Abstract
This study aimed to investigate the biotransformation of soybean isoflavones to hydroxyisoflavones, and the primary and secondary metabolite change during Aspergillus oryzae KACC40247-mediated fermentation by gas chromatography–time of flight-mass spectrometry and LC-MS with multivariate analysis. The mass spectrometric analysis revealed that acetylglycosides and glycosides decreased during the first 12 h of fermentation, while the aglycones increased up to that time point. This was followed by a decrease in aglycone levels due to the formation of hydroxyisoflavones. The hydroxyflavones, 8-hydroxydaidzein, hydroxygenistein, and hydroxyglycitein, resulting from the biotransformation of the corresponding aglycones, increased up to 24 h, and then subsequently decreased. During fermentation, the levels of monosaccharides, aspartic acid, pyroglutamic acid, gamma-aminobutyric acid, and organic acids gradually decreased, whereas the levels of threonine, serine, and glycine increased. Hydroxyisoflavone was more strongly correlated with antioxidant activity than the other metabolites. Our results suggest that biotransformation has the potential to improve the nutritional properties of soy-based food.
Soybeans are generally regarded as a healthy food because of their functional and nutritional components, including isoflavones, saponins, and phytic acids.Citation1) Fermented soybean products such as miso, tofu, and tempeh are known for their nutritional value due to improved metabolic contents.Citation2,Citation3) Recently, soybean isoflavones have received much attention due to their potential health benefits and their possible roles in preventing certain endocrine disorders and other diseases such as cancer and cardiovascular disease.Citation3Citation−Citation5)
The production of traditional foods such as doenjang, miso, and sake in Korea involves fermentation by the fungus koji, which belongs to the group Aspergillus sp. This organism produces various enzymes during the fermentation process.Citation6,Citation7) The isoflavone metabolism occurring when soybean-based food is fermented by Aspergillus sp. has been well studied, and it involves the hydrolysis of isoflavone glycosides and the hydroxylation of isoflavone aglycones.Citation8) The antioxidant properties of fermented soybean broth were studied for its reducing power, radical scavenging activity, and chelating effects.Citation9) Since active oxygen causes the oxidation of food components, followed by the release of a plethora of potentially harmful free radicals within living systems, antioxidant activity is an important factor determining the nutritional quality of food products.Citation10,Citation11)
In our investigations to understand the biochemical processes that occur during the fermentation of soybeans, the metabolomic approaches of liquid chromatography-electrospray ionization-mass spectrometry (LC-ESI-MS) and gas chromatography–time of flight-mass spectrometry (GC-TOF-MS) were applied. Metabolomics is defined as the study of all small molecules, such as metabolites, within food, plants, cells, and organs.Citation12,Citation13) For example, metabolite profiling has been applied to food quality control, toxicology, and medical diagnosis.Citation14) In the field of food sciences, it was initially used to assess food quality and food adulteration and to characterize food components.Citation15) Due to its sensitivity and accuracy in detecting metabolites, this technique has been implemented in various fields to improve overall quality.
Soy-based foods are considered to be potential therapeutics for various diseases, and studies of their metabolism have indicated possible applications. For example, soybean has been found to be rich in isoflavones, particularly glycoside moieties, but these glycosides are not directly absorbed in the human intestine.Citation16) Instead, intestinal bacteria first affect pre-hydrolytic removal of sugar moiety with the β-glucosidase enzyme, helping the body to absorb these compounds. For this reason, the biotransformation of glycosides to the corresponding aglycones improves the nutritive potential of these isoflavones. Although biotransformation of isoflavones has been reported, the levels of other secondary and primary metabolites generated during these processes remain unknown.Citation17) Acquiring an understanding of the levels of other metabolites produced during the biotransformation process should allow for improvement of the end products of this process and should improve the efficiency of the biosynthetic pathway. Hence, a study of the biotransformation of soybean isoflavones by Aspergillus oryzae KACC 40247 was carried out, along with an assessment of relative changes in the levels of the other metabolites during the fermentation process.
Materials and methods
Chemicals and reagents
High-performance liquid chromatography-grade acetonitrile, water, methanol, and ethyl acetate were purchased from Fisher Scientific (Pittsburgh, PA). Methoxyamine hydrochloride, N-methyl-N-(trimethylsilyl) trifluoroacetamide (MSTFA), formic acid, pyridine, potassium persulfate, 2,2′-azinobis (3-ethylbenzothiazoline-6-sulfonic acid) (ABTS) diammonium salt, and six standards (daidzin, glycitin, genistin, daidzein, glycitein, and genistein) with purities > 98% were from Sigma Aldrich (St. Louis, MO).
Preparation of soybean extract
Soybeans (250 g) were purchased from a local market and soaked overnight in 1 L of water at 26 °C. The mixture was heated to 105 °C for 60 min, cooled to room temperature, and then filtered through a sieve. The small and large particles in the sieved mixture were removed by centrifugation at 3,000 × g over 30 min at 4 °C, and the supernatant was filtered through Whatman filter paper No. 2. The filtrate was then autoclaved at 121 °C for 15 min and used as the medium in the biotransformation studies.
Fungal strain and culture conditions
Spores of the fungus A. oryzae KACC 40247 were grown on a potato dextrose agar (PDA) plate at 26 °C for 120 h. Eight PDA pieces (10 mm × 10 mm) from the plate were used to inoculate a 100-mL baffled flask containing 25 mL of soybean extract, and this was cultivated at 30 °C, pH 6.0, and at 200 rpm for 48 h. During this time, cultures were collected at regular intervals (0, 12, 18, 24, 30, 36, 42, and 48 h) for further analysis.
Metabolite extraction and analysis
Samples (50 mL) collected from the fermenter at regular intervals were centrifuged at 4 °C at 5,000 rpm for 15 min (Universal 320, Hettich Zentrifugen, Germany). From the supernatant, 20 mL was harvested and extraction was performed by adding an equal volume of ethyl acetate. The extracts were evaporated with a speed vacuum machine (Biotron, Seoul, Korea), and resuspended in methanol. The suspension was analyzed by LC-ESI-MS in order to detect secondary metabolites. For detection of primary metabolites, the extracts were derivatized with methoxyamine hydrochloride (20 mg/mL) in pyridine and oximated at 30 °C for 90 min. Then, the oximated samples were silylated with MSTFA at 37 °C for 30 min and analyzed by GC-TOF-MS. Three analytical replications along with experimental duplicates from each fermented sample were examined to assess the biotransformation of secondary metabolites and the changes in primary metabolites during the fermentation process.
GC-TOF-MS analysis
An Agilent 7890A gas chromatography (GC) system equipped with an Agilent 79693 autosampler coupled to a Pegasus Time-of-Flight Mass Spectrometer (TOF-MS) detector (Leco, St. Joseph, MI) was used in the analyses. An Agilent 30 m HP-5MS capillary column with an internal diameter of 0.25 mm and a film thickness of 0.25 μm was employed for separation. Chromatographic-grade helium at a constant flow of 1.0 mL/min served as carrier gas. The oven temperature was maintained at 70 °C for 2 min, and then ramped to 300 °C at a rate of 10 °C/min and maintained at 300 °C for 5 min. The mass data, collected in EI mode with 70 eV ionization energy, were used for a full scan at m/z 45−1,000. The injector and transfer line temperatures were 250 and 240 °C, respectively. The reaction mixtures were transferred to 2-mL GC autosampler vials, and 1 μL of reactant was injected into the GC-TOF-MS without split.
LC-ESI-MS analysis
Samples were analyzed with a 500-MS ion-trap mass spectrometer from Varian Technologies (Palo Alto, CA), which consisted of a 212-liquid chromatography (212-LC) binary solvent delivery system, a Prostar 335 photodiode array detector, and a Prostar 410 autosampler. Samples were separated on a Varian PurSuit XRs C18 column (i.d. 3 μm, 100 × 2.0 mm) with a MetaGuard 2.0 PurSuit XRs C18 guard column (Varian) at a column oven temperature of 30 °C. The mobile phases were 0.1% formic acid in water (A) and acetonitrile (B), and the gradient flow was as follows: from 5% to 60% B (v/v) at 30 min, then sharply increasing to 100% B (v/v) for 0.06 min, followed by holding at 100% B (v/v) for 35 min, after which it was decreased to 5% B (v/v) at 35.06 min, and that was maintained for 40 min. The flow rate was 0.2 mL/min, and the injection volume was 10 μL. Mass spectra were obtained by electrospray ionization in negative mode within a range of m/z 100–1,000. The operating parameters were as follows: spray needle voltage, ±5 kV; capillary voltage, 80 V; drying gas temperature, 350 °C; drying gas pressure, 10 psi (nitrogen); and nebulizer gas pressure, 35 psi (air). Tandem MS analysis was carried out by scan-type turbo data-dependent scanning under the conditions used for negative mode MS scanning.
Data processing and multivariate analysis
LC-MS data (*.xms) were acquired using Varian MS Workstation 6.9 software (Varian, USA). The data files were converted to the netCDF (*.cdf) format using Vx Capture (version 2.1; Adron systems, Laporte, MN). GC-TOF-MS data files were converted to the CDF format using ChromaTOF software v4.44 (Leco, St. Joseph, MI). After conversion, the MS data were processed using the metAlign software package (http://www.metalign.nl) to obtain a data matrix containing retention times, accurate masses, and normalized peak intensities. The resulting data matrix, which contained the sample name and peak area information as variables, was processed using SIMCA-P + 12.0 (Umetrics, Umea, Sweden) for multivariate statistical analysis. The data-sets were log-transformed and UV-scaled prior to principal component analysis (PCA) and partial least squares discriminant analysis (PLS-DA) modeling. Based on variable importance in the projection (VIP) values (value > 1.0) from the model and p value (< 0.05) statistics, variables were selected for further identification and analysis. Pairwise metabolite-antioxidant effect correlations were determined by Pearson’s correlation coefficient test using PASW Statistics 18 (SPSS, Chicago, IL). The metabolites that varied between time intervals were plotted and compared by generating a heat map by MEV software version 4.8 (multiple array viewer, http://www.tm4.org/).
Assay for antioxidant activity
Antioxidant activity was monitored using ABTS by a modification of the procedure of Re et al.Citation18) In brief, ABTS (7 mm) was dissolved in potassium persulfate (2.45 mm) and maintained for 12–16 h in the dark at room temperature. After color development (to dark blue), the solution was diluted until the absorbance reached 0.7 ± 0.02 at 750 nm as measured by spectrophotometer (Spectronic Genesys 6, Thermo Electron, Madison, WI). Soybean extract (10 μL) was combined with 190 μL of the ABTS solution, and the mixture was incubated for 6 min in the dark. Then, the absorbance at 750 nm was measured with a spectrophotometer. The results were expressed in Trolox equivalent activity concentration (mm) with Trolox standards in a range of 0.0625–1 mm.
Results and discussion
Primary metabolite profiling of fermented soybean extracts
Multivariate analysis was done to investigate the metabolic variation of the soybean extracts fermented by A. oryzae at different time intervals across a 48-h fermentation time course. Fig. (A) shows PCA (left) and PLS-DA (right) score plots derived from GC-TOF-MS of the fermented soybean extracts. The results revealed a clear clustering of the fermented extract samples based on the time point of removal. The PCA score plot revealed the variables by PC1 (24.4%) and PC2 (17.4%), respectively. In the PLS-DA score plot, PLS1 explained 24.4% of total variance, and PLS2 explained 17.4% of it (p < 0.05). Percent scores (explained variation) measure the extent to which a mathematical model accounts for the variation of a given data-set (MS, peak intensity, and retention time). Thus, the results revealed a clear clustering of the fermented extract samples based on the time point of removal. The changes in metabolite levels in the soybean extracts over the fermentation time course were revealed mostly by the PLS1 score.
Fig. 1. PCA, left and PLS-DA, right.
Notes: Score plots were derived using GC-TOF-MS (A) and LC-ESI-MS (B) data-sets from soybean extracts that were harvested at the 8 indicated time points during fermentation period. Each time point examined is been represented on the plot by a unique symbol: ■, 0 h; □, 12 h; ▲, 18 h; △, 24 h; ▼, 30 h; ▽, 36 h; ●, 42 h; ○, 48 h.
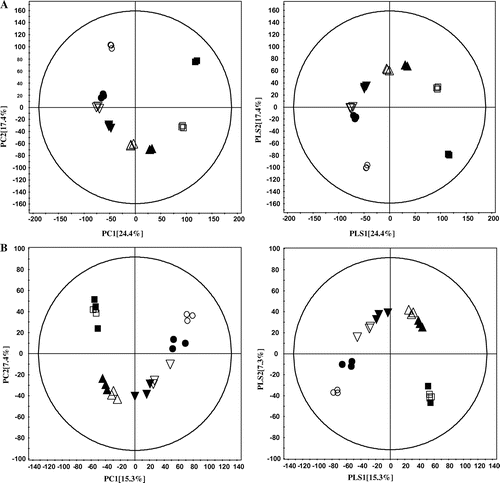
Differential variables were selected based on VIP (VIP > 1.0) values and p values (p < 0.05) obtained by the PLS-DA, and a total of 23 primary metabolites were identified (Table ). The metabolites identified as displaying variation over the fermentation time course consisted of amino acids (glycine, serine, threonine, aspartic acid, pyroglutamic acid, and gamma-aminobutyric acid), fatty acids (pentadecanoic acid, palmitic acid, and stearic acid), sugars and sugar derivatives (ribose, arabitol, tagatose, fructose, galactose, mannitol, sorbitol, myo-inositol, and maltose), and organic acids (citric acid, malonic acid, cinnamic acid, and gluconic acid). The relative levels of amino acids such as threonine, serine, and glycine gradually increased, whereas the relative levels of other amino acids such as aspartic acid, pyroglutamic acid, and gamma-aminobutyric acid decreased during fermentation. Amino acid changes during A. oryzae fermentation of soybean have been studied, and the results revealed that the amino acids did not display a uniform pattern of increase or decrease during fermentation.Citation19Citation−Citation21) The same trend was also observed in the present study. It was also found that over the fermentation time course, the levels of fatty acids increased, while the levels of most of the organic acids decreased. The increase in fatty acids might have been due to a lipase produced by A. oryzae that hydrolyzes not only triacylglycerols, but also monoacylglycerols and diacylglycerols.Citation22) With respect to the sugar content, the relative levels of identified monosaccharides, such as galactose and fructose, showed a pattern of decrease, whereas the levels of disaccharides increased up to 42 h, after which they decreased. Sugar was used as a carbon source to stimulate α-amylase production by A. oryzae. The potential of sugars such as maltose, glucose, fructose, galactose, sucrose, glycerol, mannitol, and acetate to act as a carbon source for A. oryzae has been investigated.Citation23)
Table 1. Primary metabolites displaying significant variation in levels during the fermentation of soybean extract by A. oryzae.
Secondary metabolite profiling of fermented soybean extracts
To investigate the changes in secondary metabolite levels in soybean extracts at various time intervals over the time course of fermentation by A. oryzae, PCA and PLS-DA (Fig. (B)) analyses were done on LC-ESI-MS data-sets. The PLS-DA (Fig. (B), right) and PCA (Fig. (B), left) score plots showed a clear separation between the soybean extracts depending on the time point during fermentation. In the LC-ESI-MS analysis, PLS1 explained 15.3% of the total variance and PLS2 explained 7.3% of it. The changes in metabolite levels in the soybean extracts over the fermentation period were revealed mostly by the PLS1 score. A total of 13 secondary metabolites that showed significant variation across the fermentation time course were selected from the PLS-DA, based on VIP and p values (Table ). Three acetyl glycosides (acetyldaidzin, acetylgenistin, and acetylglycitin), three glycosides (daidzin, genistin, and glycitin), three aglycones (daidzein, genistein, and glycitein), three hydroxyisoflavones (8-hydroxydaidzein, hydroxygenistein,Citation24) and hydroxyglyciteinCitation25)), and one soyasaponinCitation26) (soyasaponin 1) were identified by comparison to an in-house libraryCitation27) and the published literature (Table ). Of the three hydroxyisoflavones identified, only 8-hydroxydaidzein has been reported in the A. oryzae KACC 40247 strain,Citation28) but Chang et al. and Hirota et al. have reported 8-hydroxygenisteinCitation8), 6-hydroxydaidzein, and 8-hydroxyglyciteinCitation25) as products of A. oryzae fermentation. In our study, it was clear that hydroxygenistein and hydroxyglycitein were produced in the soybean extracts fermented by A. oryzae KACC 40247, and the metabolite was identified based on MS/MS fragmentation. The location of a hydroxyl group in hydroxyglycitein and hydroxygenistein was unclear based on the fragmentation pattern, and we did not determine structure of hydroxyl group. The structures of these isoflavones are presented in Fig. , which shows changes in the levels of each of the secondary metabolites during the fermentation time course.
Table 2. Secondary metabolites displaying significant variation in levels during the fermentation of soybean extracts by A. oryzae
Fig. 2. Changes in isoflavone derivatives during soybean fermentation determined by LC-ESI-MS Analysis.
Note: The chemical structures of the isoflavones are shown (right), and the relative levels of secondary metabolites are shown by box and whisker plots (left).
*The location of a hydroxyl group in hydroxyglycitein and hydroxygenistein was unclear.
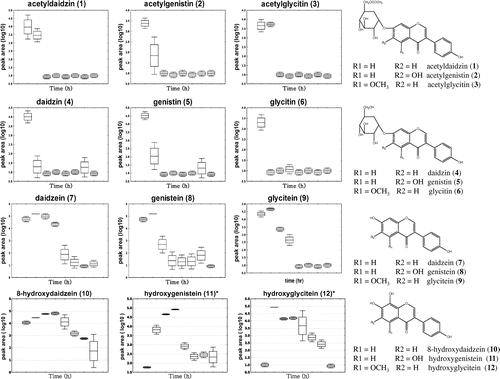
Biotransformation of soybean isoflavones
The time-dependent changes in the secondary metabolites are presented in Fig. . They indicate that the isoflavones changed from acetylglycoside and glycoside to aglycone. In the PCA score plots shown in Fig. (B), 0 and 12 h samples are slightly overlapping because the hydrolysis of glycoside to aglycone took place for the first 12 h after inoculation, and therefore the acetylglycoside and glycoside levels decreased during this initial period of fermentation. Hence, the aglycone levels increased for the first 12 h of fermentation, and then gradually decreased. Increase in the aglycone of soy can be enhanced by the incorporation of β-glucosidase from A. oryzae,Citation3) and β-glucosidase is regarded as the main catalyst mediating isoflavone conversion in fermented soybean foods.Citation29) In general, acetylglycoside is hydrolyzed to glycoside or aglycone by enzymes, 4 h time point during fermentation, the levels of the hydroxyisoflavones were high compared to those at other the time points. Detection of the three hydroxyisoflavones, 8-hydroxydaidzein, hydroxygenistein, and hydroxyglycitein, proves that biotransformation of isoflavones occurred during fermentation. This suggests that metabolite profiling of A. oryzae-fermented soybean using LC-ESI-MS demonstrates sequential isoflavone transformation over the fermentation time course
Antioxidant activity and its correlation with metabolites
In order to determine the antioxidant activity of soybean extract fermented with A. oryzae, an ABTS assay was applied. The results revealed that the antioxidant activity in the extract changed throughout the fermentation time course, showing increases up to 24 h, followed by decreases beyond this time point (Fig. (A)). These trends might be explained by the changes in secondary metabolites that occurred over the fermentation time course, particularly the transformation of aglycone to hydroxyisoflavone, which enhances antioxidant properties.
Fig. 3. Antioxidant activities of fermented soybean extracts.
Note: Fermented soybean extracts were assessed for antioxidant capacities as determined by ABTS assay (A) and correlations between various metabolites and antioxidant activity (B) at various time points across the fermentation time course. In A, the different letters above the bars in the chart indicate that the mean values are significantly different, as determined by Duncan’s multiple range test (p < 0.05). In B, each square indicates r (Pearson’s correlation analysis for a pair of metabolites or antioxidant activities). *R represents a highly positively correlation at R > 0.9 and a negative correlation at R > –0.9.
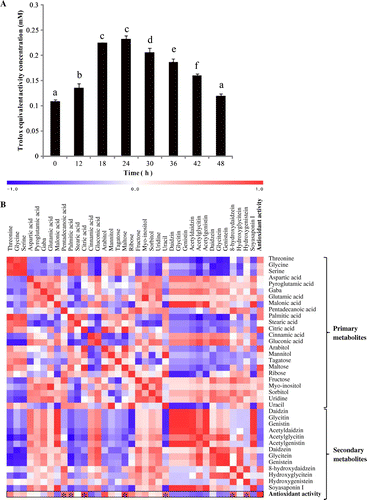
In order to establish a relationship between metabolites and antioxidant activity, a correlation heat map was produced using data-sets from the 23 primary metabolites and the 13 secondary metabolites, and their antioxidant activities (Fig. (B)). The results revealed that the levels of nine metabolites (pentadecanoic acid, palmitic acid, citric acid, arabitol, maltose, uracil, acetylglycitin, 8-hydroxydaidzein, and hydroxygenistein) showed positive correlations (0.9 > r > –0.9) with antioxidant activity. Antioxidant activities of isoflavones have been reported in several studies.Citation30Citation−Citation32) In the present study, among the secondary metabolites, hydroxyisoflavones showed highly positive correlations with antioxidant activity as observed using the metabolic correlation heat map. Thus, it is likely that biotransformation of soybean isoflavones improves the nutraceutical potential of soy-based food materials. In addition, these results suggest that the primary metabolites also exhibited strong antioxidant activity. Examples include the fatty acids, pentadecanoic acid and palmitic acid.Citation33) Citric acid, maltose, glucose, and uracil were also positively correlated with antioxidant activity, in accord with earlier reports of the antioxidant potential of these metabolites.Citation34Citation−Citation36) Our study suggests that arabitol positively correlated with antioxidant activity, but this has not been reported previously and arabitol did not show antioxidant activity in our experiment (data not shown). It was related only to the primary metabolism of A. oryzae. Threonine, glycine, serine, glutamic acid, ribose, fructose, and myo-inositol also showed positive correlations with antioxidant activity according to the heat map but they were not correlated based on the r value (–0.9 < r < 0.9), and therefore these metabolites were not considered to have significant antioxidant potential. They might have shown positive correlations with antioxidant activity due to their metabolism by A. oryzae in the soybean extract. Conversely, daidzin, glycitin, genistin, daidzein, glycitein, and genistein all showed negative correlations with antioxidant activity, although they are known for their activity against free radicals. This is because the antioxidant activity of the hydroxyisoflavones was highly correlated with scavenging activity and its values are higher than that of the other isoflavones, reflecting negative correlation in case of theirs.
In conclusion, our findings suggest that soybean isoflavones are biotransformed to hydroxyisoflavones during fermentation by A. oryzae. During the fermentation process, the levels of primary metabolites were also found to vary significantly. Because the changes in primary and secondary metabolites (particularly the hydroxyisoflavones) correlated with antioxidant potential, as clearly shown by correlation heat map analysis, we believe that biotransformed soy food, in addition to being a source of nutrition, may provide important health benefits as well.
Funding
This research was supported by a grant from the Eco-Innovation project of the Ministry of Environment, Korea [Grant number 416-111-006].
References
- Anderson RL, Wolf WJ. Compositional changes in trypsin inhibitors, phytic acid, saponins and isoflavones related to soybean processing. J. Nutr. 1995;125:581S–588S.
- Hayashi K, Noguchi N, Niki E. Action of nitric oxide as an antioxidant against oxidation of soybean phosphatidylcholine liposomal membranes. FEBS Lett. 1995;370:37–40.
- Chiou RY, Cheng SL. Isoflavone transformation during soybean koji preparation and subsequent miso fermentation supplemented with ethanol and NaCl. J. Agric. Food Chem. 2001;49:3656–3660.
- Yao LH, Jiang YM, Shi J, Tomas Barberan FA, Datta N, Singanusong R, Chen SS. Flavonoids in food and their health benefits. Plant Foods Hum. Nutr. 2004;59:113–122.
- Kishida T, Ataki H, Takebe M, Ebihara K. Soybean meal fermented by Aspergillus awanori increases the cytochrome P-450 content of the liver microsomes of mice. J. Agric. Food Chem. 2000;48:1367–1372.
- Barbesgaard P, HeldtHansen HP, Diderichsen B. On the safety of Aspergillus oryzae :a review. Appl. Microbiol. Biotechnol. 1992;36:569–572.
- Baek JG, Shim SM, Kwon DY, Choi HK, Lee CH, Kim YS. Metabolite profiling of Cheonggukjang, a fermented soybean paste, inoculated with various Bacillus strains during fermentation. Biosci. Biotechnol. Biochem. 2010;74:1860–1868.
- Chang TS, Ding HY, Tai SS, Wu CY. Metabolism of the soy isoflavones daidzein and genistein by fungi used in the preparation of various fermented soybean foods. Biosci. Biotechnol. Biochem. 2007;71:1330–1333.
- Malencic D, Maksimovic Z, Popovic M, Miladinovic J. Polyphenol contents and antioxidant activity of soybean seed extracts. Bioresour. Technol. 2008;99:6688–6691.
- Joubert E, Winterton P, Britz TJ, Gelderblom WC. Antioxidant and pro-oxidant activities of aqueous extracts and crude polyphenolic fractions of rooibos (Aspalathus linearis). J. Agric. Food Chem. 2005;53:10260–10267.
- Kumar V, Rani A, Dixit AK, Bhatnagar D, Chauhan GS. Relative changes in tocopherols, isoflavones, total phenolic content, and antioxidative activity in soybean seeds at different reproductive stages. J. Agric. Food Chem. 2009;57:2705–2710.
- Kim J, Choi JN, Kang D, Son GH, Kim YS, Choi HK, Kwon DY, Lee CH. Correlation between antioxidative activities and metabolite changes during Cheonggukjang fermentation. Biosci. Biotechnol. Biochem. 2011;75:732–739.
- Kim YM, Lee J, Park SH, Lee C, Lee JW, Lee D, Kim N, Kim HY, Lee D. LC–MS-based chemotaxonomic classification of wild-type Lespedeza sp. and its correlation with genotype. Plant Cell Rep. 2012;31:2085–2097.
- Shyur LF, Yang NS. Metabolomics for phytomedicine research and drug development. Curr. Opin. Chem. Biol. 2008;12:66–71.
- Cevallos-Cevallos JM, Reyes-De-Corcuera JI. Metabolomics in food science. Adv. Food Nutr. Res. 2012;67:1–24.
- Setchell KD, Brown NM, Zimmer-Nechemias L, Brashear WT, Wolfe BE, Kirschner AS, Heubi JE. Evidence for lack of absorption of soy isoflavone glycosides in humans, supporting the crucial role of intestinal metabolism for bioavailability. Am. J. Clin. Nutr. 2002;76:447–453.
- Hu SC, Hong K, Song YC, Liu JY, Tan RX. Biotransformation of soybean isoflavones by a marine Streptomyces sp. 060524 and cytotoxicity of the products. World J. Microbiol. Biotechnol. 2009;25:115–121.
- Re R, Pellegrini N, Proteggente A, Pannala A, Yang M, Rice-Evans C. Antioxidant activity applying an improved ABTS radical cation decolorization assay. Free Radic. Biol. Med. 1999;26:1231–1237.
- Frias J, Song YS, Martinez-Villaluenga C, Gonzalez de Mejia E, Vidal-Valverde C. Immunoreactivity and amino acid content of fermented soybean products. J. Agric. Food Chem. 2008;56:99–105.
- Shekib LA. Nutritional improvement of lentils, chick pea, rice and wheat by natural fermentation. Plant Foods Hum. Nutr. 1994;46:201–205.
- Ibrahim S, Fisher C, El Alaily H, Soliman H, Anwar A. Improvement of the nutritional quality of Egyptian and Sudanese sorghum grains by the addition of phosphates. Br. Poult. Sci. 1988;29:721–728.
- Toida J, Arikawa Y, Kondou K, Fukuzawa M, Sekiguchi J. Purification and characterization of triacylglycerol lipase from Aspergillus oryzae. Biosci. Biotechnol. Biochem. 1998;62:759–763.
- Carlsen M, Nielsen J. Influence of carbon source on α–amylase production by Aspergillus royzae. Appl. Microbiol. Biotechnol. 2001;57:346–349.
- Bursztyka J, Perdu E, Tulliez J, Debrauwer L, Delous G, Canlet C, De Sousa G, Rahmani R, Benfenati E, Cravedi JP. Comparison of genistein metabolism in rats and humans using liver microsomes and hepatocytes. Food Chem. Toxicol. 2008;46:939–948.
- Hirota A, Inaba M, Chen YC, Abe N, Taki S, Yano M, Kawaii S. Isolation of 8-hydroxyglycitein and 6-hydroxydaidzein from soybean miso. Biosci. Biotechnol. Biochem. 2004;68:1372–1374.
- Huhman DV, Berhow MA, Sumner LW. Quantification of saponins in aerial and subterranean tissues of Medicago truncatula. J. Agric. Food Chem. 2005;53:1914–1920.
- Lee JS, Kim DH, Liu KH, Oh TK, Lee CH. Identification of flavonoids using liquid chromatography with electrospray ionization and ion trap tandem mass spectrometry with an MS/MS library. Rapid Commun. Mass Spectrom. 2005;19:3539–3548.
- Seo MH, Kim BN, Kim KR, Lee KW, Lee CH, Oh DK. Production of 8-hydroxydaidzein from soybean extract by Aspergillus oryzae KACC 40247. Biosci. Biotechnol. Biochem. 2013;77:1245–1250.
- Yin LJ, Li LT, Liu H, Saito M, Tatsumi E. Effects of fermentation temperature on the content and composition of Isoflavones and β-glucosidase activity in sufu. Biosci. Biotechnol. Biochem. 2005;69:267–272.
- Rufer CE, Kulling SE. Antioxidant activity of isoflavones and their major metabolites using different in vitro assays. J. Agric. Food Chem. 2006;54:2926–2931.
- Jeon HY, Seo DB, Shin HJ, Lee SJ. Effect of Aspergillus oryzae-challenged germination on soybean isoflavone content and antioxidant activity. J. Agric. Food Chem. 2012;60:2807–2814.
- Tepavčević V, Atanacković M, Miladinović J, Malenčić D, Popović J, Cvejić J. Isoflavone composition, total polyphenolic content, and antioxidant activity in soybeans of different origin. J. Med. Food. 2010;13:657–664.
- Henry GE, Momin RA, Nair MG, Dewitt DL. Antioxidant and cyclooxygenase activities of fatty acids found in food. J. Agric. Food Chem. 2002;50:2231–2234.
- Hraš AR, Hadolin M, Knez Željko, Bauman D. Comparison of antioxidative and synergistic effects of rosemary extract with α-tocopherol, ascorbyl palmitate and citric acid in sunflower oil. Food Chemistry. 2000;71:229–233.
- Morelli R, Russo-Volpe S, Bruno N, Lo Scalzo R. Fenton-dependent damage to carbohydrates: free radical scavenging activity of some simple sugars. J. Agric. Food Chem. 2003;51:7418–7425.
- Akhatova GR, Safarova IV, Gerchikov AY. Antioxidant activity of uracil derivatives. Kinet. Catal. 2011;52:1–5.