Abstract
The Mid2-like protein MtlA is a putative sensor of the cell wall integrity (CWI) signaling pathway in Aspergillus nidulans. An MtlA-EGFP fusion protein was localized at the cell surface and septa. The mtlA disruptant (∆mtlA) showed radial colony growth similar to the wild-type (wt) strain, but showed reduced conidia formation. The ∆mtlA mutant showed growth deficiency in the presence of inhibitors of cell wall synthesis. Moreover, mtlA disruption resulted in a reduction in the glucan and chitin content in the cell wall. These results suggest that MtlA plays a significant role in asexual sporulation, cell wall stress tolerance, and the maintenance of CWI in A. nidulans, but transcriptional upregulation of α-1,3-glucan synthase gene agsB induced by micafungin was observed in the ∆mtlA strain as well as the wt strain. Thus, MtlA is not essential for activation of the downstream CWI signaling pathway components identified in previous studies of Saccharomyces cerevisiae.
Graphical Abstract
The MtlA is a putative sensor protein of the cell wall integrity signaling pathway and required for conidia formation and cell wall stress tolerance in Aspergillus nidulan
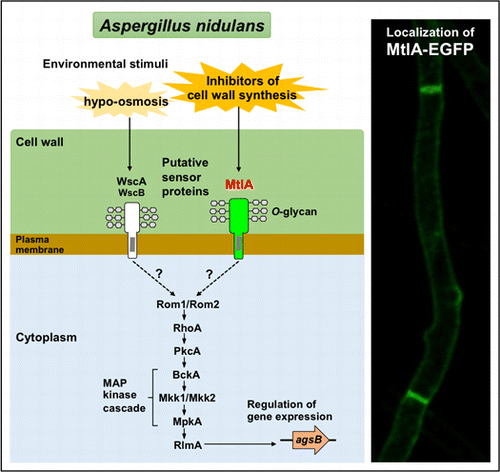
The cell wall integrity (CWI) signaling pathway plays a significant role in the regulation of cell wall biogenesis and has been well-characterized in the baker’s yeast Saccharomyces cerevisiae.Citation1) The components of CWI signaling identified in S. cerevisiae are conserved in fungi such as the Ascomycota, including Aspergillus species. In the model filamentous fungus Aspergillus nidulans, orthologs of signal transducers RhoA, PkcA, and MpkA and transcriptional factor RlmA play essential roles in cellular polarity and differentiation.Citation2–8) For example, disruption of the mpkA gene, which encodes mitogen-activated protein kinase (MAPK), results in increased sensitivity to calcofluor white (CFW) and micafungin, a potent inhibitor of cell wall assembly by binding to chitin and an inhibitor of β-1,3-glucan synthase (FksA), respectively.Citation5,9) Moreover, it has been found that the MpkA-RlmA signaling pathway is required for transcriptional upregulation of the α-1,3-glucan synthase-encoding agsB gene in a response to the cell wall stress associated with micafungin.
The stress sensor proteins associated with CWI signaling have been identified and characterized in S. cerevisiae. They include Wsc1, Wsc2, Wsc3, Mid2, and Mtl1.Citation1) Sensory proteins localized on the cell surface along with stress-activated sensors initiate a signaling cascade involving downstream transducers. In A. nidulans, two Wsc family proteins, WscA and WscB, have been identified and characterized.Citation10) The wscA gene disruptant (ΔwscA) shows hypo-osmotic growth defects and is alkaline pH-sensitive. Through an unknown mechanism, the level of phosphorylated MpkA in the ΔwscA strain is increased, resulting in an increase in the level of agsB transcripts and a consequent increase in the cell wall alkaline-soluble fraction, including α-1,3-glucan. In contrast, the wscB gene disruptant (ΔwscB) shows the same growth defects as the ΔwscA strain, although to a lesser extent, and based on the phenotype of the wscA-wscB double disruptant (ΔwscA ΔwscB), WscB and WscA appear to be functionally redundant. However, unexpectedly, wsc disruptants do not show reduced sensitivity to inhibitors of cell wall biogenesis, including CFW, Congo red, and micafungin, leading us to question what roles WscA and WscB play in CWI signaling.
Recently, three putative sensor proteins, Wsc1, Wsc3, and MidA, were characterized in A. fumigatus.Citation11) It was found that Wsc1 influences sensitivity to caspofungin (an echinocandin class of antifungal agents similar to micafungin), whereas MidA influences sensitivity to heat stress. This is in contrast to the case of A. nidulans, in which the Wsc family proteins are not involved in tolerance of micafungin stress.Citation10)
Through a search for putative O-glycosylated proteins in A. nidulans, we found that Mid2-like protein MtlA, a homolog of S. cerevisiae Mid2 and A. fumigatus MidA, is a putative sensor in CWI signaling.Citation12) Hence in the present work, we characterized MtlA in order to clarify its role in maintaining A. nidulans CWI. Our results indicate that MtlA is a highly O-glycosylated protein localized on the cell surface and septa. Disruption of mtlA led to a reduction in glucan and chitin levels in the cell wall, indicating the MtlA is involved in CWI. In contrast to previously characterized Wsc family proteins, we found that MtlA was involved in the stress tolerance of inhibitors of cell wall component synthesis, including CFW, Congo red, and micafungin. We discuss the role of MtlA in comparison to orthologous proteins in S. cerevisiae and A. fumigatus.
Materials and methods
A. nidulans strains, media, and culture conditions
The A. nidulans strains used in this study (Table ) were grown in YG medium (0.5% yeast extract and 1% glucose) with or without 0.6 M KCl or in minimal medium (MM) (1% glucose, 0.6% NaNO3, 0.052% KCl, 0.052% MgSO4·7H2O, 0.152% KH2PO4, 0.211% arginine, 5 μg/mL of biotin, and Hunter’s trace elements, pH 6.5). The media were adjusted to the required pH with NaOH. To express genes under the control of the alcA promoter, 0.1 M threonine, and 0.1% fructose were added to MM as carbon sources instead of glucose. Antifungal agents were purchased from the following companies: micafungin (Funguard), Astellas Pharma (Tokyo); Congo Red, Nakalai Tesque (Kyoto, Japan); fluorescent brightener 28 (CFW), Sigma–Aldrich (St. Louis, MO).
Table 1. Aspergillus nidulans strains used in this study.
Construction of the mtlA-disruptant strain
A. nidulans AKU89Citation13) was ectopically complemented with the A. nidulans ornithine carbamoyltransferase-encoding argB gene and used as the wt strain in this study. The mtlA gene was disrupted in strain AKU89 by insertion of the argB gene. The gene replacement cassette, encompassing 1.0 kb of the 5′ end of mtlA, 2 kb of argB, and 1.0 kb of the 3′ end of mtlA, was constructed by recombinant PCR with primer sets p4897F/p4897-argB-R, p4897-argB-F/argB-t4897-R, and argB-t4897-F/t4897R, respectively (Table S1 and Fig. S1A; see Biosci. Biotechnol. Biochem. Web site). The resulting DNA fragment amplified with primers nested-p4897-F and nested-t4897-R was used to transform strain AKU89. A standard transformation method for A. nidulans was used.Citation14) The transformants were selected on MM agar plates without arginine. Introduction of the argB gene into the mtlA locus was confirmed by PCR with primer pair mtlA-FC/mtlA-RC (Table S1 and Fig. S1C).
Construction of the MtlA-EGFP-expressing mtlA-disruptant strain
For complementation testing and localization and glycosylation studies, MtlA fused with EGFP via a 5×(GA) linker was expressed in the ΔmtlA strain. The Gateway cloning system (Invitrogen, Carlsbad, CA) with a pMT-OvE plasmid vectorCitation15) was used to construct the MtlA-EGFP-expressing mtlA-disruptant strain (ΔmtlA::mtlA-EGFP) under the control of the alcA promoter. Because the pMT-OvE vector encodes the argB gene (which was used in the disruption of mtlA, as described above) as selectable marker for transformation, the mtlA gene in strain AKU89 was disrupted again by insertion of the pyrithiamine resistance gene (ptrA). The gene replacement cassette, encompassing 1.2 kb of the 5′ end of mtlA, 2 kb of ptrA, and 1.1 kb of the 3′ end of mtlA, was constructed by recombinant PCR with primer pairs mtlA-FC/mtlA-R1, mtlA-F2/mtlA-R2, and mtlA-F3/mtlA-RC, respectively (Table S1 and Fig. S1B). The resulting DNA fragment amplified with primers mtlA-F1 and mtlA-R3 was used to transform strain AKU89. In the selection of transformants, MM agar plate supplemented with 0.1 μg/mL of pyrithiamine was used. Introduction of the ptrA gene into the mtlA locus was confirmed by PCR with primer pair mtlA-FC/mtlA-RC (Table S1 and Fig. S1C). The mtlA disruptant constructed by ptrA replacement was confirmed to show a growth defect phenotype similar to the mtlA disruptant constructed by argB insertion.
The gene cassette encompassing 2 kb of mtlA with the 5′-untranslated region and 0.7 kb of the EGFP gene was constructed by recombinant PCR with primer pairs mtlA-FC/mtlA-gfp-R1 and mtlA-gfp-F2/mtlA-gfp-R2, respectively (Table S1). For amplification of the EGFP gene, pEGFP (Clontech, Mountain View, CA) was used as template. The resulting DNA fragment, including mtlA fused with the EGFP gene, was amplified with the mtlA-gfp-ENT-F and mtlA-gfp-ENT-R primer set and cloned into plasmid pENTR/D-TOPO (Invitrogen) following the manufacturer’s protocol. The inserted DNA was then transferred into pMT-OvE using the Gateway LR Clonase II enzyme mix (Invitrogen). The constructed pMT-OvE carrying mtlA-EGFP was used to transform the mtlA disruptant, which was constructed by ptrA insertion.
Microscopy
To observe the localization of MtlA protein fused with EGFP, we inoculated 2 × 107 conidia of ΔmtlA::mtlA-EGFP into 100 mL of a medium that induces the alcA promoter, and incubated the culture at 30 °C for 18 h with shaking at 140 rpm. The mycelia were mounted on glass slides and observed by a FluoView FV10i confocal laser scanning microscope (Olympus, Tokyo). Images were acquired in Z-stack mode.
Deglycosylation of MtlA-EGFP and immunoblot analysis
A crude extract prepared from the ΔmtlA::mtlA-EGFP strain was treated with trifluoromethanesulfonic acid (TFMS, Sigma Aldrich) as previously described.Citation10,16) The proteins were then subjected to sodium dodecyl sulfate-polyacrylamide gel electrophoresis (SDS-PAGE) and immunoblot analysis with an anti-GFP monoclonal antibody (Roche). ImmunoStar LD chemiluminescence reagent (Wako, Osaka, Japan) was used for detection.
Analysis of conidiation efficiency
Approximately 105 conidia were spotted at the centers of 84-mm agar plates containing YG medium with and without 0.6 M KCl. After 5 d of incubation at 30 °C, the conidia were suspended in 5 mL of 0.01% (wt/vol) Tween 20 solution and counted by a TC10 Automated Cell Counter (Bio-Rad, Hercules, CA). The mean number of conidia and the standard deviation were determined from the results for three plates.
Analysis of cleistothecia formation
To induce cleistothecium development, approximately 105 conidia were dissolved in 5 mL of sterilized 1% agar that was melted and kept at 50 °C and then overlaid on an MM plate. The plastic plate was sealed with vinyl tape and covered with aluminum foil to prevent exposure to oxygen and light. After incubation for 2 weeks at 30 °C, the cleistothecia were retrieved by the addition of 5 mL of water to the surface of the plate and rubbing of the surface by hand with examination gloves. The cleistothecia were observed by an inverted IX70 light microscope (Olympus). The mean number of cleistothecia and the standard deviation were determined from the results for three plates.
Analysis of glucan and chitin levels
Approximately 2 × 107 conidia of the wt and ΔmtlA strains were inoculated into 100 mL of YG medium with and without 2 ng/mL of micafungin and cultivated with shaking at 140 rpm at 30 °C for 18 h or 20 h, respectively. The strains were grown longer in the presence of micafungin than in the absence of it to increase the cell yield. The mycelia were freeze-dried and their weight was determined.
For cell wall extraction, freeze-dried cells were disrupted with metal corn by a multibead shocker (Yasui Kikai, Osaka, Japan) operated at 2,500 rpm for 10 s. The disrupted cells were suspended in 10 mL of ultrapure water and homogenized three times with 2 g of 0.5-mm beads by beating with a multibead shocker operated at 2000 rpm for 4 min, with intervals for refrigeration. The supernatants without glass beads were transferred to new tubes and centrifuged at 3000 g for 10 min. To remove proteins, the resulting pellets were dissolved in buffer (50 mM Tris-HCl, 50 mM ethylenediaminetetraacetic acid, 2% SDS, and 40 mM 2-mercaptoethanol, pH 7.5), boiled for 15 min and centrifuged at 3000 g for 10 min, and then the supernatants were removed.Citation17) The boiling and centrifugation steps were repeated two times. The resulting pellets were dissolved in 10 mL of ultrapure water and centrifuged at 2600 g for 10 min, and the supernatant was removed. The centrifugation and washing steps were repeated six times. The resulting pellets were freeze-dried and used as the cell wall fraction. The weight of the cell wall fraction was determined. Measurements of total glucan, alkali-soluble glucan, alkali-insoluble glucan, and GlcNAc were done using about 5 mg of cell wall, as described previously.Citation10) Glucan and chitin content analyses were done using the phenol-sulfuric acid method and the Morgan–Elson method, respectively.Citation18,19)
Preparation of RNA
For analysis of the transcriptional response of the cell wall synthesis-related genes after micafungin treatment, approximately 2 × 107 conidia from the wt, ΔmtlA, ΔwscA ΔwscB, and ΔmpkA strains were inoculated into 100 mL of YG medium and incubated at 30 °C with shaking at 140 rpm. After 18 h, micafungin was added to a final concentration of 10 ng/mL. Mycelia were collected just before the addition of micafungin and after 30 min of the addition of micafungin, and frozen immediately at −80 °C. Total RNA was extracted by RNAiso (Takara Bio) as previously described.Citation10)
Real-time RT-PCR analysis
cDNAs were synthesized by a PrimeScript Perfect real-time reagent kit (Takara Bio) following the manufacturer’s protocol. Real-time PCR analysis was done by the LightCycler Quick 330 system (Roche, Basel, Switzerland) with SYBR Premix Ex Taq II (Tli RNaseH Plus) (Takara Bio). The following primers were used: agsB-RT-F and agsB-RT-R for agsB; fksA-RT-F and fksA-RT-R for fksA; gelA-RT-F and gelA-RT-R for gelA; gelB-RT-F and gelB-RT-R for gelB; csmA-RT-F and csmA-RT-R for csmA; csmB-RT-F and csmB-RT-R for csmB; chsA-RT-F and chsA-RT-R for chsA; chsB-RT-F and chsB-RT-R for chsB; chsC-RT-F and chsC-RT-R for chsC; chsD-RT-F and chsD-RT-R for chsD; gfaA-RT-F and gfaA-RT-R for gfaA; and histone-RT-F and histone-RT-R for the histone H2B gene (Table S1).Citation5) The histone H2B gene was used to standardize the mRNA levels of the target genes. Three independent experiments were performed from triplicate cultures, and the mean transcriptional copy number and standard deviation for each gene were calculated.
Results
Amino acid sequence of MtlA
For comparison, the amino acid sequence of A. nidulans MtlA was aligned with those of S. cerevisiae Mid2 (ScMid2) and A. fumigatus MidA (AfMidA) (Fig. S2A). MtlA has a putative N-terminal signal sequence, a Mid2 domain, and a putative transmembrane region that is important in localization to the plasma membrane. The domain constitutions of MtlA, AfMidA, and ScMid2 were conserved, but the length of the Mid2 domain of MtlA and AfMidA was shorter than that of ScMid2 (74 aa in MtlA, 78 aa in AfMidA, and 154 aa in ScMid2). Since ScMid2 is highly O-glycosylated,Citation20) MtlA showed high frequencies of Ser (19.19% or 55 of 271 aa) and Thr (22.88% or 62 of 271 aa), comparable to the ScMid2 frequencies of Ser (30.85% or 116 of 376 aa) and Thr (9.57% or 36 of 376 aa).
Effects of mtlA disruption on A. nidulans colony formation
Disruption of mtlA did not affect the radial colony growth rate of A. nidulans on either the YG or the YG + KCl medium at 30, 37, and 42 °C (Fig. S2B). This indicates that MtlA is not required for heat-stress tolerance. In contrast, the efficiency of conidia formation of the ΔmtlA strain was reduced to about 30% and about 12% of that of the wt strain in the presence and the absence of KCl, respectively (Fig. (A)), indicating that MtlA is involved in asexual development.
Fig. 1. Asexual and sexual development.
Note: (A) Formation of conidia on YG agar plates with and without 0.6 M KCl was assessed after 5 d of cultivation at 30 °C. All results are expressed as mean and standard deviation. *Statistically significant difference (p < 0.05, Welch’s t-test) relative to the result for the wt strain. (B) Cleistothecia development in the wt, ΔmtlA, and ΔwscA ΔwscB strains. Bars represent 500 μm. (C) Numbers of cleistothecia in the wt, ΔmtlA, and ΔwscA ΔwscB strains. All results are expressed as mean and standard deviation. Statistically significant difference (p < 0.05, Welch’s t-test) relative to the result for the wt strain.
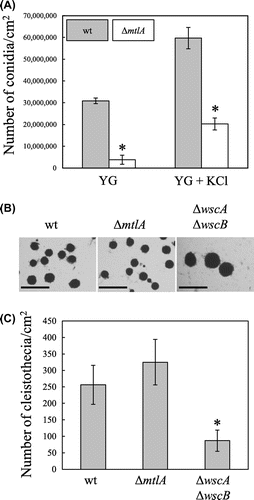
To investigate the role of MtlA in sexual development in A. nidulans, we evaluated the production of cleistothecia (Fig. (B) and (C)). Because the function of Wsc family proteins in sexual development has not been characterized, we also examined the ΔwscA ΔwscB strain for comparison. The number of cleistothecia was about 1.3-fold higher in the ΔmtlA strain and about 0.34-fold lower in the ΔwscA ΔwscB strain than in the wt strain (Fig. (C)), but the change in the ΔmtlA strain was not statistically significant. The average size of the cleistothecia was negatively correlated with the number of cleistothecia formed (Fig. (B)). The cleistothecia diameters were 202 ± 31, 151 ± 38, and 333 ± 54 μm in the wt, ΔmtlA, and ΔwscA ΔwscB strains, respectively.
Next, we investigated the role of MtlA in stress tolerance of inhibitors of cell wall biogenesis. The ΔmtlA strain was more sensitive to CFW and Congo red (a potent inhibitor of glucan synthesis) than the wt strain (Fig. ). Colony formation by the ΔmtlA strain was completely inhibited by 5 μg/mL of CFW and by 200 μg/mL of Congo red, but these concentrations showed lesser inhibition of the wt strain. This indicates that MtlA is involved in the stress tolerance to these potent inhibitors of chitin assembly and glucan synthesis.
MtlA-EGFP complementation test of the mtlA-disruptant
To determine the localization and glycosylation of MtlA, mtlA-EGFP was expressed in the ΔmtlA strain under the control of the alcA promoter. The function of the MtlA-EGFP fusion was confirmed by complementation testing of the ΔmtlA strain. The ΔmtlA::mtlA-EGFP strain showed reduced conidia formation similar to the ΔmtlA strain on MM under non-inducing conditions for the alcA promoter (Fig. (A)), but this deficiency was partially remedied under the alcA promoter-inducing condition (Fig. (B)). In addition, although the ΔmtlA::mtlA-EGFP strain showed higher sensitivity to Congo red than the wt strain under the alcA promoter-inducing condition, it was less than that of the ΔmtlA strain (Fig. (A)). These results indicate that MtlA-EGFP partially compensates for mtlA disruption.
Fig. 3. MtlA-EGFP complementation test on the ΔmtlA Strain.
Note: (A) Approximately 105 conidia of the wt, ΔmtlA, and ΔmtlA::mtlA-EGFP strains were inoculated on the centers of agar plates and incubated at 30 °C. Expression of the MtlA-EGFP fusion protein was controlled under the alcA promoter, which was repressed in MM and induced in the medium containing fructose (+Frc) and threonine (+Thr) instead of glucose (−Glc). Partial compensation for mtlA-disruption by MtlA-EGFP was confirmed by colony formation in the presence of Congo red. White bars represent 1 cm. (B) Conidia formation efficiencies under the condition of alcA promoter induction. *Statistically significant difference (p < 0.05, Welch’s t-test) relative to the result for the wt strain. #Statistically significant difference (p < 0.05, Welch’s t-test) relative to the result for the ΔmtlA strain.
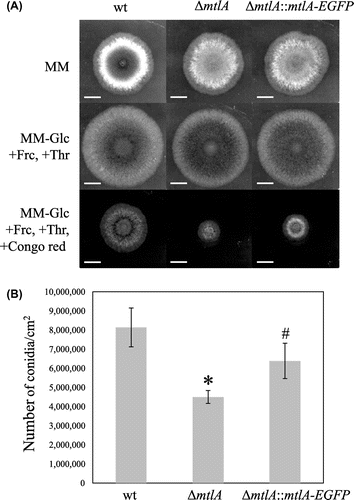
Localization of MtlA-EGFP
The localization of the MtlA-EGFP fusion protein was determined by fluorescence confocal microscopy (Fig. (A)). The fusion protein was detected on the cell surface. This was in agreement with the prediction that MtlA has a signal sequence and one transmembrane domain, and indicates that MtlA functions as a cytoplasmic membrane-spanning sensor. The signal intensity of the hyphal outline was unchanged at the growing hyphal tips, but the MtlA-EGFP fusion protein was also observed in the septa of the ΔmtlA::mtlA-EGFP strain, and the signal intensity of the septa appeared to be higher than that of the hyphal outline.
Fig. 4. O-Glycosylated MtlA-EGFP was localized at the cell surface and septa.
Note: (A) The MtlA-EGFP-expressing mtlA-disruptant (ΔmtlA::mtlA-EGFP) strain was observed by confocal microscopy. White bar represents 10 μm. (B) Cell extracts of the ΔmtlA::mtlA-EGFP strain treated with and without TFMS were subjected to immunoblot analysis by anti-GFP antibody.
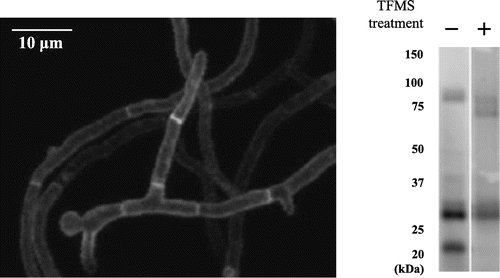
O-Glycosylation of MtlA-EGFP
Because MtlA has a large number of Ser and Thr residues that are predicted O-glycosylation sites, we investigated the molecular mass of MtlA-EGFP. Immunoblot analysis revealed a difference between the observed molecular mass (about 82–92 kDa) and the calculated molecular mass (about 52 kDa) of the MtlA-EGFP fusion protein (Fig. (B)). To determine whether this difference was due to O-glycosylation, we treated the MtlA-EGFP fusion protein with TFMS, which removes both N- and O-glycans, and this resulted in a decrease in the molecular mass of MtlA to about 63 kDa. Because A. nidulans MtlA has no potential N-glycosylation sites (Asn-Xaa-Ser/Thr) (Fig. S1A) and N-glycosidase F treatment yielded no evidence of N-glysocylation of MtlA (data not shown), we conclude that the MtlA protein is only O-glycosylated. In addition, two bands (about 28 and 21 kDa) that were lower than the calculated molecular mass (about 52 kDa) were detected on immunoblot analysis, indicating that MtlA-EGFP is partially degraded by proteolysis in the ΔmtlA strain.
Effect of micafungin on growth
In a previous study, we characterized the Wsc proteins of A. nidulans, and found that the disruption of wsc genes did not cause hypersensitivity to micafungin.Citation10) To further examine the issue of micafungin sensitivity, we compared the radial growth rates of wt, ΔmtlA, and ΔwscA ΔwscB colonies in the absence and the presence of various concentrations of micafungin (Fig. ). The radial growth rates of the wt and ΔmtlA strains were similar in the absence and the presence of 1 ng/mL of micafungin, but at micafungin concentrations of 2, 4, and 8 ng/mL, the radial growth rate of the ΔmtlA strain was lower than that of the wt strain. In contrast, the ΔwscA ΔwscB strain showed a reduced growth rate even in the absence of micafungin, as previously found.Citation10) The growth rate of the ΔwscA ΔwscB strain was reduced in the presence of micafungin in a dose-dependent manner, but the radial growth rate of the ΔwscA ΔwscB strain relative to that of the wt strain increased, so that the growth rates were similar in the presence of 8 ng/mL of micafungin. These results suggest that MtlA plays an important role in the response to micafungin-induced stress, and that this role differs from those of WscA and WscB.
Fig. 5. Comparison of the effects of micafungin on the growth of A. nidulans Wt, ΔmtlA, and ΔwscA ΔwscB Strains.
Note: A total of 104 conidiospores of a given strain were inoculated onto YG plates containing micafungin and cultured at 30 °C. The radial growth rate (cm/d) of each strain was then calculated.
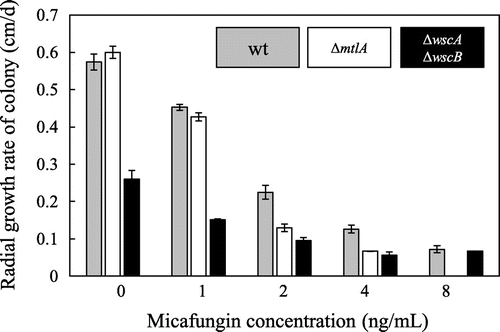
Effect of micafungin on the cell wall
To investigate further whether MtlA is involved in ensuring CWI, we examined the cell walls of the wt and ΔmtlA strains cultivated in the absence and the presence of micafungin, which triggers the signaling cascade that promotes CWI. In the wt strain, the amounts of all cell wall fractions examined increased following the addition of micafungin (Fig. (A)), indicating that the levels of all cell wall glucans increased as a result of the response to micafungin. However, although the amounts of total glucan and the alkali-soluble and insoluble fractions increased in the ΔmtlA strain following the addition of micafungin, the amount of total GlcNAc remained the same regardless of whether the cells were grown in the presence or the absence of micafungin. The alkali-soluble fraction contains α-glucan and soluble β-1,3-/1,6-glucan, whereas the alkali-insoluble fraction contains β-1,3-/1,6-glucan covalently linked to chitin.Citation19,21,22) GlcNAc is a unit of the chitin polymer. This indicates that the response to micafungin-induced stress of the ΔmtlA strain leads to an increase in the amount of cell wall glucan, but not the chitin.
Fig. 6. Comparison of the effects of micafungin on cell wall components and growth in liquid culture.
Note: (A) Cell wall composition of the A. nidulans wt and ΔmtlA strains cultured at 30 °C in YG medium with and without micafungin for 18 h and 20 h, respectively. *Statistically significant difference (p < 0.05, Welch’s t-test) relative to the result obtained in the absence of micafungin. #Statistically significant difference (p < 0.05, Welch’s t-test) relative to the result obtained for the wt strain. B, Mycelia yields of the wt and ΔmtlA strains cultured at 30 °C in YG medium with and without micafungin for 18 h and 20 h, respectively. *Statistically significant difference (p < 0.05, Welch’s t-test) relative to the result obtained for the wt strain.
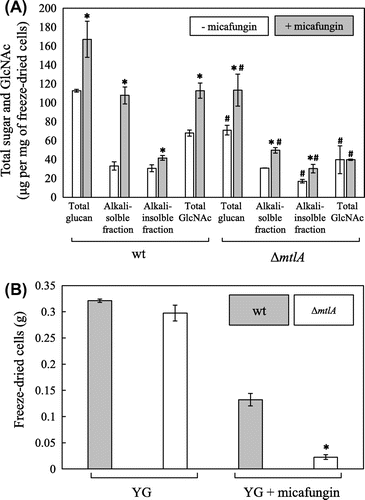
Although the wt and ΔmtlA strains grown in the absence of micafungin produced similar yields of mycelia (Fig. (B)), the total glucan, the alkali-insoluble fraction, and the total GlcNAc contents in the cell wall of the ΔmtlA strain were lower than in the wt strain (Fig. (A)). A similar reduction in the amounts of glucan and chitin in the cell wall was also observed in the ΔmtlA strain cultivated with 2 ng/mL of micafungin, a concentration at which the growth of the ΔmtlA strain was inhibited as compared with the wt strain (Fig. (B)). The total glucan, alkali-soluble and insoluble fractions, and total GlcNAc contents of the ΔmtlA strain were reduced as compared with the wt strain in the presence of micafungin (Fig. (A)). These results suggest that MtlA is involved in glucan and chitin synthesis regardless of the presence or absence of micafungin.
Effects of micafungin treatment on the transcription of cell wall biogenesis-related genes
Because it has been found that micafungin treatment transiently upregulates the transcription of agsB via MpkA-RlmA signaling in A. nidulans,Citation5) we compared the transcription of agsB in the wt, ΔmtlA, ΔwscA ΔwscB, and ΔmpkA strains to determine whether MtlA functions upstream of the MpkA-RlmA signaling pathway (Fig. ). The ΔmpkA strain was used as a negative control because it lacks the signal transduction mechanism for the upregulation of agsB.Citation5) The strains were cultivated in YG medium for 18 h, and then the medium was amended with micafungin. In the ΔwscA ΔwscB strain, transcription of agsB was induced by micafungin treatment as described previously.Citation10) On the other hand, upregulation of agsB transcription following micafungin treatment was also observed in the ΔmtlA strain, and the degree of upregulation was the same as that observed for the wt strain. These results indicate that MtlA is not essential for the activation of MpkA-RlmA signaling, and moreover, MtlA might not function upstream of that pathway.
Fig. 7. Level of transcription of agsB following exposure to micafungin in the Wt, ΔmtlA, ΔwscA ΔwscB, and ΔmpkA strains.
Note: Strains were cultured at 30 °C for 18 h, and then micafungin was added to the medium and transcription of agsB and the histone H2B gene was measured at 0 and 30 min. The copy number of the histone H2B gene was used as housekeeping control. The y-axis shows the level of mRNA relative to that of the histone H2B gene. *Statistically significant difference (p < 0.05, Welch’s t-test) relative to the result obtained at 0 min.
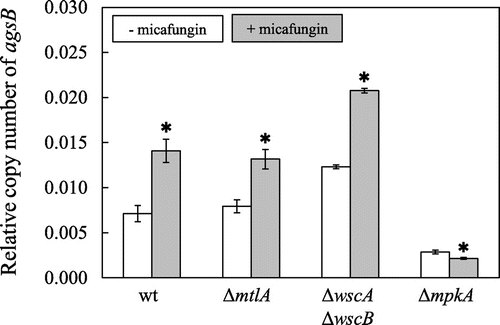
Next, we investigated to determine whether MtlA plays a role in the transcriptional regulation of other cell wall biogenesis-related genes, including the genes encoding β-1,3-glucan synthase (fksA), β-1,3-glucanosyl transferase (gelA and gelB), a glutamine-fructose-6-phosphate amidotransferase (gfaA), and various chitin synthase genes (csmA, csmB, chsA, chsB, chsC, and chsD) (Fig. S3). The transcription of gfaA depends partially on MpkA-RlmA signaling, whereas the transcription of these other genes does not,Citation5) but no differences were observed between the wt and ΔmtlA strains in terms of the transcription of these genes in response to stress associated with micafungin.
Discussion
This study indicates that in A. nidulans, MtlA is required for conidia formation and for tolerance to cell wall stress associated with exposure to CFW, Congo red, and micafungin. In contrast to the Wsc proteins, which are involved in stress tolerance of hypo-osmotic and acidic pH conditions,Citation10) MtlA is involved in tolerance of inhibitors of cell wall biogenesis. Thus, MtlA plays a role in stress tolerance that is distinct from those of WscA and WscB.
We found that the MtlA-EGFP fusion protein localizes at the cell surface and septa in A. nidulans. Localization at the cell surface is consistent with what would be predicted for a protein that senses cell wall perturbations caused by environmental stimuli. On the other hand, the significance of the observed localization to the septa is currently unclear, but it is of interest in that the septa are sites of chitin biogenesis. The septum of A. nidulans is surrounded by the plasma membrane,Citation23) indicating that localization of MtlA to the septum is structurally possible. Nevertheless, there is also a concern that mislocalization might have occurred as a result of expression driven by the alcA promoter and/or as a result of fusion with EGFP, which might have led to the degradation of MtlA-EGFP (Fig. (B)).
Our results indicate that MtlA is highly O-glycosylated, but is not N-glycosylated. Protein O-glycosylation has been found to play crucial roles in morphological development and CWI in A. nidulans.Citation24) Disruption of the genes encoding protein O-mannosyltransferases (PmtA, PmtB, and PmtC) results in increased sensitivity to CFW and Congo red.Citation13,25) Thus a failure in the O-glycosylation of MtlA might be involved in the observed phenotypes of glycosylation-deficient strains. In contrast, ScMid2 is both O- and N-glycosylated.Citation26) O-Mannosylation of ScMid2 is required for protein stability,Citation20) whereas N-glycosylation near the N-terminus (Fig. S2A, N35) might be directly involved in sensory activity.Citation26) The N35 residue in ScMid2 is not conserved in MtlA and AfMidA, indicating that the sensing mechanism in mold MtlA and AfMidA is different from that of ScMid2.
The downstream components of CWI signaling, MpkA and PkcA, play significant roles in colony formation in A. nidulans.Citation3–8) Disruption of mtlA resulted in radial colony growth similar to the wt strain in the absence of growth inhibitors, indicating that MtlA plays a lesser role in hyphal development under normal growth conditions, but conidia formation in the ΔmtlA strain was significantly reduced, indicating that MtlA is involved in asexual development in A. nidulans. The deficiency in asexual development in the ΔmtlA strain might be related to reduced synthesis of cell wall components. The amounts of cell wall glucan and chitin produced by the ΔmtlA strain were lower than in the wt strain.
Disruption of wscA and wscB caused a decrease (about 0.34-fold) in the number of cleistothecia formed, indicating that the Wsc proteins also function in sexual development in A. nidulans. One hypothesis as to why the development of cleistothecia was affected by disruption of the wscA and wscB genes is that disruption affects the regulation of cell wall synthesis. It has been reported that A. nidulans mutants lacking both melanin and α-1,3-glucan are incapable of forming cleistothecia.Citation27) A. nidulans α-1,3-glucanase (mutanase) MutA is expressed during sexual differentiation in Hülle cells and mobilizes mutan.Citation28) In the ΔwscA ΔwscB double mutant, the levels of MpkA phosphorylation and basal expression of agsB increase, resulting in increases in the alkali-soluble cell wall fraction containing α-1,3-glucan,Citation10) which might affect cleistothecia development.
A comparison of the cell wall components of the wt and ΔmtlA strains was done with micafungin as stressor in order to induce CWI signaling. The basal levels of cell wall glucan and GlcNAc were reduced in the ΔmtlA strain as compared with the wt strain, regardless of the presence or absence of micafungin. This indicates that MtlA is involved in cell wall glucan and chitin biosynthesis. Moreover, the addition of micafungin did not increase the amount of GlcNAc in the ΔmtlA strain, indicating that MtlA plays an important role in chitin biosynthesis in the presence of micafungin. Cell wall chitin has been found to play a significant role in maintaining CWI in A. nidulans,Citation29) but the change in cell wall components cannot be explained by the transcriptional levels of the cell wall synthesis-related genes investigated in this study. For example, disruption of mtlA reduced GlcNAc but did not affect the transcriptional levels of the chitin synthase genes (csmA, csmB, chsA, chsB, chsC, and chsD) under 18 h of cultivation in YG medium. To explain this, further global transcriptional analyses of cell wall metabolism, including synthesis and degradation, are required.
In this study, transcriptional upregulation of agsB after 30 min of micafungin treatment was observed in both the ΔmtlA and the wt strain. Because the ΔmtlA strain showed higher sensitivity to micafungin than the wt strain, it is unlikely that upregulation of agsB contributes significantly to tolerance of micafungin-induced stress. AgsB plays a crucial role in cell wall α-1,3-glucan biogenesis, and α-1,3-glucan is considered to have a significant role in tolerance of Congo red, but not of micafungin or CFW.Citation30) Thus, the increased sensitivity of the ΔmtlA strain to micafungin and CFW might have been due to decreased amounts of β-1,3-/1,6-glucan and/or chitin in the cell wall rather than to a decrease in the amount of α-1,3-glucan.
The WscA and MtlA proteins of A. nidulans are counterparts of the Wsc1 and Mid2 proteins, which play important roles in maintaining CWI in S. cerevisiae.Citation1) ScWsc1, but not ScMid2, plays a significant role in the protective response to cell wall perturbation caused by caspofungin.Citation31) In contrast, in A. nidulans it is MtlA, not the Wsc proteins, that is involved in tolerance of micafungin-induced stress. Thus, the roles of these proteins appear to be different in A. nidulans and S. cerevisiae.
Three candidate CWI sensors, Wsc1, Wsc3, and MidA, have been characterized in A. fumigatus.Citation11) In BLASTP searches, A. nidulans WscA, WscB, and MtlA were returned as reciprocal best hits to A. fumigatus Wsc1, Wsc3, and MidA, respectively, but the A. fumigatus MidA disruption mutant showed a different growth deficiency. Dichtl et al. concluded that MidA is involved in tolerance of elevated temperature, CFW, and Congo red in A. fumigatus, but is dispensable in resistance to caspofungin. Moreover, A. fumigatus Wsc1 is required for caspofungin tolerance. Thus, the results of this and our previous studyCitation10) suggest that the Mid and Wsc proteins play different roles even in A. fumigatus and A. nidulans. These differences might have to do with different evolutionary histories. For example, unlike A. nidulans, A. fumigatus is an opportunistic pathogen that causes the disease invasive aspergillosis. This is supported by a study indicating that the upstream sensors and downstream transcriptional factors have evolved more rapidly than the central signaling transducers in fugal signal transduction pathways.Citation32) In fact, the 57% BLASTP identity between A. nidulans MtlA and A. fumigatus MidA is significantly lower than that between central signal transducers (e.g. RhoA, PkcA, and MpkA of A. nidulans show BLASTP identities of 94%, 84%, and 88%, respectively, to the counterparts of A. fumigatus).
In conclusion, the MtlA protein plays significant roles in conidia formation, cell wall synthesis, and tolerance of stress associated with cell wall inhibitors CFW, Congo red, and micafungin, but it is not involved in hyphal growth or the response to high temperatures. In addition, putative CWI sensor proteins appear to be involved in cleistothecia development in A. nidulans. The results of this study and those of previous onesCitation10,11) suggest that the sensory ability of the putative sensor proteins differs not only between Aspergillus species and S. cerevisiae, but also among Aspergillus species. Thus a species-level study is important to clarify the functions of sensor orthologs.
Supplemental material
The supplemental material for this paper is available at http://dx.doi.org/10.1080/09168451.2014.878218.
Figures S1, S2 and S3, and Table S1
Download PDF (342.4 KB)Funding
This work was supported in part by Japan Society for the Promotion of Science (JSPS) Grants-in-Aid for Scientific Research [grant number 23780084].
Notes
Abbreviations: CFW, calcofluor white; CWI, cell wall integrity; MAPK, mitogen-activated protein kinase; MM, minimal medium; SDS-PAGE, sodium dodecyl sulfate-polyacrylamide gel electrophoresis; TFMS, trifluoromethanesulfonic acid.
References
- Levin DE. Regulation of cell wall biogenesis in Saccharomyces cerevisiae: the cell wall integrity signaling pathway. Genetics. 2011;189:1145–1175.10.1534/genetics.111.128264
- Guest GM, Lin X, Momany M. Aspergillus nidulans RhoA is involved in polar growth, branching, and cell wall synthesis. Fungal Genet. Biol. 2004;41:13–22.10.1016/j.fgb.2003.08.006
- Ichinomiya M, Uchida H, Koshi Y, Ohta A, Horiuchi H. A protein kinase C-encoding gene, pkcA, is essential to the viability of the filamentous fungus Aspergillus nidulans. Biosci. Biotechnol. Biochem. 2007;71:2787–2799.10.1271/bbb.70409
- Ronen R, Sharon H, Levdansky E, Romano J, Shadkchan Y, Osherov N. The Aspergillus nidulans pkcA gene is involved in polarized growth, morphogenesis and maintenance of cell-wall integrity. Curr. Genet. 2007;51:321–329.10.1007/s00294-007-0129-y
- Fujioka T, Mizutani O, Furukawa K, Sato N, Yoshimi A, Yamagata Y, Nakajima T, Abe K. MpkA-Dependent and -independent cell wall integrity signaling in Aspergillus nidulans. Eukaryot. Cell. 2007;6:1497–1510.10.1128/EC.00281-06
- Teepe AG, Loprete DM, He Z, Hoggard TA, Hill TW. The protein kinase C orthologue PkcA plays a role in cell wall integrity and polarized growth in Aspergillus nidulans. Fungal Genet. Biol. 2007;44:554–562.10.1016/j.fgb.2006.10.001
- Colabardini AC, De Castro PA, de Gouvêa PF, Savoldi M, Malavazi I, Goldman MH, Goldman GH. Involvement of the Aspergillus nidulans protein kinase C with farnesol tolerance is related to the unfolded protein response. Mol. Microbiol. 2010;78:1259–1279.10.1111/j.1365-2958.2010.07403.x
- Katayama T, Uchida H, Ohta A, Horiuchi H. Involvement of protein kinase C in the suppression of apoptosis and in polarity establishment in Aspergillus nidulans under conditions of heat stress. PLoS ONE. 2012;7:e50503.10.1371/journal.pone.0050503
- Ram AF, Klis FM. Identification of fungal cell wall mutants using susceptibility assays based on Calcofluor white and Congo red. Nat. Protoc. 2006;1:2253–2256.10.1038/nprot.2006.397
- Futagami T, Nakao S, Kido Y, Oka T, Kajiwara Y, Takashita H, Omori T, Furukawa K, Goto M. Putative stress sensors WscA and WscB are involved in hypo-osmotic and acidic pH stress tolerance in Aspergillus nidulans. Eukaryot. Cell. 2011;10:1504–1515.10.1128/EC.05080-11
- Dichtl K, Helmschrott C, Dirr F, Wagener J. Deciphering cell wall integrity signalling in Aspergillus fumigatus: identification and functional characterization of cell wall stress sensors and relevant Rho GTPases. Mol. Microbiol. 2012;83:506–519.10.1111/mmi.2012.83.issue-3
- Futagami T, Goto M. Putative cell wall integrity sensor proteins in Aspergillus nidulans. Commun. Integr. Biol. 2012;5:206–208.10.4161/cib
- Goto M, Harada Y, Oka T, Matsumoto S, Takegawa K, Furukawa K. Protein O-mannosyltransferase B and C support hyphal development and differentiation in Aspergillus nidulans. Eukaryot. Cell. 2009;8:1465–1474.10.1128/EC.00371-08
- Yelton MM, Hamer JE, Timberlake WE. Transformation of Aspergillus nidulans by using a trpC plasmid. Proc. Nat. Acad. Sci. USA. 1984;81:1470–1474.10.1073/pnas.81.5.1470
- Toews MW, Warmbold J, Konzack S, Rischitor P, Veith D, Vienken K, Vinuesa C, Wei H, Fischer R. Establishment of mRFP1 as a fluorescent marker in Aspergillus nidulans and construction of expression vectors for high-throughput protein tagging using recombination in vitro (GATEWAY). Curr. Genet. 2004;45:383–389.
- Yamazaki H, Tanaka A, Kaneko J, Ohta A, Horiuchi H. Aspergillus nidulans ChiA is a glycosylphosphatidylinositol (GPI)-anchored chitinase specifically localized at polarized growth sites. Fungal Genet. Biol. 2008;45:963–972.10.1016/j.fgb.2008.02.008
- Richie DL, Hartl L, Aimanianda V, Winters MS, Fuller KK, Miley MD, White S, McCarthy JW, Latge JP, Feldmesser M, Rhodes JC, Askew DS. A role for the unfolded protein response (UPR) in virulence and antifungal susceptibility in Aspergillus fumigatus. PLoS Pathog. 2009;5:e1000258.10.1371/journal.ppat.1000258
- Reissig JL, Storminger JL, Leloir LF. A modified colorimetric method for the estimation of N-acetylamino sugars. J. Biol. Chem. 1955;217:959–966.
- Borgia PT, Dodge CL. Characterization of Aspergillus nidulans mutants deficient in cell wall chitin or glucan. J. Bacteriol. 1992;174:377–383.
- Rajavel M, Philip B, Buehrer BM, Errede B, Levin DE. Mid2 is a putative sensor for cell integrity signaling in Saccharomyces cerevisiae. Mol. Cell. Biol. 1999;19:3969–3976.
- Fontaine T, Simenel C, Dubreucq G, Adam O, Delepierre M, Lemoine J, Vorgias CE, Diaquin M, Latge JP. Molecular organization of the alkali-insoluble fraction of Aspergillus fumigatus cell wall. J. Biol. Chem. 2000;275:41528.
- Lee HH, Park JS, Chae SK, Maeng PJ, Park HM. Aspergillus nidulans sod(VI)C1 mutation causes defects in cell wall biogenesis and protein secretion. FEMS Microbiol. Lett. 2002;208:253–257.10.1111/fml.2002.208.issue-2
- Momany M, Hamer JE. Relationship of actin, microtubules, and crosswall synthesis during septation in Aspergillus nidulans. Cell Motil. Cytoskeleton. 1997;38:373–384.10.1002/(ISSN)1097-0169
- Goto M. Protein O-glycosylation in fungi; diverse structures and multiple functions. Biosci. Biotechnol. Biochem. 2007;71:1415–1427.10.1271/bbb.70080
- Oka T, Hamaguchi T, Sameshima Y, Goto M, Furukawa K. Molecular characterizations of protein O-mannosyltransferase and its involvement in cell wall synthesis in Aspergillus nidulans. Microbiology. 2004;150:1973–1982.10.1099/mic.0.27005-0
- Hutzler F, Gerstl R, Lommel M, Strahl S. Protein N-glycosylation determines functionality of the Saccharomyces cerevisiae cell wall integrity sensor Mid2p. Mol. Microbiol. 2008;68:1438–1449.10.1111/mmi.2008.68.issue-6
- Polacheck I, Rosenberger RF. Aspergillus nidulans mutant lacking α-(1,3)-glucan, melanin, and cleistothecia. J. Bacteriol. 1977;132:650–656.
- Wei H, Scherer M, Singh A, Liese R, Fischer R. Aspergillus nidulans α-1,3-glucanase (mutanase), mutA, is expressed during sexual development and mobilises mutan. Fungal Genet. Biol. 2001;34:217–227.10.1006/fgbi.2001.1303
- Horiuchi H. Functional diversity of chitin synthases of Aspergillus nidulans in hyphal growth, conidiophore development and septum formation. Med. Mycol. 2009;47:S47–S52.10.1080/13693780802213332
- Yoshimi A, Sano M, Inaba A, Kokubun Y, Fujioka T, Mizutani O, Hagiwara D, Fujikawa T, Nishimura M, Yano S, Kasahara S, Shimizu K, Yamaguchi M, Kawakami K, Abe K. Functional analysis of the α-1,3-glucan synthase genes agsA and agsB in Aspergillus nidulans: agsB is the major α-1,3-glucan synthase in this fungus. PLoS ONE. 2013;8:e54893.10.1371/journal.pone.0054893
- Reinoso-Martin C, Schuller C, Schuetzer-Muehlbauer M, Kuchler K. The yeast protein kinase C cell integrity pathway mediates tolerance to the antifungal drug caspofungin through activation of Slt2p mitogen-activated protein kinase signaling. Eukaryot. Cell. 2003;2:1200–1210.10.1128/EC.2.6.1200-1210.2003
- Nikolaou E, Agrafioti I, Stumpf M, Quinn J, Stansfield I, Brown AJ. Phylogenetic diversity of stress signalling pathways in fungi. BMC Evol. Biol. 2009;9:44. doi:10.1186/1471-2148-9-44.