of glucose. On the other hand, the addition of 5 μm compounds 1–4 did not enhance glycerol output or lipolysis (Fig. (B)). These compounds at the concentrations used in this study did not affect cell viability (data not shown).
Fig. 3. 13C6-Glucose Uptake (A), Glycerol Output (B), and De novo 13C-FA Synthesis (C) in 3T3-L1 Adipocytes after the addition of compounds as determined by LC/MS analysis of the medium at 48 h. Incubation. 13C6-Glucose uptake and glycerol output of 3T3-L1 adipocytes after addition of coumarins and polymethoxyflavones were determined by LC/MS. The concentrations of 13C6-glucose and glycerol were determined by the LC/MS system at 185 m/z (13C6-glucose) and 91 m/z (glycerol) respectively. De novo synthesis of palmitic acid was monitored by analyzing 271 (m/z). Lane 1, control; lane 2, pioglitazone; lanes 3–4, compounds 1 and 2; lanes 5 and 6, compounds 3 and 4, respectively. Lane 1, control; lane 2, pioglitazone; lanes 3 and 4, compounds 1 and 2; lanes 5 and 6, compounds 3 and 4, respectively. Concentrations (in μm) at all data points is 5.0 **p < 0.05 compared to control.
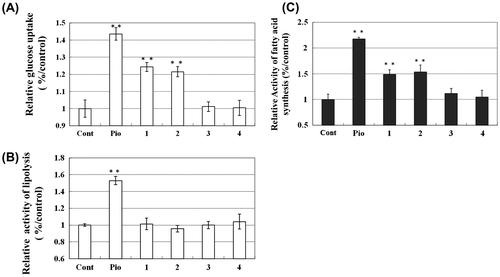
De novo FA synthesis activity and lipid accumulation of adipocytes
To assess the effects of compounds 1–4 on lipid metabolism in 3T3-L1 adipocytes, we analyzed De novo FA syntheses activity with compounds 1–4. Fig. (C) shows new synthesis of palmitic acid in 3T3-L1 adipocytes treated with compounds 1–4 for 48 h. The addition of pioglitazone at (5.0 μm) increased the amounts of synthesized FA, by approximately 2.2-fold in the 3T3-L1 adipocytes as compared with control. The addition of 5.0 μm of compounds 1 and 2 enhanced FA syntheses by approximately 1.5-fold as compared with control. Finally, to investigate the dose-dependent effects of furocoumarins on the lipid accumulation of adipocytes, we performed Oil Red O staining. The addition of furocoumarins increased lipid accumulation dose-dependently (Fig. ).
Fig. 4. De novo 13C-FA Synthesis and Lipid Accumulation of 3T3-L1 Adipocytes. Adipocytes treated with pioglitazone or compounds 1,2 (furocoumarins) (1–100 μm) were fixed with 10% formaldehyde/PBS and stained with Oil Red O solution (0.5% Oil Red O-isopropyl alcohol/H2O 3:2, v/v). Microscopy views of representative 3T3-L1 cells (original magnification × 100).
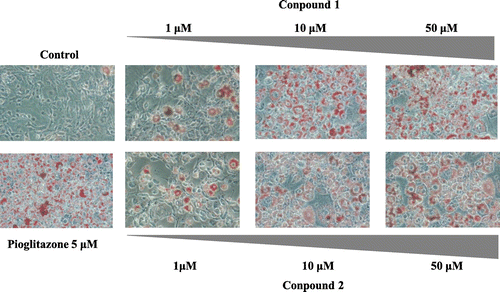
Obesity and associated disorders are the major noncommunicable public health problems of the twenty-first century. Studies indicate that high levels of body fat are associated with an increased risk of developing numerous adverse health conditions.Citation19,20) Hence, effective therapeutic approaches to obesity and obesity-induced metabolic syndrome are of wide interest. In this study, we isolated two furocoumarins and two polymethoxyflavones from leaves of C. edulis fruit and evaluated the functions of glucose and lipids metabolism activation. We found that the addition of furocoumarins (compounds 1 and 2) caused increased glucose uptake, FA synthesis, and lipid accumulation in 3T3-L1 adipocytes. Furanocoumarins are known to have biological effects in humans. For example, bergamottin and dihydroxybergamottin are attributable to the grapefruit juice effect, in which these furanocoumarins affect the metabolism of certain drugs,Citation21) but the concentration of furanocoumarins used in our assays had no influence on cell viability. They might lower blood glucose levels by orally ingested in a meal or an ordinary drink, and this calls for further evaluation in vivo.
Notes
Abbreviations: LC/MS, liquid chromatography mass spectrometry; PPAR, peroxisome proliferator-activated receptor; FA, fatty acid.
References
- Mata-Greenwood E, Ito A, Westenburg H, Cui B, Mehta RG, Kinghorn AD, Pezzuto JM. Anticancer Res. 2001;21:1763–1770.
- Maiti A, Cuendet M, Kondratyuk T, Croy VL, Pezzuto JM. J. Med. Chem. 2007;50:350–355.10.1021/jm060915+
- Garcia-Gonzalez M, Freer BE, Morales MO. Rev. Biol. Trop. 1994;42:115–119.
- Vidrio H, Magos GA. Planta Med. 1991;57:217–220.10.1055/s-2006-960077
- Magos GA, Vidrio H, Enríquez R. J. Ethnopharmacol. 1995;47:1–8.10.1016/0378-8741(95)01247-B
- Garzón-De la Mora P, Garcı́a-López PM, Garcı́a-Estrada J, Navarro-Ruı́z A, Villanueva-Michel T, et al. J. Ethnopharmacol. 1999;68:275–282.10.1016/S0378-8741(99)00125-7
- Navarro RA, Bastidas RBE, Garcia EJ, Garcia LP, Garzon P. J. Ethnopharmacol. 1995;45:199–206.
- Mata-Greenwood E, Ito A, Westenburg H, Cui B, Mehta RG, Kinghorn AD, Pezzuto JM. Anticancer Res. 2001;21:1763–1770.
- Spiegelman BM, Flier JS. Cell. 2001;104:531–543.10.1016/S0092-8674(01)00240-9
- Ingle DL, Bauman DE, Garrigus US. J. Nutr. 1972;102:617–623.
- Saggerson ED. Biochem. J. 1974;142:477–482.
- Tzameli I, Fang H, Ollero M, Shi H, Hamm JK, et al. J. Biol. Chem. 2004;279:36093–36102.10.1074/jbc.M405346200
- Spiegelman BM, Flier JS. Cell. 1996;87:377–389.10.1016/S0092-8674(00)81359-8
- Eckard W, Munekazu I, Toshiyuki T, Mizuo M. Phytochemistry. 1990;29:633–637.
- Nagai H, Ebisu S, Abe R, Goto T, Takahashi N, Hosaka T. Biosci. Biotechnol. Biochem. 2011;75:337–341.10.1271/bbb.100810
- Emma M, Imeida M, Fernando C, Alfredo O. Heterocycles. 2008;755:3057–3064.
- Bilkis H, Horst R, Anita A, Otto S, Simon G, et al. Phytochemistry. 2005;66:649–652.
- Ito A, Shamon LA, Yu B, Mata-Greenwood E, Lee SK, et al. J. Agric. Food Chem. 1998;46:3509–3516.10.1021/jf9802373
- Cornier MA, Dabelea D, Hernandez TL, Lindstrom RC, Steig AJ, et al. Endocr. Rev. 2008;29:777–822.10.1210/er.2008-0024
- Yach D, Stuckler D, Brownell KD. Nature Med. 2006;12:62–66.10.1038/nm0106-62
- Kakar SM, Paine MF, Stewart PW, Watkins PB. Clin. Pharmacol. Ther. 2004;75:569–579.10.1016/j.clpt.2004.02.007