Abstract
6-Deoxy-l-glucose, 6-deoxy-l-altrose, and 6-deoxy-l-allose were produced from l-rhamnose with an immobilized enzyme that was partially purified (IE) and an immobilized Escherichia coli recombinant treated with toluene (TT). 6-Deoxy-l-psicose was produced from l-rhamnose by a combination of l-rhamnose isomerase (TT-PsLRhI) and d-tagatose 3-epimerase (TT-PcDTE). The purified 6-deoxy-l-psicose was isomerized to 6-deoxy-l-altrose and 6-deoxy-l-allose with l-arabinose isomerase (TT-EaLAI) and l-ribose isomerase (TT-AcLRI), respectively, and then was epimerized to l-rhamnulose with immobilized d-tagatose 3-epimerase (IE-PcDTE). Following purification, l-rhamnulose was converted to 6-deoxy-l-glucose with d-arabinose isomerase (TT-BpDAI). The equilibrium ratios of 6-deoxy-l-psicose:6-deoxy-l-altrose, 6-deoxy-l-psicose:6-deoxy-l-allose, and l-rhamnulose:6-deoxy-l-glucose were 60:40, 40:60, and 27:73, respectively. The production yields of 6-deoxy-l-glucose, 6-deoxy-l-altrose, and 6-deoxy-l-allose from l-rhamnose were 5.4, 14.6, and 25.1%, respectively. These results indicate that the aldose isomerases used in this study acted on 6-deoxy aldohexoses.
Only seven of all of known monosaccharides are found abundantly in nature, d-glucose, d-fructose, d-galactose, d-mannose, d-xylose, l-arabinose, and d-ribose. These are commonly referred to as natural sugars, whereas the remaining monosaccharides are known as rare sugars, because they represent a group of monosaccharides and their derivatives that exist only rarely in nature. The term rare sugars has been defined by the International Society of Rare Sugar.Citation1)
Rare sugars exhibit many interesting properties for application in the pharmaceutical and food industries. The well-known rare sugar d-psicose brought about improved insulin sensitivity and d-glucose tolerance in type 2 diabetes Otsuka Long-Evans Tokushima Fatty rats.Citation2) Furthermore, it was found to improve the foaming property of egg white protein and the color of cookies.Citation3) d-Tagatose, a particularly important rare sugar, has recently been the subject of considerable interest from the food and pharmaceutical industries because it is considered to be a safe low-calorie material,Citation4,5) and it has consequently found widespread application in the USA as a sweetener. With regard to improving food quality, d-tagatose has been reported to extend the shelf life and improve the food safety of cooked cured ham by preventing the growth of spoilage bacteria such as lactic acid bacteria, as well as pathogenic bacteria such as Escherichia coli O157:H7, Salmonella Typhimurium, Staphylococcus aureus, Bacillus cereus, and Yersinia enterocolitica. Based on these results, d-tagatose is now regularly used in cooked cured ham as a preservative in place of dextrose and corn syrup solids.Citation6)
In contrast, 6-deoxy sugars, such as l-rhamnose and l-fucose, exist at comparatively abundant levels in nature. Deoxy sugars are monosaccharide derivatives in which one or more of the hydroxyl groups of the common monosaccharide have been replaced with a hydrogen atom yielding a variety of deoxy sugars by artificial production. These are important carbohydrates that play roles as potentially active components of natural products. Some 6-deoxy sugars form structural components of a variety of natural products, including antibiotics,Citation7,8) antifungals,Citation9) and anticancer agents.Citation10–12) These sugars generally participate in the molecular recognition of cellular targets by active compounds.Citation13) 6-Deoxy-l-galactose (l-fucose) is known as a major component of fucoidan from brown seaweed. Its polymer exhibits a broad range of interesting biological properties, including anticancer,Citation11,12) antitumor,Citation14) and anticoagulantCitation15) activities. 6-Deoxy-l-mannose (l-rhamnose) plays a major role in the gel formation processes of bacterial polysaccharide (gellan) and deacetylated rhamsan gum in aqueous solution.Citation16,17) 6-Deoxy-l-altrose is a component of Gram-negative pathogen lipopolysaccharides, and most likely plays a role in antigens. 6-Deoxy-d-altrose, which was isolated from the fruiting bodies of the edible mushroom Lactarius lividatus,Citation18) has been used for a long time in Chinese folk medicine for its antitumor and antiviral actvities.Citation19) In addition, 6-deoxy-d-glucose is used to study glucose transport in human erythrocytes infected with the malaria parasite, Plasmodium falciparum.Citation20) 6-Deoxy-6-iodo-d-glucose is used as a tool compound to assess glucose transport, which is of major clinical importance because changes in the glucose transport process occur in a number of different pathologies.Citation21) Based on their interesting properties, deoxy monosaccharides have become compounds of considerable interest. Unfortunately, however, their characteristics and functions have not been investigated to any great extent because of their rarity. For this reason, the development of a methodology allowing for the mass production of deoxy monosaccharides is strongly desired to allow for their properties to be thoroughly evaluated.
Traditionally, rare sugars have been synthesized by chemical reactions, but these processes generally require many steps that occur with low specificity and poor purity profiles, resulting in low yields of the desired products. Furthermore, they often result in the formation of hazardous waste products.Citation22,23) Based on these limitations, a biochemical method using enzymatic reactions represents a potentially environmentally friendly procedure for the biosynthesis of monosaccharides, especially rare sugars. d-Xylose isomerase, which catalyzes the conversion of d-glucose to d-fructose, is widely used with amylases to produce a high-fructose corn syrup sweetener from starch,Citation24) and there are many different enzymes that can catalyze isomerization processes other than d-xylose isomerase. To establish effective procedures for the production of rare sugars, we constructed an Izumoring strategy involving a combination of several microbial enzymes. An Izumoring strategy is a structural framework containing 34 six-carbon monosaccharides and their alditols, 16 five-carbon monosaccharides and their alditols, and 9 four-carbon monosaccharides and their alditols, linked through a series of enzymatic reactions.Citation1,25) Rare sugar biosynthesis is catalyzed by (i) alditol dehydrogenases, which oxidize alditols to ketoses, (ii) aldose reductases, which oxidize alditols to aldoses, (iii) d-tagatose 3-epimerase (DTE), which epimerizes the C-3 positions of ketoses, and (iv) aldose isomerases, which catalyze the isomerization reactions of ketoses and aldoses. It is thus possible to use this strategy for the mass production of rare sugars from abundant and inexpensive sugars such as d-glucose and d-fructose.
The microbial enzymes making up an Izumoring strategy have broad substrate specificity, in that it is possible for one of the enzymes to recognize and catalyze the transformation of multiple monosaccharides. There are two enzymes in which the real substrate is not a common monosaccharide in the Izumoring strategy. One of these is l-rhamnose isomerase (l-RhI), which shows the highest activity in the isomerization between l-rhamnose (6-deoxy-l-mamnose) and l-rhamnulose (6-deoxy-l-fructose), and the other enzyme is l-fucose isomerase, which shows the highest activity in the isomerization between l-fucose (6-deoxy-l-galactose) and l-fuculose (6-deoxy-l-tagatose). These enzymes, however, are active towards common monosaccharides, as in the conversion between d-allose and d-psicose affected by l-rhamnose isomerase.Citation26) Hence, we thought that different sugar isomerases showing high levels of activity towards common monosaccharides can also act on 6-deoxy monosaccharides, and we constructed a deoxy-Izumoring strategy based on the results of previous Izumoring strategies and our own experiences. All 12 of the 5-deoxyhexoses and 10 of the 16 1- and 6-deoxyketohexoses were successfully produced in our laboratory, by a combination of chemical and biotechnological methods.Citation27,28) We are currently investigating whether the d-arabinose isomerase (d-AI), l-arabinose isomerase (l-AI), l-ribose isomerase (l-RI), and DTE produced in our laboratory act on 6-deoxy monosaccharides.
Here, we describe the development of a novel procedure for the synthesis of three 6-deoxy-l-aldohexoses by a combination of enzymatic reactions. To the best of our knowledge, this study represents the first on the production of 6-deoxy-l-allose and 6-deoxy-l-altrose by microbial enzymes. Toluene-treated E. coli, harboring the l-RhI gene of Pseudomonas stutzeri LL172,Citation29) the DTE gene of Pseudomonas cichorii ST-24,Citation30) the d-AI gene of Bacillus pallidus Y25,Citation31) the l-AI gene of Enterobacter aerogenes IK7 (unpublished data), and the l-RI gene of Acinetobacter calcoaceticus LR7C,Citation32) were used as biocatalysts in the production of 6-deoxy-l-glucose, 6-deoxy-l-altrose, and 6-deoxy-l-allose. All of the toluene-treated E. coli cells had recombinant proteins as well as the original host proteins, and effectively functioned in the same way as an immobilized microbe. These 6-deoxy-l-aldohexoses were produced from l-rhamnose via l-rhamnulose and 6-deoxy-l-psicose. The overall procedure for the production of these three deoxy-l-aldohexoses is summarized in Scheme .
Materials and methods
Chemicals
l-Rhamnose, d-arabinose, l-arabinose, and l-ribose were purchased from Sigma (St. Louis, MO). d-Tagatose was prepared in our laboratory by a method described previously.Citation33) Bacto tryptone and Bacto yeast extract were purchased from Becton, Dickinson (Sparks, MD). All other chemicals and culture media used were from Wako Pure Chemical Industry (Osaka) and were of reagent grade.
Bacterial strains and culture conditions
The bacterial strains used in this study were recombinant E. coli JM 109 harboring the l-RhI gene of P. stutzeri LL172, the DTE gene of P. cichorii ST-24, the d-AI gene of B. pallidus Y25, the l-AI gene of E. aerogenes IK7, and the l-RI gene of A. calcoaceticus LR7C. These bacteria were transferred from stock culture to Luria agar (Becton, Dickinson) supplemented with 50 μg/mL of ampicillin, and were grown routinely at 30 °C for 24 h.
Preparation of the toluene-treated E. coli harboring enzyme producing gene
Each recombinant E. coli strain was inoculated in a 500-mL Erlenmeyer flask containing super broth (SB) medium (3.5% w/v Bacto tryptone, 2.0% w/v yeast extract, and 0.5% w/v NaCl) supplemented with 100 μg/mL of ampicillin and incubated at 30°C with shaking at 150 rpm for 12 h. Enzyme expression was induced by 1 mM isopropyl β-d-1-thiogalactoside (IPTG) after the cell culture reached an optical density of 0.5–0.7 at 660 nm, and the culture broth was incubated continuously for a further 4 h. The bacterial cells were then harvested by centrifugation at 10,000 × g over 10 min at 4°C. Each recombinant strain was resuspended in an appropriate buffer: 50 mM sodium phosphate buffer (pH 7.5) for l-RhI and DTE, 50 mM glycine–NaOH (pH 9.0) for d-AI and l-RI, and 50 mM glycine–NaOH (pH 10.0) for l-AI.
As preparation of toluene-treated E. coli harboring an enzyme-producing gene, the washed cells were treated with toluene. A mixture of the cell suspension and toluene (9:1) was shaken vigorously for 15 min and the toluene was removed by centrifugation at 10,000 × g over 10 min at 4°C. The resulting pellet was then washed three times with buffer. Finally, the pellet of toluene-treated E. coli was resuspended with the same buffer and used in the enzymatic reaction.
Preparation of immobilized DTE
E. coli JM 109 harboring the DTE gene was grown in SB medium, and the corresponding cell suspension was prepared by the procedure described above. The resulting cell pellet was washed twice with 10 mM Tris–HCl buffer (pH 7.5) and resuspended in the same buffer. CF-PcDTE was prepared with a Branson Digital Sonifier (Model 1450D, Branson, Dallas, TX). Cell debris was removed by centrifugation at 10,000 × g for 10 min at 4°C. To eliminate any thermolabile-contaminated enzymes from the E. coli, CF-PcDTE supplemented with 10 mM MnCl2 was heated at 50°C for 10 min. The CF-PcDTE supernatant was then collected by centrifugation at 10,000 × g over 10 min at 4°C and then partially purified by the addition of polyethylene glycol #6000 (PEG#6000, final concentration 30% w/v). The resulting precipitate was dissolved in the same buffer and immobilized on Chitopearl beads BCW 2510 (Fuji Spinning, Tokyo) at 4°C by a modified version of a previously reported method,Citation34) providing IE-PcDTE.
Enzyme assay
l-Rhamnose, l-arabinose, l-arabinose, and l-ribose were used as substrates to determine the activities of l-RhI, d-AI, l-AI, and l-RI, respectively. The reaction mixtures consisted of 50 mM of the substrate (50 μL), diluted toluene-treated E. coli harboring each enzyme-producing gene suspended in an appropriate buffer (50 μL), and 10 mM MnCl2 (50 μL). The final volume was adjusted with the same buffer to 500 μL. The reaction mixtures were then incubated for 10 min at 30°C for l-RI, 37°C for l-RhI and l-AI, and 55°C for d-AI. The reactions were stopped by the addition of 50 μL of 10% trichloroacetic acid. Ketose formation was determined by the cysteine-carbazole methodCitation35) and with a visual spectrophotometer (UV-1700 Pharmaspec; Shimadzu, Kyoto). For DTE activity, the reaction mixture consisted of 10% (w/v) d-tagatose (100 μL) and each diluted TT-PcDTE, CF-PcDTE, or PP-PcDTE solution (100 μL). The final volume was brought to 500 μL with the following buffers: 50 mM sodium phosphate buffer (pH 7.5) for TT-PcDTE, and 10 mM Tris–HCl buffer (pH 7.5) for CF-PcDTE and PP-PcDTE. The reaction mixture was incubated in a water bath at 37°C for 10 min and then the reaction was stopped by boiling for 3 min. d-Tagatose and d-sorbose were measured by high-performance liquid chromatography (HPLC). One unit of enzymatic activity was defined as 1 μmol of product formed within 1 min under the conditions described above.
Preparation of 6-deoxy-l-psicose and l-rhamnulose from l-rhamnose
To study the enzymatic equilibrium between l-rhamnose and l-rhamnulose, l-rhamnose (0.5 g) was reacted with 5 mL of TT-PsLRhI suspended in sodium phosphate buffer (50 mM, pH 7.5) and 10 mM MnCl2 in a total volume of 10 mL. The reaction was run in an l-tube and incubated at 37°C with gentle shaking (60 rpm).
Transformation of l-rhamnose to 6-deoxy-l-psicose was effected in a 500-mL Erlenmeyer flask with TT-PsLRhI and TT-PcDTE as biocatalysts. The reaction consisted of l-rhamnose (10 g) and a mixture of TT-PsLRhI and TT-PcDTE in 50 mM sodium phosphate buffer (pH 7.5, 50 mL) with 10 mM MnCl2. The final volume of the reaction mixture was brought to 100 mL with the same buffer. The reaction mixture was incubated at 37°C with gentle shaking (60 rpm).
The purified 6-deoxy-l-psicose was further epimerized to l-rhamnulose with IE-PcDTE. The reaction mixture consisted of 6-deoxy-l-psicose (corresponding to 10 g) and IE-PcDTE (4,500 U) in a total volume of 100 mL of 10 mM Tris–HCl buffer (pH 7.5). It was incubated at 45°C with shaking (100 rpm) until it reached equilibrium.
Production of 6-deoxy-l-glucose
6-Deoxy-l-glucose was produced from purified l-rhamnulose by means of TT-BpDAI activity. The reaction was composed of purified l-rhamnulose (corresponding to 1 g), TT-BpDAI in a 50 mM glycine–NaOH buffer (pH 9.0, 50 mL), and 1 M MnCl2 (0.25 mL). The final volume was brought to 100 mL with the same buffer. The reaction mixture was incubated at 55°C with gentle shaking (60 rpm). After the reaction reached equilibrium, it was stopped by heating in boiling water for 5 min. Following the removal of the cell catalyst, the clear supernatant, containing a mixture of l-rhamnulose and 6-deoxy-l-glucose, was adjusted to pH 7.5 and treated with E. coli, an l-rhamnulose-degrading microbial strain, for substrate elimination.
An E. coli suspension in 100 mM sodium phosphate buffer (pH 7.5, OD660 nm = 40) was mixed with an equal volume of clear TT-BpDAI reaction mixture and this was incubated at 37°C with shaking at 150 rpm for 72 h. The remaining l-rhamnulose was monitored by the cysteine-carbazole methodCitation35) and HPLC. Upon complete consumption of l-rhamnulose, the microbial cells were removed by heating in boiling water for 5 min followed by centrifugation at 10,000 × g for 10 min at 4°C and suction filtration with a 0.22 μm cellulose acetate membrane filter.
Production of 6-deoxy-l-altrose and 6-deoxy-l-allose
6-Deoxy-l-altrose and 6-deoxy-l-allose were produced from the purified 6-deoxy-l-psicose. The reaction mixture consisted of purified 6-deoxy-l-psicose (corresponding to 1 g), TT-EaLAI in a 50 mM glycine–NaOH buffer (pH 10.0) or TT-AcLRI in a 50 mM glycine–NaOH buffer (pH 9.0, 50 mL), and 1 M MnCl2 (0.25 mL). The final volume was brought to 100 mL with the same buffer. The reaction mixture was incubated with gentle shaking (60 rpm) at 37 and 30°C for TT-EaLAI and TT-AcLRI, respectively.
Separation and purification of the products
After the reaction reached equilibrium, the toluene-treated recombinant cells were removed by centrifugation at 10,000 × g over 10 min at 4°C. The immobilized enzyme was removed by filtration, and the supernatant was treated with activated charcoal for decolorization and centrifuged at 10,000 × g for 30 min at 4°C. The activated charcoal was removed by filtration. The filtrate was deionized with a mixture of SK1B (H+ form; Mitsubishi Chemical, Tokyo) and Amberlite IRA-411 ( form; Organo, Tokyo) ion exchange resins. The deionized solution was then evaporated and concentrated, resulting in 10–30% Brix of solution, which was applied to a column of DOWEX 50 W-X2 (Ca2+ form; Dow Chemical, Midland, MI). The column was eluted with ultrapure water, and 20 mL fractions were collected. The amount of reducing sugar in each fraction was determined with the Somogyi–Nelson method.Citation36,37) Fractions containing product were evaporated until they reached a suitable concentration for the following step. The production yield was calculated by the following equation:
Identification of the products
The purified products were identified by NMR by an ALPHA 600 spectrometer (JEOL, Tokyo) at 600 MHz in D2O with 0.1 M trimethylsilyl propionic acid as internal reference. The specific optical rotations of the products were recorded on a Jasco R1030 polarimeter (Na lamp; Jasco, Tokyo) at 30°C in deionized H2O at a path length of 10 dm.
Analytical methods
Unless otherwise stated, all of the deoxy sugars were analyzed by HPLC (Hitachi Gl-611 column, Tokyo, and Shimadzu RId-10A refractive index detector, Kyoto), and eluted with ultrapure water containing 0.1 mM NaOH at a flow rate of 1.0 mL/min at 60°C. The ketoses were analyzed by the cysteine-carbazole method,Citation35) and the reducing sugars were analyzed by the Somogyi–Nelson method.Citation36,37)
Results and discussion
Preparation of 6-deoxy-l-psicose and l-rhamnulose from l-rhamnose
TT-PsLRhI (63.72 U/mL) and TT-PcDTE (80.01 U/mL) were prepared by the procedure described above. l-Rhamnose was used as starting substrate in the mass production of 6-deoxy-l-psicose because it is one of the few 6-deoxy monosaccharides that is commercially available. When 5% (w/v) l-rhamnose was isomerized by TT-PsLRhI in a buffer (pH 7.5) at 37°C, the l-rhamnose to l-rhamnulose equilibrium ratio was 60:40 (Fig. (A)). We have reported that purified l-RhI from P. stutzeri LL172 showed an estimated ratio of l-rhamnose to l-rhamnulose of 55:45 in a glycine–NaOH buffer (pH 9.0) at 30°C.Citation29) This difference was attributed to the temperature and the pH conditions of the reaction, because the equilibrium point can be influenced by both of these factors.
Fig. 1. Conversion of l-Rhamnose to l-Rhamnulose by TT-PsLRhI (A), conversion of l-Rhamnose and l-Rhamnulose to 6-Deoxy-l-psicose by a combination of TT-PsLRhI and TT-PcDTE (B), and conversion of l-Rhamnulose to 6-Deoxy-l-psicose by IE-PcDTE (C).
Note: Symbols – ●, l-rhamnose; ▲, l-rhamnulose; ■, 6-deoxy-l-psicose. All experiments were carried out in triplicate. Error bars represent standard deviations from the mean.
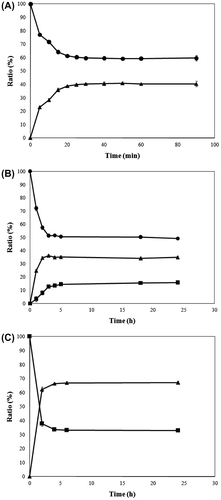
6-Deoxy-l-psicose was produced from 10% (w/v) l-rhamnose by one-pot reaction at a pH of 7.5 and a temperature of 37°C with TT-PsLRhI and TT-PcDTE. The bioconversion rate of l-rhamnulose increased rapidly during the first hour, while the amount of 6-deoxy-l-psicose increased gradually (Fig. (B)). The reaction reached equilibrium at 5 h at an l-rhamnose to l-rhamnulose to 6-deoxy-l-psicose ratio of 50:35:15. Subsequent purification gave 6-deoxy-l-psicose at a production yield of 8.7%. Although the substrate of 6-deoxy-l-psicose is l-rhamnulose, pure l-rhamnulose was not obtained due to the difficulties encountered during the separation of l-rhamnose from l-rhamnulose. According to the HPLC chromatogram of the TT-PsLRhI reaction, the retention times of l-rhamnose and l-rhamnulose were very similar (16.83 and 16.22 min, respectively, data not shown). On the basis of the data, a coupling reaction was selected for the preparation of pure l-rhamnulose. 6-Deoxy-l-psicose was readily purified by DOWEX 50 W-X2 column chromatography, because there was a significant difference between its retention time (27.8 min) and those of l-rhamnose and l-rhamnulose. TT-PsLRhI was found to be active under neutral conditions (pH 7.5), the same as those for optimal DTE.Citation38) Although this procedure allowed for an acceptable conversion of 15% of 6-deoxy-l-psicose, a pH of 9.0 has been reported to be optimal for l-RhI.Citation29)
l-Rhamnulose was prepared from purified 6-deoxy-l-psicose by means of DTE activity to estimate the equilibrium point between l-rhamnulose:6-deoxy-l-psicose and to determine the level of 6-deoxy-l-glucose production, as described below. IE-PcDTE was used instead of TT-PcDTE in this particular preparation to eliminate any contaminated d-AI that might have stemmed from the E. coli cells. Although the use of toluene during the preparation of the enzyme used in this study led to the formation of pores in the cell membranes due to its lipid-dissolving character, no cell lysis or isomerase damage was observed,Citation39,40) and the cells functioned like immobilized microbes, entrapping most of the enzymes in them. Low-molecular-weight substrates such as monosaccharides were taken through the porous cell wall, and the products resulting from their enzymatic reaction were discharged to the outside of the cell when they were not used by the immobilized microbes. l-Rhamnulose was produced from 6-deoxy-l-psicose by TT-PcDTE. Unfortunately, the reaction mixture was contaminated with 6-deoxy-l-glucose, which indicates that d-AI from E. coli was acted on l-rhamnulose. All attempts to separate l-rhamnulose and 6-deoxy-l-glucose by chromatography were unsuccessful, indicating that this reaction did not provide the correct equilibrium ratio between l-rhamnulose and 6-deoxy-l-psicose with TT-PcDTE.
In the current study, IE-PcDTE epimerized 10% (w/v) 6-deoxy-l-psicose under neutral conditions (pH 7.5) at 45°C, and the reaction exhibited an equilibrium ratio of 67:33 between l-rhamnulose and 6-deoxy-l-psicose at 24 h (Fig. (C)). l-Rhamnulose was obtained at a production yield of 44% without any byproduct formation following purification by DOWEX 50 W-X2 column chromatography. The equilibrium ratio of l-rhamnulose to 6-deoxy-l-psicose was biased towards trans-configuration at the C-3 and C-4 positions. This is in accord with the results of a previous study.Citation38) The equilibrium ratios of d-sorbose to d-tagatose, d-fructose to d-psicose, d-xylulose to d-ribulose, and l-xylulose to l-ribulose were 70:30, 80:20, 85:15, and 70:30, respectively.Citation38) DTE also exhibited a similar ratio (75:25) between 6-deoxy-d-fructose (d-rhamnulose) and 6-deoxy-d-psicose (unpublished data).
Production of 6-deoxy-l-glucose
6-Deoxy-l-glucose was synthesized from purified 1% (w/v) l-rhamnulose by TT-BpDAI activity (2.02 U/mL) under alkaline conditions (pH 9.0) at 55°C. Initially, a peak corresponding to 6-deoxy-l-glucose was not detected by HPLC analysis due to the similarity in the retention times of the materials. Any decrease in the amount of l-rhamnulose was hence determined by the cysteine-carbazole method, which allowed for increases in the amount of 6-deoxy-l-glucose to be calculated. TT-BpDAI exhibited an equilibrium ratio between l-rhamnulose and 6-deoxy-l-glucose of 27:73 at 120 h (Fig. ), revealing that the ratio was biased toward deoxy-aldose and that the use of BpDAI was particularly advantageous for the production of 6-deoxy-l-glucose from l-rhamnulose. In contrast, d-AI from Klebsiella pneumoniae 40bXX (KpDAI), previously used in our laboratory, gave common l-glucose from l-fructose at a ratio of 35:65,Citation41) biased towards the ketose. Although it is well known that the equilibrium ratio of d-glucose to d-fructose is approximately 50:50 at 60°CCitation42) and that the ratios of d-glucose to d-fructose and l-glucose to l-fructose are the same regardless of the D/L form, l-glucose production from l-fructose with KpDAI did not reach the equilibrium point. This result was probably due to low thermostability and low activity towards them. Hence thermostable d-AI from B. pallidus (BpDAI) was cloned and used in the production of 6-deoxy-l-glucose from l-rhamnulose.
Fig. 2. Conversion of l-Rhamnulose to 6-Deoxy-l-glucose by TT-BpDAI.
Note: Symbols – ▲, l-rhamnulose, and □, 6-deoxy-l-glucose. All experiments were carried out in triplicate. Error bars represent standard deviations from the mean.
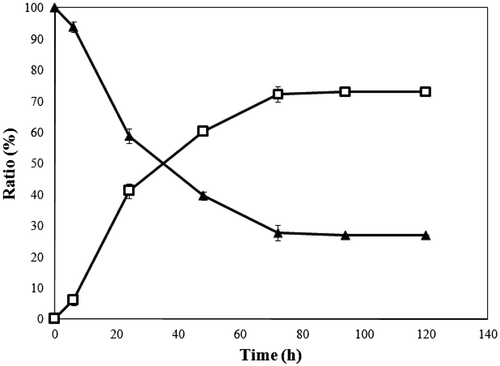
We developed a new method to purify 6-deoxy-l-glucose in a reaction mixture, one that involves elimination of the remaining l-rhamnulose by means of E. coli, as described above in “Materials and Methods.” All of the l-rhamnulose was removed from the mixture with this method, none of the material being observed by HPLC after 48 h (data not shown). The l-rhamnulose taken into the E. coli cells was then metabolized by l-rhamnulose kinase and l-rhamnulose-1-phosphate aldolase.Citation43) The intermediate metabolite, l-lactaldehyde, has been formed and further oxidized to l-lactate with the enzyme lactaldehyde dehydrogenase.Citation43) In contrast, 6-deoxy-l-glucose remained in a reaction mixture treated with E. coli, suggesting that it was not possible for d-AI from E. coli to act on 6-deoxy-l-glucose. Following the degradation of l-rhamnulose, the resulting 6-deoxy-l-glucose solution was sequentially decolorized, deionized, and subjected to HPLC purification to remove any other residual contaminants. Finally, 0.140 g of 6-deoxy-l-glucose was derived from 1 g of l-rhamnulose, at a production yield of 14.0%. The entire procedure from l-rhamnose to 6-deoxy-l-glucose achieved a production yield of 5.4%.
Production of 6-deoxy-l-altrose and 6-deoxy-l-allose
6-Deoxy-l-altrose was synthesized from 1% (w/v) purified 6-deoxy-l-psicose under alkaline conditions (pH 10.0) at 37°C with TT-EaLAI (3.5 U/mL) as biocatalyst. The reaction reached equilibrium at 120 h at a ratio of 6-deoxy-l-psicose to 6-deoxy-l-altrose of 60:40 (Fig. (A)). l-AI from wild-type E. aerogenes IK7 was also found to be capable of converting l-psicose into l-altrose at an equilibrium ratio of 84:16 (data not shown). Recombinant l-AI was used in the current study to convert 6-deoxy-l-psicose to 6-deoxy-l-altrose. By several purification steps, 0.168 g of purified 6-deoxy-l-altrose was obtained from 1 g of 6-deoxy-l-psicose, representing a production yield of 16.8%. The total production yield of 6-deoxy-l-altrose from l-rhamnose was 14.6%.
Fig. 3. Conversion of 6-Deoxy-l-psicose to 6-Deoxy-l-altrose by TT-EaLAI (A), and conversion of 6-Deoxy-l-psicose to 6-Deoxy-l-allose by TT-AcLRI (B).
Note: Symbols – ■, 6-deoxy-l-psicose; ○, 6-deoxy-l-altrose; △, 6-deoxy-l-allose. All experiments were carried out in triplicate. Error bars represent standard deviations from the mean.
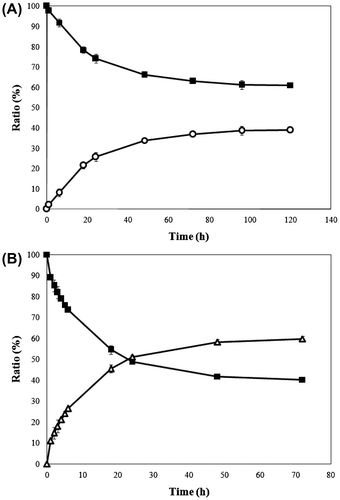
Scheme 1. Overall procedures for the production of 6-Deoxy-l-glucose, 6-Deoxy-l-altrose, and 6-Deoxy-l-allose.
Note: 6-Deoxy-l-psicose was prepared in a one-pot reaction by means of TT-PsLRhI and TT-PcDTE activities. Purified 6-deoxy-l-psicose was further epimerized by IE-PcDTE to produce l-rhamnulose. 6-Deoxy-l-glucose was produced from l-rhamnose via l-rhamnulose by TT-BpDAI. 6-Deoxy-l-altrose and 6-deoxy-l-allose were produced from l-rhamnose via 6-deoxy-l-psicose by TT-EaLAI and TTAcLRI, respectively.
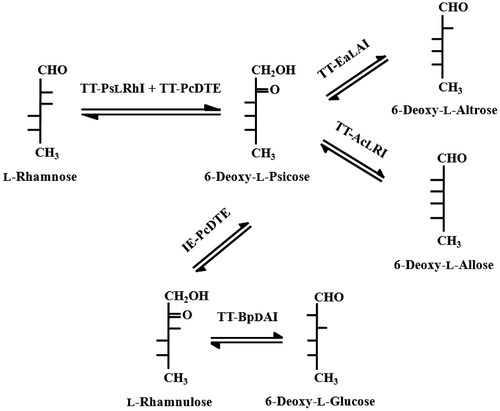
6-Deoxy-l-allose was also produced from 1% (w/v) purified 6-deoxy-l-psicose. The reaction was run under alkaline conditions (pH 9.0) at 30°C with TT-AcLRI (1.39 U/mL) to catalyze isomerization. The reaction reached equilibrium at 72 h at a 6-deoxy-l-psicose to 6-deoxy-l-allose ratio of 40:60 (Fig. (B)). Recombinant l-RI from A. calcoaceticus LR7C was used to convert l-psicose into l-allose at an equilibrium ratio of 70:30 (data not shown), although it showed high activity towards l-ribose. This procedure ultimately provided 0.288 g of purified 6-deoxy-l-allose from 1 g of 6-deoxy-l-psicose, representing a production yield of 28.8%. The total production yield of 6-deoxy-l-allose from l-rhamnose was 25.1%.
These results suggest that the equilibrium ratios achieved in this study for the 6-deoxy-ketoses and 6-deoxy-aldoses were biased towards the 6-deoxy-aldose rather than the 6-deoxy-ketose starting materials, except for 6-deoxy-l-psicose and 6-deoxy-l-altrose. The equilibrium ratios of common aldoses and ketoses resulting from isomerases, however, are generally biased towards the ketoses, except for d-glucose/d-fructoseCitation42) and d-galactose/d-tagatose.Citation44) The equilibrium ratio of 6-deoxy-l-psicose to 6-deoxy-l-altrose was biased towards 6-deoxy-psicose in this study, although the ratio of 6-deoxy-l-altrose was greater than that of the common aldose l-altrose, which was generated from l-psicose (ratio 20:80, data not shown). These observations suggest that 6-deoxy-aldose is favored by isomerization, in contrast to the isomerization reactions between common aldoses and ketoses.
Identification of products
Purified 6-deoxy-l-glucose, 6-deoxy-l-altrose, and 6-deoxy-l-allose were identified on the basis of their Citation13C NMR spectra (Table ). As compared to authentic 6-deoxy-d-glucose, the 6-deoxy-l-glucose generated in the current study gave the same spectrum. Haverkamp et al.Citation45) reported the inclinations of the 13C NMR spectra of 6-deoxy-d-glucose and d-glucose, and they suggested that the C-6 of 6-deoxy-l-glucose underwent a large upfield shift as compared to glucose, and that the C-4 and C-5 of 6-deoxy-d-glucose were shifted slightly downfield and upfield, respectively, as compared to d-glucose. The Citation13C NMR spectra also revealed similar patterns between l-fucose and d-galactose, and between l-rhamnose and d-mannose.Citation45) The specific optical rotations of our synthetic 6-deoxy-l-glucose and authentic 6-deoxy-d-glucose were determined at 30°C with a 2% sugar solution (Table ). Srivastava and LernerCitation46) reported a specific optical rotation of 6-deoxy-d-glucose similar to our result. These results indicate that the monosaccharide resulting from the isomerization of l-rhamnulose by TT-BpDAI was 6-deoxy-l-glucose.
Table 1. 13C NMR spectra of the 6-Deoxy-l-aldohexoses.
Table 2. Specific optical rotations of the 6-deoxy-l-aldohexoses.
Tako et al.Citation18) recently reported detailed 13C, 1H, COSY, and HMQC NMR data for 6-deoxy-d-altrose. A comparison of these data with those of the 6-deoxy-l-altrose generated in this study revealed that the materials were the same. Furthermore, the specific optical rotation of our synthetic 6-deoxy-l-altrose was almost the same as an absolute value reported for 6-deoxy-d-altroseCitation18) (Table ). On the basis of these results, the monosaccharide isomerized from 6-deoxy-l-psicose by TT-EaLAI was identified as 6-deoxy-l-altrose.
The Citation13C NMR spectrum of 6-deoxy-l-allose has not yet been reported, and hence we postulated the nature of this spectrum based on the spectra of the 6-deoxy sugars described above for 6-deoxy-l-glucose. Our analysis indicated that the chemical shifts in 6-deoxy-l-allose and d-allose should show patterns similar to those observed between 6-deoxy-l-glucose and d-glucose. This caused a large upfield shift, the C-4 position of 6-deoxy-l-allose being shifted slightly downfield. The C-5 position of 6-deoxy-l-allose was shifted slightly upfield as compared to d-allose.Citation47) In addition, the COSY and HMQC data strongly indicated that this material was 6-deoxy-allose (data not shown). Araya et al.Citation48) have reported a specific optical rotation of 6-deoxy-d-allose that was entirely consistent with the absolute value for the 6-deoxy-l-allose produced in this study (Table ). These results strongly indicate that the monosaccharide isomerized from 6-deoxy-l-psicose by TT-AcLRI was 6-deoxy-l-allose.
Conclusion
Enzymatic reactions are important in the production of rare sugars, and procedures of this type are considered to be environmentally friendly. Many microbial enzymes have been reported to be biocatalysts for the production of rare sugars.Citation25–32,38,41,42) Here, we report the successful production of three 6-deoxy-aldohexoses, 6-deoxy-l-glucose, 6-deoxy-l-altrose, and 6-deoxy-l-allose, by the use of four aldose isomerases and DTE reactivities. In its entirety, our procedure, which uses l-rhamnose as starting material, resulted in production yields of 5.4, 14.6, and 25.1%, respectively. Although the equilibrium ratios of the various substrates and products were investigated, the kinetic parameters of the substrates corresponding to recombinant DTE, l-RhI, d-AI, l-AI, and l-RI used in this study have not yet been determined. An investigation of the kinetic parameters and the specific activities of the various monosaccharides of these recombinant enzymes might lead to an increase in the general availability of these enzymes and hence to an increase in the number of rare sugars produced.
Funding
This work was supported in part by the Japan Society for the Promotion of Science KAKENHI as follows: a Grant-in-Aid for Scientific Research (C) and a Grant-in-Aid for Young Scientists (B).
Notes
Abbreviations: TT, toluene-treated Escherichia coli recombinants; TT-PsLRhI, toluene-treated Escherichia coli JM 109 harboring the l-rhamnose isomerase (l-RhI) gene of Pseudomonas stutzeri LL172; TT-PcDTE, toluene-treated Escherichia coli JM 109 harboring the d-tagatose 3-epimerase (DTE) gene of Pseudomonas cichorii ST-24; CF-PcDTE, cell-free extract of Pseudomonas cichorii ST-24 DTE; PP-PcDTE, partially purified Pseudomonas cichorii ST-24 DTE by PEG#6000 precipitation; IE-PcDTE, immobilized Pseudomonas cichorii ST-24 DTE; TT-BpDAI, toluene-treated Escherichia coli JM 109 harboring the d-arabinose isomerase (d-AI) gene of Bacillus pallidus Y25; TT-EaLAI, toluene-treated E. coli JM 109 harboring the l-arabinose isomerase (l-AI) gene of Enterobacter aerogenes IK7; TT-AcLRI, toluene-treated Escherichia coli JM 109 harboring the l-ribose isomerase (l-RI) gene of Acinetobacter calcoaceticus LR7C.
References
- Granström TB, Takata G, Tokuda M, Izumori K. J. Biosci. Bioeng. 2004;97:89–94.
- Hossain MA, Kitagaki S, Nakano D, Nishiyama A, Funamoto Y, et al. Biochem. Biophys. Res. Commun. 2011;405:7–12.10.1016/j.bbrc.2010.12.091
- Sun Y, Hayakawa S, Ogawa M, Fukada K, Izumori K. J. Agric. Food Chem. 2008;56:4789–4796.10.1021/jf800050d
- Lee DW, Jang HJ, Choe EA, Kim BC, Lee SJ, Kim SB. Appl. Environ. Microbiol. 2004;70:1397–1404.10.1128/AEM.70.3.1397-1404.2004
- Livesey G, Brown JC. J. Nutr. 1996;126:1601–1609.
- Bautista DA, Pegg RB, Shand PJ. J. Food Protect. 2000;63:71–77.
- Liu HW, Thorson JS. Annu. Rev. Microbiol. 1994;48:223–256.10.1146/annurev.mi.48.100194.001255
- Rodrı́guez L, Aguirrezabalaga I, Allende N, Braña AF, Méndez C, Salas JA. Chem. Biol. 2002;9:721–729.10.1016/S1074-5521(02)00154-0
- Kim WG, Yoon TM, Kwon HJ, Suh JW. J. Antibiot. 2006;59:640–645.10.1038/ja.2006.85
- Zhang G, Fang L, Zhu L, Aimiuwu JE, Shen J, et al. J. Med. Chem. 2005;48:5269–5278.10.1021/jm050144u
- Aisa Y, Miyakawa Y, Nakazato T, Shibata H, Saito K, Ikeda Y, Kizaki M. Am. J. Hematol. 2005;78:7–14.10.1002/(ISSN)1096-8652
- Teruya T, Konishi T, Uechi S, Tamaki H, Tako M. Int. J. Biol. Macromol. 2007;41:221–226.10.1016/j.ijbiomac.2007.02.010
- Weymouth-Wilson AC. Nat. Prod. Rep. 1977;14:99–110.
- Itoh H, Noda H, Amana H, Zhuang C, Mizuno T, Ito H. Anticancer Res. 1993;13:2045–2052.
- Nishino T, Aizu Y, Nagumo T. Agric. Biol. Chem. 1991;55:791–796.10.1271/bbb1961.55.791
- Tako M, Tohma S, Taira T, Ishihara M. Carbohydr. Polym. 2003;54:279–285.10.1016/S0144-8617(03)00029-8
- Tako M, Teruya T, Tamaki Y, Konishi T. Colloid Polym. Sci. 2009;287:1445–1454.10.1007/s00396-009-2112-2
- Tako M, Dobashi Y, Tamaki Y, Konishi T, Yamada M, Ishida H, Kiso M. Carbohydr. Res. 2012;350:25–30.10.1016/j.carres.2011.12.016
- Zhang M, Cui SW, Cheung PCK, Wang Q. Trends Food Sci. Technol. 2007;18:4–19.10.1016/j.tifs.2006.07.013
- Goodyer ID, Hayes DJ, Eisenthal R. Mol. Biochem. Parasitol. 1997;84:229–239.10.1016/S0166-6851(96)02802-2
- Henry C, Tanti JF, Grémeaux T, Morin C, van Obberghen E, Comet M, Le Marchand-Brustel Y. Nucl. Med. Biol. 1997;24:99–104.10.1016/S0969-8051(96)00182-5
- Giffhorn F, Köpper S, Huwig A, Freimund S. Enzyme Microb. Technol. 2000;27:734–742.10.1016/S0141-0229(00)00293-3
- Usvalampi A, Turunen O, Valjakka J, Pastinen O, Leisola M, Nyyssölä A. Enzyme Microb. Technol. 2012;50:71–76.10.1016/j.enzmictec.2011.09.009
- Douglas Crabb W, Mitchinson C. Trends Biotechnol. 1997;15:349–352.10.1016/S0167-7799(97)01082-2
- Izumori K. J. Biotechnol. 2006;124:717–722.10.1016/j.jbiotec.2006.04.016
- Bhuiyan SH, Itami Y, Rokui Y, Katayama T, Izumori K. J. Ferment. Bioeng. 1998;85:539–541.10.1016/S0922-338X(98)80104-9
- Gullapalli P, Yoshihara A, Morimoto K, Rao D, Akimitsu K, Jenkinson SF, Fleet GWJ, Izumori K. Tetrahedron Lett. 2010;51:895–898.10.1016/j.tetlet.2009.12.024
- Rao D, Best D, Yoshihara A, Gullapalli P, Morimoto K, Wormald MR, Wilson FX, Izumori K, Fleet GWJ. Tetrahedron Lett. 2009;50:3559–3563.10.1016/j.tetlet.2009.03.061
- Bhuiyan SH, Itami Y, Izumori K. J. Ferment. Bioeng. 1997;84:319–323.10.1016/S0922-338X(97)89251-3
- Ishida Y, Kamiya Y, Itoh H, Kimura Y, Izumori K. J. Ferment. Bioeng. 1997;83:529–534.
- Takeda K, Yoshida H, Izumori K, Kamitori S. Biochim. Biophys. Acta. 2010;1804:1359–1368.10.1016/j.bbapap.2010.01.018
- Mizanur RMD, Takata G, Izumori K. Biochim. Biophys. Acta. 2001;1521:141–145.10.1016/S0167-4781(01)00290-1
- Muniruzzaman S, Tokunaga H, Izumori K. J. Ferment. Bioeng. 1994;78:145–148.10.1016/0922-338X(94)90253-4
- Leang K, Maekawa K, Menavuvu BT, Morimoto K, Granström TB, Takada G, Izumori K. J. Biosci. Bioeng. 2004;97:383–388.10.1016/S1389-1723(04)70223-6
- Dishe A, Borenfreund E. J. Biol. Chem. 1951;192:583–587.
- Nelson N. J. Biol. Chem. 1944;153:375–380.
- Somogyi M. J. Biol. Chem. 1952;195:19–23.
- Itoh H, Okaya H, Khan AR, Tajima S, Hayakawa S, Izumori K. Biosci. Biotechnol. Biochem. 1994;58:2168–2171.10.1271/bbb.58.2168
- Jackson RW, DeMoss JA. J. Bacteriol. 1965;90:1420–1425.
- Dobrogosz WJ, DeMoss RD. J. Bacteriol. 1963;85:1350–1354.
- Leang K, Sultana I, Takada G, Izumori K. J. Biosci. Bioeng. 2003;95:310–312.10.1016/S1389-1723(03)80036-1
- Lloyd NE, Khaleeluddin K. Cereal Chem. 1976;53:270–282.
- Baldoma L, Aguilar J. J. Bacteriol. 1988;170:416–421.
- Wanarska M, Kur J. Microb. Cell Fact. 2012;11:113.10.1186/1475-2859-11-113
- Haverkamp J, De Bie MJA, Vliegenthart JFG. Carbohydr. Res. 1975;39:201–211.10.1016/S0008-6215(00)86130-3
- Srivastava VK, Lerner LM. Carbohydr. Res. 1977;64:263–265.
- Katayama T, Iwai A, Kobori R, Suzuki T, Izumori K. In: Tajima S, Asada Y, editors. Rare sugars: creating a novel bio-world with rare sugars; International Symposium of Rare Sugar, Kagawa. Miki: Art-Printing Co., Ltd.; 2005. p. 347–350.
- Araya JJ, Kindscher K, Timmermann BN. J. Nat. Prod. 2012;75:400–407.10.1021/np2008076