Abstract
The fermentative properties of thermo-sensitive strain Corynebacterium glutamicum 2262 were investigated in processes coupling aerobic cell growth and the anaerobic fermentation phase. In particular, the influence of two modes of fermentation on the production of lactate, the fermentation product model, was studied. In both processes, lactate was produced in significant amount, 27 g/L in batch culture, and up to 55.8 g/L in fed-batch culture, but the specific production rate in the fed-batch culture was four times lower than that in the batch culture. Compared to other investigated fermentation processes, our strategy resulted in the highest yield of lactic acid from biomass. Lactate production by C. glutamicum 2262 thus revealed the capability of the strain to produce various fermentation products from pyruvate.
Carboxylic acids, among them mono (i.e. acetate, butyrate, lactate, etc.) and diacids (succinate, malate, etc.), are generally regarded as building block chemicals. Due to their carboxylic functional groups, these molecules are valuable in synthesis of higher molecular weight compounds such as polymers. Nowadays, numerous organic acids are largely if not exclusively produced through microbiological processes,Citation1–3) and such production is regarded as an important economic alternative to chemical synthesis.Citation4) For example, citric acid, the yearly production of which is about 1.4 million tons,Citation5) is produced only by fermentation using mainly the filamentous fungus Aspergillus niger. It is noteworthy that the economic viability of microbial processes is due mainly to the capacity of industrial micro-organisms to use a large variety of substrates, among them cheap raw materials such as molasses, whey, starch waste, and beets.
At an annual production of 2.5 million tons of L-glutamate and 1.5 million tons of L-lysine, Corynebacterium glutamicum is the major amino acid producer. The processes occur under aerobic conditions, at high rates and productivities, enabling C. glutamicum to hold the leading position in this field.Citation6) Alternatively, and like many aerobic and facultative anaerobic bacteria, C. glutamicum is also capable of producing organic acids under anaerobic conditions. Using wild-type or recombinant strains, organic acids such as succinate and lactate have been reported to be produced in significant amounts,Citation7–10) but also alcohols such as ethanolCitation11) and isobutanol,Citation12) under oxygen-depleted conditions. In C. glutamicum, a metabolic switch that takes place during the transition from aerobic to anaerobic conditions has been documented. Under oxygen transfer limiting conditions and strict oxygen deprivation, an increase in glucose consumption rates occurs resulting in an increase in gene expression coding for enzymes of the glycolytic and organic acid synthesis pathways such as gapA, pgk, ppc, mdh, and ldhA.Citation13) Upregulation of these genes is controlled by SigB, a group-2 sigma factor, which has been found to be expressed during the transition from exponential phase to stationary phase, under various stresses, and under oxygen deprivation in C. glutamicum.Citation14) As a consequence, a metabolic shift is driven from the glycolytic pathway toward the reductive arm of the TCA cycle, enabling an increase in organic acid production.Citation15) From a biotechnological point of view, these modifications of the physiological state of cells during the transition from aerobic to anaerobic conditions probably constitute critical points. Recent studies have reported that the enzymatic machinery, adjusted by the application of a microaerobic phase at the end of the aerobic growth phase of E. coli, greatly influences the performance of cells in the anaerobic production phase.Citation16,17)
Pyruvate, produced at the last step of glycolysis, is located at the crossroad of most fermentation pathways and can be reduced directly to lactate by lactate dehydrogenase to regenerate the NAD+ pool under oxygen limitated conditions. Hence, lactate production capabilities can be regarded as a good indicator of the fermentative potential of microbial strains when oxygen is limited. Recently, C. glutamicum was reported to produce lactate anaerobically at a high volumetric productivity of 42.9 g/L h, but these results were obtained with high cell density cultures made with washed cells previously cultivated aerobically. Besides the fact it can be hardly expanded to industrial scales, this washing procedure may also strongly affect the physiological state of cells due to various stresses, such as acceleration during the centrifugation step, and cold shock.Citation18,19)
The aim of this study was to determine the fermentative potential of thermo-sensitive strain C. glutamicum 2262 through a one-step lactate fermentation process, associating the aerobic cell growth phase and the anaerobic fermentation phase. In particular, the influence of the duration of the aerobic phase on the second phase, i.e. the production of lactate by C. glutamicum 2262 under oxygen-deprivated conditions, was investigated in batch and fed-batch cultures.
Materials and methods
Bacteria and culture medium
The strain used throughout this study was C. glutamicum 2262.Citation20) The culture medium used was based on the MCGC medium.Citation21) Our modified MCGC consisted of (per L): 40 g, glucose, 3 g, Na2HPO4, 6 g, KH2PO4, 2 g, NaCl, 8 g, (NH4)2SO4, 400 mg, MgSO4.7H2O, 40 mg, FeSO4.7H2O, 4 mg, FeCl3, 1 mg, ZnSO4.7H2O, 0.4 mg CuCl2.2H2O, 4 mg, MnSO4.H2O, 0.2 mg, (NH4)6Mo7O2.4H2O, 0.4 mg, Na2B4O7.10H2O, 84 mg, CaCl2, 2 mg, biotin, 20 mg, thiamine, 3 mg, deferoxamine, and 2 g betaine. Polypropylene glycol (1.3 g/L) was used as antifoaming agent.
Fermentation conditions
Pre-culture of C. glutamicum 2262 was performed in a 500-mL flask containing 50 mL of modified MCGC medium. The cultures were incubated at 33 °C at 200 rpm. The pre-culture was used to inoculate a 2-L fully instrumented bioreactor (Applikon) containing initially 1.5 L of modified MCGC at 33 °C. The pH set point was regulated at 7.6 by the addition of NH3 12 N. In the first phase, the culture medium was oxygenated by the addition of air. During culture, the dissolved oxygen concentration was measured online with an oxygen electrode (Mettler Toledo) and controlled when needed by varying the stirring speed and airflow rate. The airflow rate was varied from 1 to 5 L/min and agitation from 500 to 1200 rpm.
Dissolved oxygen was initially set at 100% and was maintained at 30% of saturation until the biomass concentration reached the desired OD570. At this point, aeration was stopped and the stirring speed was reduced to 200 rpm, resulting in a drop in oxygen partial pressure. In fed-batch experiments, glucose was fed with a solution of 350 g/L glucose to maintain a residual concentration close to 5 g/L. All over the culture, 5 mL samples were collected to quantify biomass (C. glutamicum 2262), substrate (glucose), and the product (acetate, lactate, and succinate) concentrations.
Analytical methods
The biomass concentration was determined for each sample by measurement of absorbance at 570 nm (Spectrophotometer DU 7500, Beckman). The correlation coefficient between the OD and dry weight of C. glutamicum 2262 was 0.26 g/L per OD570 unit.
After sample centrifugation (5 min, 10.000 rpm, microfuge®lite, Beckman), the glucose and lactic acid concentrations were measured enzymatically in the supernatants (Elitech). The succinic and acetic acid concentrations were determined in the supernatants by High Pressure Liquid Chromatography (SPD-M10A VP, Shimadzu) with a Polypore H (Bio-Rad) column maintained at 50 °C. Separation was done with acidic water (5 mM H2SO4). The signal is measured with an UV detector (Shimadzu).
Kinetic parameter calculations for the fermentation processes
The specific rates of cell growth (µ) and lactate (π) and glucose consumption (ν) were calculated by the following equations:
where X, S, and P are the biomass concentration (g/L), and the concentrations of substrates and products (g/L), respectively.
Other parameters were calculated as follows:
where YX/S, yield of biomass from glucose in growth phase (g/g); X, biomass concentration at the end of growth phase (g/L); X0, biomass concentration at the beginning of growth phase (g/L); S0, glucose concentration at the beginning of growth phase (g/L); and S, glucose concentration at the end of growth phase (g/L).
whereYP/S, yield of lactate from glucose in production phase (g/g); Pf, lactate concentration at the end of production phase (g/L); P0, lactate concentration at the beginning of production phase (g/L); S0, glucose concentration at the beginning of production phase (g/L); and Sf, glucose concentration at the end of production phase (g/L).
where YP/X, yield of lactate produced by biomass in production phase (g/g); Pf, lactate concentration at the end of production phase (g/L); and Xf, glucose concentration at the end of production phase (g/L).
where P, productivity (g/L h); Pf, lactate concentration at the end of production phase (g/L); and t, fermentation time (h).
Results
Influence of the transition point from aerobic to anaerobic conditions in a batch culture
As previously indicated, facultative anaerobic C. glutamicum produces fermentation products under anaerobic conditions, but its growth is poor if not completely abolished (see below). Hence, an aerobic phase, preceding the anaerobic one and necessary for cell growth, must be implemented in the process.
In order to determine the suitable point of transition from aerobic to anaerobic conditions in batch culture, the growth characteristics of C. glutamicum 2262 under aerobic conditions were investigated. At an initial glucose concentration of 40 g/L, the highest biomass concentration, 18.7 g/L, was reached after 8 h of culture, with a maximum specific growth rate of 0.64 h−1. Under this condition, the glucose was exhausted after 8 h of culture, and a low but significant production of lactate, up to 0.9 g/L, was detected. This was due to oxygen limitation (data not shown) as a consequence of the massive production of biomass and the difficulty of maintaining an efficient oxygenation at the highest agitation and aeration rates (Fig. ). Thus, lactate is consumed by the micro-organism. Hence, an absence of aeration in combination with a decrease in the stirring rate to establish the production phase of C. glutamicum in the most favorable physiological state is important in developing a one-step fermentation process. Under these conditions, lactate production was induced in batch cultures after a first aerobic phase for biomass accumulation. Suppression of the aeration was applied at various OD570 values of 3, 10, 30, and 60 (Fig. ).
Fig. 1. Kinetics of growth, glucose consumption, and lactate production by C. glutamicum 2262 under aerobic conditions during a batch process.
Note: Time courses of growth (a) glucose consumption (b), and lactate production (c). Dotted lines represent corresponding specific rates (g/g h).
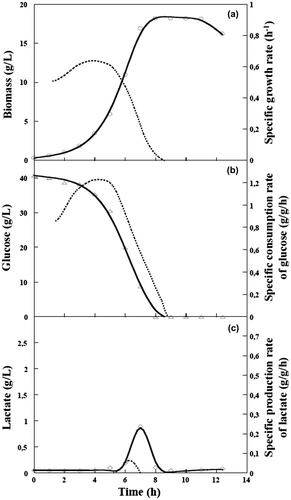
Fig. 2. Lactate production by C. glutamicum 2262 in batch culture under oxygen-deprived conditions.
Note: Time-course profiles of growth (a, d, g, j), glucose consumption (b, e, h, k), and lactate production (c, f, i, l). Dotted lines represent corresponding specific rates (g/g h). The black arrow and the vertical broken line indicate the time when oxygen deprivation was applied.
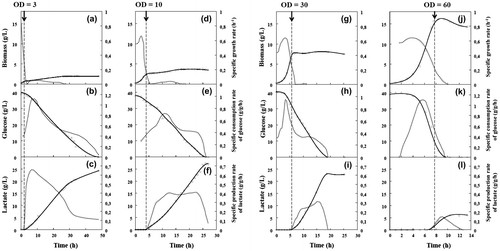
These four OD570 values are representative of different cell growth phase. At an OD570 of 3 (a biomass concentration of 0.78 g L−1), cells were in the accelerating growth phase. A second OD570 value of 10 (a biomass concentration of 2.6 g/L) corresponded to cells in exponential phase, with a maximal specific growth rate and a specific glucose consumption rate of 0.64 h−1 and 0.71 g/g h, respectively. The third and fourth OD570 values, 30 and 60 (biomass concentration of 7.8 and 15.6 g/L), are representative of cells in early and late decelerating phases, respectively.
The kinetics of growth, glucose consumption, and lactic acid formation by C. glutamicum 2262 in these cultures are reported in Fig. . As expected,Citation22) the highest growth rate, 0.64 h−1, was reached during the aerobic phase and was similar in all cultures (Fig. (d, g, j)) except for the first condition (Fig. (a)). During this period, glucose was used mostly to support the cellular growth, as indicated by the absence of fermentation products (Fig. ).
As indicated in Fig. , suppression of aeration at different OD570 values had a dramatic effect on cell growth and provoked rapid lactic acid excretion. Suppression of aeration during the accelerating phase (OD570 of 3) led to the production of 24.5 g/L lactic acid with 2 g/L biomass and resulted in a productivity of 0.54 g/L h after 47 h of culture (Table ). Without aeration, C. glutamicum 2262 continued to grow until 25 h of culture. Hence, glucose was used not only for lactate production but also to support the growth of the cells (Fig. (a)).
Table 1. Performance of the lactate production process with C. glutamicum 2262 in the production phase of one-step batch culture.
When the switch from the aerobic to the anaerobic phase was performed at an OD570 of 10, the specific growth rate decreased, and, this led to an accumulation of biomass of 3.7 g/L after 26 h of culture (Fig. (d)). As expected, the cells produced lactate immediately upon oxygen deprivation and accumulated up to 27 g L−1 until glucose was completely consumed at 26 h of culture (Fig. (e, f)). Under these conditions, the specific rate of glucose consumption reached a maximal value of 0.71 g/g h 7 h after suppression of aeration (Fig. (e)). During the anaerobic phase, lactate was produced continuously and it reached a maximal specific production rate of 0.4 g/g h at 14 h of oxygen deprivation (Fig. (f)). Lactate was produced continuously until the carbon source was exhausted, suggesting that most of the glucose was used up in lactate production during the anaerobic phase.
When initiated at an OD570 value of 30, the anaerobic phase resulted in kinetics of cell growth, glucose consumption, and lactate production similar to those obtained when oxygen deprivation was imposed at an OD570 of 10. As shown in Fig. , cell growth was completely abolished under anaerobic conditions, and most of the glucose was used for the production of lactate (Fig. (g, h, i)). Under these conditions, the final lactate and biomass concentrations were 23 and 8.1 g/L, respectively, at 19 h of culture.
When aeration was suppressed at an OD570 of 60, complete growth arrest was observed as well as there is a slight production of lactate (up to 6 g/L) at 12 h of culture. This was clearly due to the rapid exhaustion of residual glucose (Fig. (j)).
From these results, it appears that the cell concentration obtained when the switch from the aerobic to the anaerobic phase occurred had a strong influence on lactate production. As shown in Table , the suppression of aeration at different OD570 values dramatically affected the yields of lactate from glucose (YP/S) and lactate produced by biomass (YP/X), and productivities. Although the YP/S values were average when suppression of the aeration was performed at an OD570 value of 3, lactate productivity was the lowest among the conditions, whereas the yield of lactate produced by biomass (YP/X) was the highest (Table ). The highest yields of lactate from glucose, 0.79 and 0.8, were obtained when suppression of the aeration was performed at OD570 values of 10 and 30, respectively. Under this last condition, the highest lactic acid productivity of 1.65 g/L h was obtained.
Production of lactate during the fed-batch process
In fed-batch mode, the production of lactate by C. glutamicum 2262 under oxygen-deprived conditions can be extended during the anaerobic phase, i.e. when glucose is metabolized into fermentation products. Hence, C. glutamicum cultures were supplied with glucose when aeration was suppressed at OD570 values of 10 and 30, under the same initial operating conditions as for the batch culture experiments. In fed-batch mode, glucose was added to the culture medium by pulse to maintain the residual concentration at over 5 g/L without exceeding 30 g/L.
When oxygen was depleted at an OD570 of 10, the specific rate of glucose consumption reached a maximal value of 0.5 g/g h in 12 h of fermentation (Fig. (b)) and then decreased progressively to 0.05 g/g h at 50 h, but, the specific rates of glucose consumption and lactic acid production remained constant and relatively low, even though lactic acid was produced steadily (Fig. (b, c)). The specific rate of lactate production reached a maximum of 0.42 g/g h before the first glucose feeding and then decreased rapidly for 30–50 h reaching a minimum value of 0.02 g/g h until the end of fermentation. This fed-batch fermentation resulted in a lactate production of 83 g, corresponding to a final concentration of 55.8 g/L (Table ). In addition to lactate, 15.3 g/L, succinate was produced at 220 h of culture (data not shown).
Fig. 3. Lactate production by C. glutamicum 2262 in fed-batch culture under oxygen-deprived conditions.
Note: Time-course profiles of growth (a, d), glucose consumption (b, e), and lactate production (c, f). Because of dilution due to the fed-batch process, glucose consumption and lactate production are expressed in grams (g) instead of grams per liter (g/L). Dotted lines represent corresponding specific rates (g/g h). The black arrow and the vertical broken line indicate the time when oxygen deprivation was applied. Broken arrows indicate glucose addition to the bioreactor.
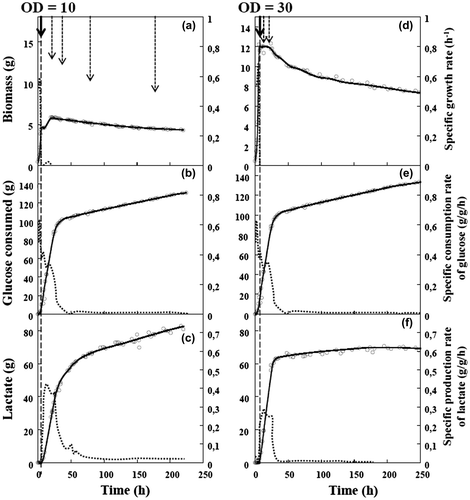
Table 2. Performance of the lactate production process with C. glutamicum 2262 in the production phase of one-step fed-batch culture.
The depletion of oxygen at OD570 value of 30 had a strong effect on the performance of the culture. Although the concentration profiles over the first 26 h were similar to those of the previous fed-batch process (oxygen depletion at an OD570 of 10), the specific rates of glucose consumption and lactate production dropped, close to zero, resulting in a complete cessation of lactate production even in the presence of the high concentration of glucose remaining in the medium (Fig. (d, e, f)). As a consequence, the yield of lactate produced by biomass (YP/X) was three times lower than the yield obtained when aeration was suppressed at an OD570 value of 10 (Table ). In this fed-batch culture, succinate was also produced, at up to of 21 g/L at 220 h of culture (data not shown). In contrast, less than 1 g/L of acetate was produced in batch and fed-batch cultures (data not shown).
Comparing the batch and fed-batch processes carried out with oxygen depletion at an OD570 of 30, the glucose-to-lactic acid conversion yield (YP/S) and the lactic acid productivities (P) in batch mode exceeded by more than 1.3- and 7.8-fold, respectively, those obtained in the fed-batch cultures (Tables and ). This difference can be explained by a difference in glucose utilization in the two culture modes: 38% of the carbon was recovered in succinate and other products at the end of the fed-batch culture, while this value was only 17% in batch mode (data not shown). However, the yield of lactate produced by biomass (YP/X) was twofold higher in fed-batch than in batch culture. In fact, the fed-batch mode increased the metabolically active period of the cells, resulting in an extended lactate production phase. However, as shown in Fig. (c), the specific rate of lactate production remained at a very low value of less than 0.05 g/g h after 61 g of lactate was produced (corresponding to a concentration of 40 g/L), and although more than 17 g/L of glucose remained in the culture medium. This decline in the lactate production rate might have been a consequence of end-product inhibition,Citation23) and also of a decrease in metabolic potential due to the prolonged anaerobic period of the microbial biocatalyst. Further study to characterize the metabolic behavior of C. glutamicum cultivated anaerobically over a prolonged period of time might be useful in the attempt to improve process performance.
Discussion
The physiological state of C. glutamicum is crucial for the production of organic acid under oxygen deprivation conditions, and is obviously influenced during the aerobic growth phase preceding the anaerobic production phase. Improvement of fermentation productions can be achieved by genetic engineering, by process optimization, or by combining the two approaches. In C. glutamicum, genetic engineering strategies consisting in the addition or the deletion of competing pathways are not always successful, since the metabolic fluxes are precisely controlled by cellular regulation mechanisms.Citation7,24) For example, inactivation of the ppc, pyc, ppc-pyc, pckA, fum, and aceA genes, which impaired the succinic acid and the acetic acid synthetic pathways, respectively, in C. glutamicum, has shown no benefit in lactate production.Citation24)
In this study, process conditions for optimal production of lactate as a representative of most of the fermentation products deriving directly or indirectly from pyruvate were established. Under oxygen deprivation, C. glutamicum strain 2262 produced 27 g/L and 55.8 g/L lactate in batch and fed-batch culture, respectively,. However, physiological changes in C. glutamicum during the transition from aerobic to oxygen deprivation conditions remain largely unknown, although they appeared to be key parameters in improving fermentation performance. Martinez et al.,Citation16) and Vemuri et al.,Citation17) have shown that the physiological state of the cell during the transition from aerobic to anaerobic condition significantly influenced overall fermentation product formation by succinate-producing E. coli strains.
In addition to lactate, significant amounts of acetate (about 1 g/L) and succinate (6 and 21 g/L) were produced by both batch and fed-batch processes. These observations are in agreement with studies reporting the ability of C. glutamicum to produce significant amounts of organic acids from glucose despite cessation of growth under oxygen-deprived conditions.Citation7,13,24) Our study also demonstrates that C. glutamicum 2262 can utilize lactate formed during the aerobic phase when glucose is exhausted. This is in agreement with previously reported studies, indicating that C. glutamicum is able to grow using lactate as sole carbon source under aerobic conditions,Citation25,26) probably through respiratory quinone-dependent LDH.Citation27,28)
In contrast, under oxygen deprivation, lactate was consumed only when glucose was exhausted in the culture medium.
Lactate production by C. glutamicum has been also studied by Okino et al.Citation7) In their study, a single anaerobic production phase with high cell density cultures made with washed cells was carried out. A final lactic acid concentration of 10 g/L, with a yield of lactate from glucose of 0.89 g/g, was achieved at a cell concentration of 10 g/L after 2 h of batch culture, whereas 52 g/L lactate was produced at 8 h of fed-batch culture with an initial concentration of 30 g/L of washed cells. In our study, the yields of lactate produced by biomass (YP/X) were 7–8-fold higher than yields obtained by Okino et al.Citation7), especially when suppression of the aeration occurred at an OD570 of 10 (1 g/g vs. 7.3 g/g and 1.7 g/g vs. 14.3 g/g in the batch and fed-batch processes, respectively).
These results were obtained by a simple one-step fermentation process operated at low initial biomass concentration of 2.6 g/L. Thus, the yields of lactate produced by biomass (YP/X) obtained in our study are much higher than those reported. In addition, compared to other fermentation procedures previously described, which result in higher final product concentrations, additional handling steps are generally required, including a washing step and a very high biomass concentration of 30 g/L.Citation7,24)
Further studies are needed to improve the production yields of fermentation product by C. glutamicum. Indeed, with an average yield of lactate production (YP/S) of 1.6 mol/mol, an increase of 20% can be gained on the basis of the theoretical maximum yield of 2 mol/mol.
Acknowledgments
The authors acknowledge the French Embassy in Vietnam through the Evarist Galois Program and the Institut Carnot Énergie et Environnement en Lorraine (ICEEL) for their support.
Notes
Abbreviation: LDH, lactate dehydrogenase.
References
- Tsao GT, Cao NJ, Du J, Gong CS. Production of multifunctional organic acids from renewable resources. Adv. Biochem. Eng. Biotechnol. 1999;65:243–280.
- Chotani G, Dodge T, Hsu A, Kumar M, LaDuca R, Trimbur D, Weyler W, Sanford K. The commercial production of chemicals using pathway engineering. Biochim. Biophys. Acta. 2000;1543:434–455.10.1016/S0167-4838(00)00234-X
- Ohara H. Biorefinery. Appl. Microbiol. Biotechnol. 2003;62:474–477.10.1007/s00253-003-1383-7
- Sauer M, Porro D, Mattanovich D, Branduardi P. Microbial production of organic acids: expanding the markets. Trends Biotechnol. 2008;26:100–108.10.1016/j.tibtech.2007.11.006
- Soccol CR, Vandenberghe LPS, Rodrigues C, Pandey A. New Perspectives for Citric Acid Production and Application. Food Technol. Biotechnol. 2006;44:141–149.
- Becker J, Wittmann C. Systems and synthetic metabolic engineering for amino acid production – the heartbeat of industrial strain development. Curr. Opin. Biotechnol. 2012;23:718–726.10.1016/j.copbio.2011.12.025
- Okino S, Inui M, Yukawa H. Production of organic acids by Corynebacterium glutamicum under oxygen deprivation. Appl. Microbiol. Biotechnol. 2005;68:475–480.10.1007/s00253-005-1900-y
- Okino S, Noburyu R, Suda M, Jojima T, Inui M, Yukawa H. An efficient succinic acid production process in a metabolically engineered Corynebacterium glutamicum strain. Appl. Microbiol. Biotechnol. 2008;81:459–464.10.1007/s00253-008-1668-y
- Okino S, Suda M, Fujikura K, Inui M, Yukawa H. Production of D-lactic acid by Corynebacterium glutamicum under oxygen deprivation. Appl. Microbiol. Biotechnol. 2008;78:449–454.10.1007/s00253-007-1336-7
- Litsanov B, Brocker M, Bott M. Toward homosuccinate fermentation: metabolic engineering of Corynebacterium glutamicum for anaerobic production of succinate from glucose and formate. Appl. Environ. Microbiol. 2012;78:3325–3337.10.1128/AEM.07790-11
- Inui M, Kawaguchi H, Murakami S, Vertes AA, Yukawa H. Metabolic engineering of Corynebacterium glutamicum for fuel ethanol production under oxygen-deprivation conditions. J. Mol. Microbiol. Biotechnol. 2004;8:243–254.10.1159/000086705
- Blombach B, Riester T, Wieschalka S, Ziert C, Youn JW, Wendisch VF, Eikmanns BJ. Corynebacterium glutamicum tailored for efficient isobutanol production. Appl. Environ. Microbiol. 2011;77:3300–3310.10.1128/AEM.02972-10
- Inui M, Suda M, Okino S, Nonaka H, Puskas LG, Vertes AA, Yukawa H. Transcriptional profiling of Corynebacterium glutamicum metabolism during organic acid production under oxygen deprivation conditions. Microbiology 2007;153:2491–2504.10.1099/mic.0.2006/005587-0
- Ehira S, Shirai T, Teramoto H, Inui M, Yukawa H. Group 2 sigma factor SigB of Corynebacterium glutamicum positively regulates glucose metabolism under conditions of oxygen deprivation. Appl. Environ. Microbiol. 2008;74:5146–5152.10.1128/AEM.00944-08
- Yamamoto S, Sakai M, Inui M, Yukawa H. Diversity of metabolic shift in response to oxygen deprivation in Corynebacterium glutamicum and its close relatives. Appl. Microbiol. Biotechnol. 2011;90:1051–1061.10.1007/s00253-011-3144-3
- Martinez I, Bennett GN, San KY. Metabolic impact of the level of aeration during cell growth on anaerobic succinate production by an engineered Escherichia coli strain. Metab. Eng. 2010;12:499–509.10.1016/j.ymben.2010.09.002
- Vemuri GN, Eiteman MA, Altman E. Succinate production in dual-phase Escherichia coli fermentations depends on the time of transition from aerobic to anaerobic conditions. J. Ind. Microbiol. Biotechnol. 2002;28:325–332.10.1038/sj.jim.7000250
- Champagne CP, Gardner NJ. Effect of process parameters on the production and drying of Leuconostoc mesenteroides cultures. J. Ind. Microbiol. Biotechnol. 2002;28:291–296.10.1038/sj.jim.7000245
- Horn G, Hofweber R, Kremer W, Kalbitzer HR. Structure and function of bacterial cold shock proteins. Cell. Mol. Life Sci. 2007;64:1457–1470.10.1007/s00018-007-6388-4
- Delaunay S, Gourdon P, Lapujade P, Mailly E, Oriol E, Engasser JM, Lindley ND, Goergen J-L. An improved temperature-triggered process for glutamate production with Corynebacterium glutamicum. Enzyme Microb. Technol. 1999;25:762–768.10.1016/S0141-0229(99)00120-9
- Von der Osten CH, Gioannetti C, Sinskey AJ. Design of a defined medium for growth of Corynebacterium glutamicum in which citrate facilitates iron uptake. Biotechnol. Lett. 1989;11:11–16.10.1007/BF01026778
- Bokas D, Uy D, Grattepanche F, Duportail G, Guedon E, Delaunay S, Goergen JL. Cell envelope fluidity modification for an effective glutamate excretion in Corynebacterium glutamicum 2262. Appl. Microbiol. Biotechnol. 2007;76:773–781.10.1007/s00253-007-1046-1
- Seletzky JM, Noack U, Fricke J, Hahn S, Buchs J. Metabolic activity of Corynebacterium glutamicum grown on L-lactic acid under stress. Appl. Microbiol. Biotechnol. 2006;72:1297–1307.10.1007/s00253-006-0436-0
- Inui M, Murakami S, Okino S, Kawaguchi H, Vertes AA, Yukawa H. Metabolic analysis of Corynebacterium glutamicum during lactate and succinate productions under oxygen deprivation conditions. J. Mol. Microbiol. Biotechnol. 2004;7:182–196.10.1159/000079827
- Cocaign-Bousquet M, Lindley ND. Pyruvate overflow and carbon flux within the central metabolic pathways of Corynebacterium glutamicum during growth on lactate. Enzyme Microb. Technol. 1995;17:260–267.10.1016/0141-0229(94)00023-K
- Wendisch PG, Kreutzer C, Kalinowski J, Patek M, Sahm H, Eikmanns BJ. Pyruvate carboxylase from Corynebacterium glutamicum: characterization, expression and inactivation of the pyc gene. Microbiology 1998;144:915–927.10.1099/00221287-144-4-915
- Uy D, Delaunay S, Germain P, Engasser JM, Goergen JL. Instability of glutamate production by Corynebacterium glutamicum 2262 in continuous culture using the temperature-triggered process. J. Biotechnol. 2003;104:173–184.10.1016/S0168-1656(03)00151-2
- Stansen C, Uy D, Delaunay S, Eggeling L, Goergen JL, Wendisch VF. Characterization of a Corynebacterium glutamicum lactate utilization operon induced during temperature-triggered glutamate production. Appl. Environ. Microbiol. 2005;71:5920–5928.10.1128/AEM.71.10.5920-5928.2005