Abstract
Two distinct extracellular lipases were obtained from Penicillium solitum 194A, isolated from domestic compost, and Cladosporium cladosporioides 194B, isolated from dairy wastewater. These alkaline enzymes had molecular masses of 42 and 30 kDa, respectively. The P. solitum 194A lipase differed in mass from previously reported enzyme, indicating that it is a novel lipase, and indicating that penicillia can secrete lipase isoenzymes. The C. cladosporioides lipase was more active on esters of medium-chain acids, whereas the P. solitum lipase was more active on longer chained substrates. The C. cladosporioides enzyme displayed higher thermal stability than the P. solitum lipase, preserving full activity up to 48 °C and showing a T50 (10 min) of 60 °C. Their different catalytic properties and good protein stability should make these enzymes suitable for biotechnological applications. Furthermore, the combined use of these two fungal strains may prove to be valuable in lipid-rich waste management.
Lipolytic enzymes are important biocatalysts due to their numerous applications in industries, such as food, dairy, pharmaceuticals, cosmetics, leather, fine chemicals, detergents, textiles, and paper.Citation1–4) Although they can be obtained from different sources, the most widely exploited enzymes are produced by micro-organisms.
Lipolytic enzymes act on carboxyl esters and include lipases (EC 3.1.1.3) and carboxylesterases (EC 3.1.1.1). Lipases, commonly called true lipases, are more active on the carboxyl ester bonds present in triacylglycerols, liberating long-chain fatty acids (>10 carbons) and glycerol, whereas carboxylesterases preferentially hydrolyze esters with relatively short fatty acid chains (<10 carbons).Citation5) Many lipases are stable in organic solvents, and under certain conditions they can also perform esterification, transesterification, acidolysis, alcoholysis, and aminolysis.Citation6–8) Their reactions usually involve high regio- and/or enantio-selectivity, making these enzymes potential tools for organic chemists.
In spite of their distinctive substrate specificities, lipases and carboxylesterases show similar catalytic mechanisms and molecular structures. They are serine hydrolases endowed with both the canonical catalytic triad (i.e. Ser-His-Asp/Glu) in the active site, and the characteristic α/β-hydrolase fold in their three-dimensional structures.Citation9) Apart from their structural and catalytic features, bacterial and fungal lipases widely differ in their pH and temperature optima, substrate specificity, effector and inhibitor sensitivity, and cellular localization.Citation8,10) Hence, they have various applications as biocatalysts in several industrial fields. In addition to the extensive use of lipases in industry, a promising field for the application of lipolytic enzymes and lipolytic micro-organisms lies in environmental management, in particular in the treatment of waste that is rich in fats, oils, and greases (FOGs).Citation2) FOGs are among the main components of organic matter in wastewater and solid waste of municipal and industrial origin. Unless biologically treated, FOGs are detrimental to the environment, causing land and water pollution. Moreover, they tend to clog drainpipes and corrode sewer pipes.Citation11,12) Thus, pretreating FOG-rich waste with lipolytic biocatalysts can minimize its negative effects by improving removal efficiency and accelerating the degradation process. Several strategies have been developed for this purpose, consisting mainly in the use of hydrolytic microbial enzymes or viable lipase-secreting micro-organisms.Citation11,13,14) One way of enhancing biodegradation is to select micro-organisms with high degradation activity for FOGs and apply them to the removal of pollutants.Citation15)
The purpose of this study was to characterize the extracellular lipases of Penicillium solitum 194A and Cladosporium cladosporioides 194B, two mesophilic microfungi isolated from domestic compost and dairy wastewater samples and selected for their lipolytic activity. Here we report the production, purification, and a biochemical characterization of these two enzymes, which we found to be attractive for biotechnological applications. The enzyme from P. solitum 194A is an additional lipase secreted by the same species, confirming the hypothesis that penicillia can produce extracellular lipase isoforms.
Materials and methods
Reagents
Tween 20 (polyethylene glycol sorbitan monolaurate), Tween 80 (polyethylene glycol sorbitan monooleate), tributyrin (glycerol tributyrate), p-nitrophenol, p-nitrophenyl octanoate (pNPO, C8), p-nitrophenyl decanoate (pNPD, C10), p-nitrophenyl dodecanoate (pNPDD, C12), p-nitrophenyl myristate (pNPM, C14), p-nitrophenyl palmitate (pNPP, C16), and p-nitrophenyl stearate (pNPS, C18) were purchased from Sigma (Schnelldorf, Germany). The olive oil used in the study was purchased from a local market (Pavia, Italy). All other chemicals used were of reagent grade.
Isolation of microfungi
The microfungal strains used in this study, P. solitum 194A (MUT 3450) and C. cladosporioides 194B (MUT 3447), were selected as good lipase producers based on their lipolytic activity on solid media, starting from a strain pool isolated from various sources, including industrial and domestic compost, oleaginous seeds, food, dairy wastewater, and hydrocarbon-contaminated soils.
P. solitum 194A and C. cladosporioides 194B were isolated from domestic compost and dairy industry wastewater (Pavia, Italy), respectively, by the dilution plate method. The final dilutions of the compost (5000-fold)Citation16) and of the dairy wastewater (10-fold and 100-fold) were poured into 140 mm Petri dishes containing potato dextrose agar (PDA, Biokar Diagnostics, Beauvais, France), a generic culture medium used to isolate microfungi, and Tween 20-Agar, a medium that is effective in detecting and isolating lipolytic microfungi.Citation17) Antibiotics (penicillin 70 mg/L and chloramphenicol 50 mg/L) were added to the media to limit bacterial growth. The fungi isolated from the plates were maintained on PDA slants at room temperature.
Taxonomic characterization of fungi
Identification of the isolated strains was performed on the basis of their micro- and macro-morphological features, as evaluated on agar plates containing specific diagnostic media, following PittCitation18) and Domsch et al.Citation19) Specific identifications were confirmed by molecular biology approaches: β-tubulin gene sequence analysis was used to identify the Penicillium strain, and the translation elongation factor 1-α (tef1) gene and internal transcribed spacer region (ITS) sequencing were used for the Cladosporium strain (BCCM/MUCL, (Agro) Industrial Fungi and Yeasts Collection, Louvain-la-Neuve, Belgium; GenBank accession nos. KF444170, KF444171, and KF444172).
Determination of lipolytic activity on solid media
A semi-quantitative evaluation of the lipolytic activity of the strains was carried out on solid media containing the following substrates: Tween 20, Tween 80, tributyrin, and olive oil. Culture basal medium was prepared for both Tweens following Sierra,Citation20) and for tributyrin and olive oil following AndersonCitation21) and Ko et al.Citation22) Tributyrin and olive oil were added to the basal medium to final concentrations of 1 and 0.5% v/v, respectively, and this was homogenized in a Waring blender. Tributyrin and olive oil were better emulsified in these media by adding Tween 80 (0.1 and 0.05% v/v, respectively). The media containing fatty substrates were poured into Petri dishes and inoculated at the center with conidial suspensions (106 spores/mL) of each fungal strain. Lipolytic activity on the Tween media was recognized by the appearance of a precipitation zone around the colony,Citation20) while on tributyrin and olive oil media it was recognized by the presence of a clearing zone. Three replicates were made for each strain, and the plate cultures were incubated at 25 °C. Colony diameter and precipitation/clearing zones were measured on the 7th and 14th incubation days. Yarrowia lipolytica CBS 6124, a known lipolytic yeast,Citation23) was adopted as positive control. Enzyme activity was calculated from the ratio between the diameter of the hydrolysis zone and the colony diameter, and was expressed as the lipolytic activity index.Citation24)
The effect of carbon source on lipase production
The effect of the carbon source on lipase production was examined in submerged culture in media containing 5.0 g/L of peptone, 3.0 g/L of yeast extract, and Tween 80, tributyrin, or olive oil at a final concentration of 1% v/v. The basal medium with the addition of glucose (2% w/v) was used as control. For inoculum preparation, fungi were grown at 25 °C for 72 h in 2 mL of potato dextrose broth. The cultures obtained were inoculated in 300 mL Erlenmeyer flasks containing 100 mL of the fatty media, and were maintained at 25 °C for 7 or 14 d in a rotatory shaker. Then they were filtered through a filter paper (Albet 412, ALBET-Hahnemuehle, Barcelona, Spain) to remove the mycelium, and then passed through sterile 8 μm filters. The culture broth filtrates were used as crude enzyme preparations to determine lipase activity.
Culture conditions and lipase purification
In order to obtain extracellular lipases, each strain was grown at 25 °C for 14 d in two 2 L Erlenmeyer flasks containing 500 mL of broth, with the addition of 1% olive oil in the case of P. solitum 194A, and with 1% Tween 80 in the case of C. cladosporioides 194B. The broth for P. solitum 194A consisted of basal medium (5.0 g/L of peptone and 3.0 g/L of yeast extract). The broth for C. cladosporioides 194B was the medium of Cho et al.Citation25) One liter of solution contained 6.0 g of KH2PO4, 1.0 g of MgSO4x7H2O, 2.0 g of (NH4)2SO4, and 4.0 g of peptone. This allowed us to obtain large quantities of enzymes. At the end of incubation, the mycelium was removed by filtration as previously described, and the culture broth filtrate was centrifuged at 23,000 × g for 90 min, concentrated by ultrafiltration to nearly 100 mL with a 10 kDa cut-off membrane (Amicon—Millipore, Billerica, MA, USA), adjusted to pH 8.5 with 1 M Tris, subdivided into two aliquots, and applied separately to two settled-in-series HiTrap DEAE FF columns (5 mL, GE Healthcare), equilibrated in 50 mM Tris-HCl, pH 8.5. After they were washed with the equilibration buffer, the bound proteins were eluted with a discontinuous KCl gradient (0.0–1.0 M). The flow rate was 2 mL/min. Fractions of 1.5 mL were collected, and those containing lipase activity were pooled, concentrated to 5 mL by ultrafiltration, and loaded onto a HiLoad 16/60 Superdex 75 column (120 mL, GE Healthcare) equilibrated in 50 mM Na-phosphate buffer, pH 7.5. Protein elution was performed with the equilibration buffer at a flow rate of 1.0 mL min−1. Fractions of 2 mL were collected, and those containing lipase activity were pooled together, concentrated by ultrafiltration, and tested for purity on 12% SDS-PAGE. The C. cladosporioides 194B lipase was then run on a HiTrap DEAE FF column (1 mL, GE Healthcare) equilibrated in 50 mM Tris-HCl, pH 8.5, at 1.0 mL min−1. Protein elution was performed with a discontinuous gradient of KCl (0.0–1.0 M). Fractions of 0.3 mL were collected. Fractions with lipase activity were pooled, desalted, and concentrated. The protein concentration was determined following Lowry et al.Citation26)
Measurement of lipase activity
Unless otherwise indicated, lipase activity was determined spectrophotometrically at 37 °C with pNPP as substrate for the P. solitum 194A enzyme and with pNPO as a substrate for the C. cladosporioides 194B enzyme, and by monitoring the p-nitrophenol released at 405 nm.Citation27,28) All substrates were dissolved in 2-propanol. The standard reaction mixture contained 50 mM Na-carbonate, pH 9.5 and 0.5 mM substrate in a final volume of 0.5 mL. The reaction mixture for C. cladosporioides 194B also contained 50 mM Na-phosphate. The reaction was started by adding enzyme solution (0.1–0.5 μg). The reference cuvette contained all the components of the standard reaction mixture with the exception of the enzyme. Under these assay conditions, the molar extinction coefficient of p-nitrophenol was 18,200 M−1 cm−1. One unit was defined as the amount of enzyme that catalyzes the formation of 1 μmol of p-nitrophenol/min under the above conditions.
Determination of the optimum pH value
To determine the optimum pH value, enzyme activity was tested at 0.5 mM substrate while varying the pH through a range 7.0–10.5 with the following buffers (50 mM): PIPES pH 7.0–7.5; HEPES pH 7.5–8.5; Na-phosphate pH 7.0–9.0; and Na-carbonate pH 9.0–10.5. The reaction mixtures for C. cladosporioides 194B also contained 50 mM Na-phosphate. The pH-dependent activity was evaluated with six different substrates (C8–C18). The molar extinction coefficients of p-nitrophenol were determined prior to measurement under each reaction condition.
Thermal stability assays
For thermal stability assays, the enzyme (0.1–0.2 mg/mL) was incubated at given temperatures (30–75 °C) in 50 mM Na-phosphate buffer, pH 7.5. At regular intervals, samples were removed, chilled on ice, and assayed for enzyme activity. Relative activity was calculated as the percentage of enzyme activity before incubation. T50 is defined as the temperature at which the enzyme loses 50% of its activity in 10 min.
Kinetic analysis
For steady-state kinetic analysis, unless otherwise indicated, enzymatic activity was assayed at 37 °C with various concentrations (0.05–0.5 mM) of the various substrates in 50 mM Na-carbonate, pH 9.5 under conditions identical to those described above, except for the substrates used. In this case, the substrates were p-nitrophenyl esters with various acyl chains (C8–C18) as follows: pNPO (C8), pNPD (C10), pNPDD (C12), pNPM (C14), pNPP (C16), and pNPS (C18).
In all cases, the reaction was started by adding the enzyme solution (0.1–0.5 μg). All measurements were taken in triplicate with a Jasco V-550 UV–VIS spectrophotometer (Jasco-Europe, Cremalla, Italy). Kinetic parameters were calculated with Sigma Plot software (SPSS Inc, Chicago, IL). kcat, or turnover number, is the number of catalytic events per second. Km is the substrate concentration at which the reaction velocity is half-maximal. kcat/Km is a measure of how efficiently an enzyme converts substrate to product at sub-saturating substrate concentrations.
Effects of metal ions on enzyme activity
To determine the effects of different ions on enzyme activity, both lipases were assayed as previously reported, but in the presence of various ions at a final concentration of 2 mM. The activity of the enzymes without metallic ions was taken to be 100%.
Results
Lipolytic activity on solid media
Two fungal strains, 194A and 194B, isolated from domestic compost and dairy wastewater, were selected from a strain pool after an evaluation of lipolytic activity on solid media supplemented with four fatty substrates, tributyrin, Tween 20, Tween 80, and olive oil. In all cases, they developed sizeable halos around the fungal colony. On the basis of their micro- and macro-morphological features and distinctive gene sequence analysis (see “Materials and methods”), the two strains were identified as P. solitum 194A and C. cladosporioides 194B. The resulting sequence of the P. solitum 194A β-tubulin gene (459 bp, GenBank acc. no. KF444170) showed the highest identity (99%) and coverage (98%) to sequences of P. solitum strains deposited in the GenBank database. As for the C. cladosporioides 194B analysis, the greatest similarity for the tef1 gene sequence (246 bp, GenBank acc. no. KF444171) was 99% (coverage 99%) with C. cladosporioides deposited sequences, and 100% (coverage 100%) for the ITS sequence (541 bp, GenBank acc. no. KF444172).
To evaluate the trend of enzyme production over time, the two strains were cultured after the first selection for up to 14 d on the same fatty substances. Their lipolytic activities were measured at 7 and 14 d of growth with the lipolytic activity index (hydrolysis zone diameter/colony diameter). The results were then compared with those of the positive control, Y. lipolytica CBS 6124 (Table ).
Table 1. Lipolytic activity index for P. solitum 194A and C. cladosporioides 194B on solid media on various fatty substrates.
The lipolytic activities exhibited by C. cladosporioides 194B increased with growth time, and were generally higher than the activities exhibited by P. solitum 194A and Y. lipolytica CBS 6124 (Table ). The highest activities were displayed towards the synthetic substrates (Tweens and tributyrin). The activity towards the natural substrate (olive oil) was similar to the activity exhibited by P. solitum 194A, although it was detectable only on the 14th day. The lipolytic activities of P. solitum 194A on tributyrin and olive oil were higher than the activities exhibited by control strain, Y. lipolytica CBS 6124. Overall, the lipolytic activities exhibited by P. solitum 194A towards all substrates reached a maximum level after almost 7 d of growth, and were slightly higher with synthetic substrates than with natural ones.
Lipase production in liquid media
The extracellular lipase activity of the two strains was also evaluated spectrophotometrically at 7 and 14 d of culture in liquid media supplemented with various carbon sources (see “Materials and methods”). Both strains exhibited lipase activity only in the presence of olive oil and Tween 80. No activity was recorded in the presence of glucose (control) or tributyrin (Table ). In addition, growth time affected the level of activity: both strains showed maximum lipase activity on the 14th day.
Table 2. Lipase activities of P. solitum 194A and C. cladosporioides 194B in liquid media containing various carbon sources.
As shown in Table , olive oil appeared to be the best substrate for lipase production by P. solitum 194A (0.11 U/mL). This was not a finding: some Penicillium species have been reported to display similar behavior.Citation29) The amount of lipase expressed was generally lower for C. cladosporioides 194B than for P. solitum 194A, both with Tween 80 (0.044 U/mL vs. 0.071 U/mL) and olive oil (0.038 U/mL vs. 0.11 U/mL).
Based on these findings, in order to obtain the enzyme, P. solitum 194A was grown in the presence of olive oil, and C. cladosporioides 194B in the presence of Tween 80.
Lipase purification
The extracellular lipases from P. solitum 194A and C. cladosporioides 194B were purified by the procedures described above in “Materials and methods”. Shortly afterwards, at the end of incubation, culture broth filtrate was applied to a HiTrap DEAE FF column (pH 8.5) (GE Healthcare, Little Chalfont, Buckinghamshire, UK). The elution profiles of the proteins are reported in Fig. (A) and (B). Both lipases eluted at KCl concentrations between 0.2 and 0.4 M. After concentration, the lipase solutions were subjected to size exclusion chromatography on a HiLoad 16/60 Superdex 75 column (GE Healthcare, Little Chalfont, Buckinghamshire, UK). The elution profiles of lipase activity for P. solitum 194A and C. cladosporioides 194B are reported in Fig. (C) and (D), respectively. The major peak of P. solitum 194A lipase activity (Fig. (C)) was just before the major peak of C. cladosporioides 194B (Fig. (D)), indicating that the former had a higher molecular mass. Based on 12% SDS-PAGE, the P. solitum 194A lipase was found to be a pure protein with a molecular mass of nearly 42 kDa (Fig. (E)). The specific activity of the purified enzymes was 44 U/mg.
Fig. 1. Purification of P. solitum 194A and C. cladosporioides 194B lipases.
Note: Elution profiles of P. solitum 194A and C. cladosporioides 194B enzymes from a HiTrap DEAE FF column (panels (A) and (B), respectively) and from a HiLoad 16/60 Superdex 75 column (panels (C) and (D) respectively). Circles represent lipase activity, the continuous line represents the absorbance at 280 nm, and the broken line represents the KCl gradient used for elution from the anionic exchange columns. Silver-stained 12% SDS-PAGE of the two purified lipases is represented in panel (E). Lane M, molecular weight markers; lane P, P. solitum 194A lipase; lane C, C. cladosporioides 194B lipase. Panel (F) represents the final chromatography performed for the C. cladosporioides 194B lipase on a HiTrap DEAE FF column.
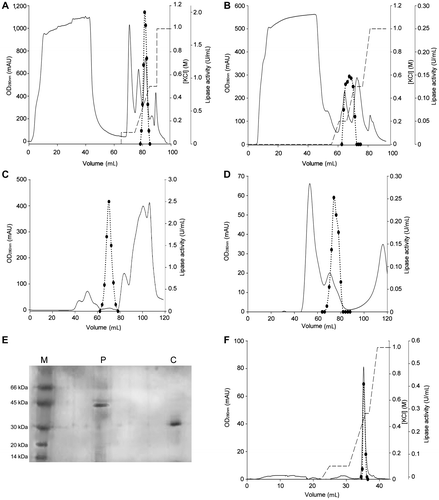
As for the C. cladosporioides 194B lipase, 12% SDS-PAGE of the enzyme obtained by size exclusion chromatography indicated the presence of additional proteins (data not shown). To remove these proteins, the C. cladosporioides 194B lipase was subjected to a further chromatographic step on the HiTrap DEAE FF column. The enzyme eluted at 0.3 M KCl (Fig. (F)). Its apparent molecular mass, as judged by 12% SDS-PAGE (Fig. (E)), approximated 30 kDa. The specific activity of the C. cladosporioides 194B lipase was 12 U/mg. The enzymes were stored at 4 °C in 50 mM Na-phosphate buffer, pH 7.5.
Lipases from both strains were inhibited by serine hydrolase inhibitor PMSF (IC50, 6.5 and 10.5 mM for P. solitum 194A and C. cladosporioides 194B, respectively; data not shown), indicating that they are classic serine hydrolases.Citation8) Moreover, the enzyme from C. cladosporioides 194B required phosphate for enzyme activity and stability (data not shown).
Dependence of activity on pH
The effect of pH on the enzymatic activity of the lipases from P. solitum 194A and C. cladosporioides 194B was investigated with p-nitrophenyl esters of various fatty acids (C8–C18) in a pH range of 7.0–10.5. All substrates were used at a fixed concentration of 0.5 mM. As shown in Fig. , both enzymes were active at alkaline pH, and their maximal activities varied according to the type of substrate used.
Fig. 2. pH activity profiles of the lipases.
Note: The effects of pH on the activity of P. solitum 194A (panel (A)) and C. cladosporioides 194B (panel (B)) lipases were determined at 0.5 mM of p-nitro derivative substrates, with the following buffers (50 mM): PIPES (7.0–7.5), HEPES (7.5–8.5), Na-phosphate (7.0–9.0), and Na-bicarbonate (9.0–10.5). For the assays of the C. cladosporioides 194B lipase, all the buffers contained 50 mM Pi. ● pNPO; ▼ pNPD; ■ pNPDD; ♦ pNPM ▲ pNPP;
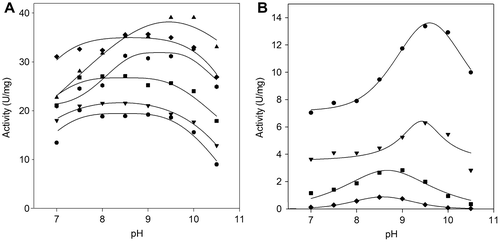
The pH activity profiles fitted the bell-shaped model with plateau, and optimum pH values ranged between 8.5 and 9.7. The P. solitum 194A lipase exhibited higher activities with all substrates than the C. cladosporioides 194B enzyme, with a maximum towards esters of long-chain fatty acids (Fig. (A)). The C. cladosporioides 194B enzyme was more active with esters of medium-chain fatty acids (Fig. (B)), and it failed to show any activity with esters of fatty acids as long as C16 and C18 at any pH value tested.
Thermal stability properties
To evaluate heat stability, the enzymes were incubated at various temperatures for 10 min and assayed for residual activities (Fig. ). The P. solitum 194A lipase was found to be less stable than the C. cladosporioides 194B lipase, showing a steep decline in activity when heated to over 40 °C. Its T50 value was calculated to be 42.4 °C, 18 °C lower than the value for the C. cladosporioides 194B enzyme. The C. cladosporioides 194B lipase preserved full activity up to an incubation temperature of 48 °C, after which it showed gradual inactivation as the temperature increased, until it was completely inactive after 10 min of incubation at 72 °C.
Fig. 3. Thermal stability of the lipases.
Note: The thermal stability of P. solitum 194A (●) and C. cladosporioides 194B (▼) lipases was assessed by subjecting each to heat treatment ranging between 30 and 75 °C. After 10 min of incubation at a given temperature, the enzyme sample was chilled and residual activity was measured. Residual activity was expressed as a percentage of the enzyme activity before incubation.
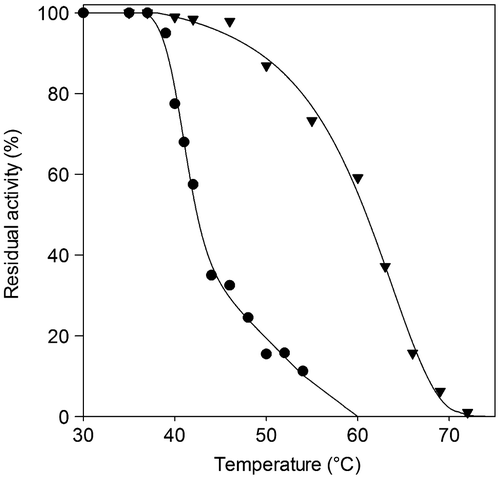
Catalytic properties
Activity as a function of substrate concentration was assayed for six different p-nitrophenyl esters (C8–C18) by spectrophotometric monitoring of the p-nitrophenol released. Each kinetic run was carried out at the pH value at which the enzyme showed maximal activity (Fig. ). The parameters derived from these kinetic runs are summarized in Table . As for the P. solitum 194A lipase (Fig. (A)), Vmax values increased with increasing lengths of the fatty acid carbon chains. Conversely, Km values decreased with increasing lengths of the carbon chains between C8 and C14, but increased when the fatty acid chains were longer than C14. Hence, although the highest activity was towards pNPS (C18; kcat, 39.2 s−1), the optimal substrate for the P. solitum 194A lipase was pNPM (C14; kcat/Km, 959.2 s−1 mM−1). As for the C. cladosporioides 194B lipase (Fig. (B)), both activity and affinity (Table ) decreased with increasing lengths of carbon chain, and pNPO was the optimal substrate (C8; kcat/Km, 260.0 s−1 mM−1). As observed above (Fig. (B)), no activity was detected with pNPP (C16) or pNPS (C18).
Fig. 4. Steady-state kinetics of the lipases.
Note: Steady-state kinetics of the P. solitum 194A (panel (A)) and C. cladosporioides 194B (panel (B)) lipases as a function of various concentrations of p-nitrophenyl esters as substrates. Enzyme activity was assayed at pH 9.5, 37 °C (see “Materials and methods”). ● pNPO; ▼ pNPD; ■ pNPDD; ♦ pNPM ▲ pNPP;
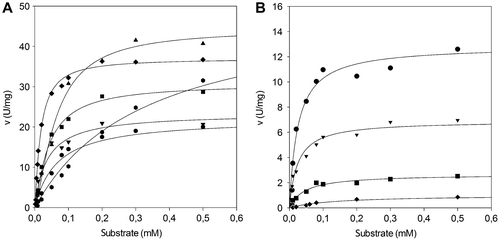
Table 3. Kinetic parameters of the P. solitum 194A and C. cladosporioides 194B lipases.
Effects of metal ions on lipase activity
To evaluate the effects of metal ions on the activity of P. solitum 194A and C. cladosporioides 194B lipases, the enzymes were assayed in the presence of various cations (K+, Li+, Mg2+, Ca2+, Ni2+, Cu2+, Fe2+, and Pb2+) at a final concentration of 2 mM (Fig. ). Cu2+, Fe2+, and Pb2+ severely affected P. solitum 194A lipase activity (inhibition nearly 60%). Li+ and Ni2+ were also inhibitory, although their negative effects were less marked (inhibition about 20%). Compared to the enzyme from P. solitum 194A, C. cladosporioides 194B lipase activity was less affected by the metal ions examined. Cu2+ and Fe2+ caused moderate inactivation (nearly 20%), and Pb2+ was even less effective (inhibition less than 10%). All the other ions left the enzyme activity unchanged, with the exception of Mg2+, which raised lipolytic activity by approximately 30%. In all cases, both inhibition and activation increased with increasing metal ion concentrations (data not shown).
Fig. 5002E Effects of metal ions on lipase activity.
Note: Effects of various metal ions on P. solitum 194A (dark bars) and C. cladosporioides 194B (light bars) lipase activity were determined at a final ion concentration of 2 mM. Enzyme activity was assayed as reported in “Materials and methods” and was expressed as residual activity with respect to the activity of the untreated enzyme.
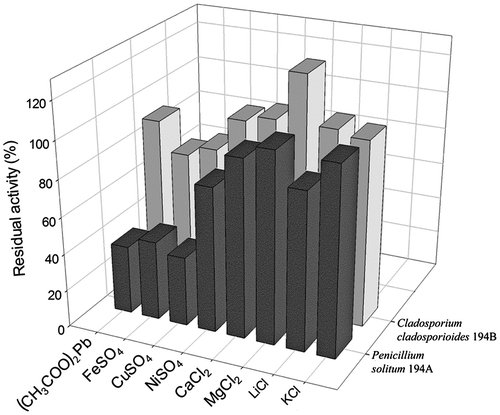
Discussion
Extracellular lipases are among the most promising enzymes that have biotechnological and environmental potential, owing to their peculiar catalytic properties. The ongoing search for new lipases with improved features increasingly involves filamentous fungi, due to their capability to secrete enzymes.Citation4)
Penicillium is one of the most investigated genera,Citation29) being a good producer of various extracellular enzymes as well as a very important decomposer–recycler of organic matter of all types.Citation18,30) Cladosporium has been found to be able to produce cellololytic and xylanolytic enzymes, but little is known about its lipolytic enzymes.Citation25,31)
The present study found that two fungal strains, P. solitum 194A and C. cladosporioides 194B, isolated from domestic compost and dairy wastewater and characterized for their different lipolytic activities on media containing fatty substrates, are good lipase producers. A preliminary evaluation of their activities on solid media, measured by the lipolytic activity index, confirms the importance of this tool in detecting various lipolytic activities in micro-organisms. C. cladosporioides, previously considered to be a suspect lipolytic species,Citation25) was found to be a lipase producer.
Although most biotechnological applications do not require homogeneous enzyme preparations, extensive purification sometimes enables efficient and successful usage in certain industrial processes. Moreover, pure enzymes are needed for a proper assessment of their molecular and kinetic properties and for a better understanding of kinetic mechanisms. Unfortunately, the purification of lipases often requires laborious procedures, making it difficult to obtain highly purified preparations.Citation8,32) The lipases from P. solitum 194A and C. cladosporioides 194B were purified to homogeneity by simple and time-saving procedures (two and three chromatographic steps, respectively), making possible accurate characterizations of their molecular and functional properties.
Most extracellular lipases from micro-organisms appear to be monomeric proteins with molecular masses of about 25–60 kDa. Moreover, some micro-organisms appear to produce at least two types of lipases, characterized by different biochemical and molecular properties.Citation33) The P. solitum 194A and C. cladosporioides 194B lipases showed molecular masses of 42 and 30 kDa, respectively. The value obtained for P. solitum 194A was in line with the most well-known lipases from the genus Penicillium (a molecular mass in the range of 25–43 kDa),Citation29,34) similar to the value obtained for the type II lipases from P. camembertii (38 kDa)Citation5,35,36) and P. cyclopium (40–43 kDa).Citation5,35) Conversely, the value differed from the molecular mass of the type I P. solitum lipase previously reported (27 kDa).Citation5,35,37) This difference is likely to be due to an isoform of the enzyme secreted by this micro-organism. Evidence of an additional lipase secreted by P. solitum is noteworthy, because it supports the suggestion that penicillia are capable of producing more than one extracellular lipase.Citation37,38)
Microbial lipases can act in a wide range of pH, even though the pH optimum for bacterial enzymes is usually neutralCitation39–41) or alkaline,Citation8,42–46) and the pH optimum of fungal enzymes is in a range 4.0–8.0.Citation3) Maximal hydrolytic activity also depends on the type of ester used as substrate.Citation8) The pH optima for the P. solitum 194A and C. cladosporioides 194B lipases fell in an alkaline range (pH 8.5–9.7). The value obtained for P. solitum 194A differed from that obtained for the type I lipase previously reported for the same species (pH optimum, 7.0).Citation37) This indicates that the enzyme we purified was a new lipase. The P. solitum 194A and C. cladosporioides 194B lipases were found to be highly heat-resistant enzymes (full activity preserved up to 40 and 48 °C, respectively, and especially T50 for C. cladosporioides 194B, which equates to 60 °C) (Fig. ), indicating that they were endowed with good protein stability. Usually, fungal lipases are less thermostable than bacterial ones (<40 °C vs. >50 °C),Citation47,48) and thus the new heat-resistant lipases from P. solitum 194A and C. cladosporioides 194B are particularly suitable for industrial applications.
Lipases are by definition enzymes that can hydrolyze long-chain acylglycerols (C ≥ 10), but most known lipases are also active on shorter acyl chain esters as well as on p-nitrophenyl esters, with different efficiencies.Citation29,49) The lipases from P. solitum 194A and C. cladosporioides 194B differed from each other in terms of maximal activity towards a range of p-nitrophenyl esters (C8–C18). Overall, the P. solitum 194A enzyme showed the characteristics of a true lipase by preferentially hydrolyzing ester substrates with a wide range of carboxylic acids, mainly long-chain fatty acid esters. The lipase from C. cladosporioides 194B stood out from the others because of its preference for medium-chain fatty acid esters, appearing to have traits of common carboxylic ester hydrolases.Citation50) Overall, the affinities of both enzymes for their preferred substrates were similar, and significantly higher than the affinities of the most closely corresponding microbial enzymes (Km values, μM vs. mM).Citation33,51) The Km of the P. solitum 194A enzyme towards long-chain fatty acid esters was even lower (up to 14-fold vs. pNPM) than the Km of the P. chrysogenum SNP5 lipase, which was recently reported to be a novel lipase in view of its uncommonly high affinity toward esters.Citation52) Obviously, great emphasis should be placed on comparing the kinetic parameters of lipases due to the different experimental conditions adopted, the type of substrate, or the assay method.Citation10)
The P. solitum 194A and C. cladosporioides 194B lipases are distinguished from other fungal extracellular lipases by their sensitivity to metal ions.Citation8,29,33,51) Surprisingly, the enzyme from C. cladosporioides 194B was stimulated by Mg2+ (nearly 30% activation), whereas the lipase activities of most microfungi usually remain almost unchanged (or even slightly inhibited) under exposure to Mg2+.Citation53,54) On the other hand, the P. solitum 194A enzyme was highly affected by Pb2+, Cu2+, and Fe2+ (about 60% inhibition). Ca2+, which has been found to affect fungal lipase activity and stability,Citation29) had no effect on the activity of these novel lipases.
In conclusion, two fungal strains, P. solitum 194A and C. cladosporioides 194B, were found to be good lipase producers as they displayed different lipolytic activities on media containing fatty substrates. This study confirms lipase production by C. cladosporioides, which was previously treated as a suspect lipolytic species.Citation25) Its enzyme shows preference for medium-chain fatty acid esters and a noticeably higher thermostability than the corresponding enzymes from mesophilic filamentous fungi. P. solitum 194A secretes an additional lipase, which reinforces the suggestion that filamentous fungi can produce various lipolytic enzymes. Similarly to the enzyme from C. cladosporioides 194B, the P. solitum 194A lipase displayed optimal activities at alkaline pH values, but it was more active and showed a preference for long-chain fatty acid esters.
Further research into activity and stability in organic solvents, regio- and stereo-specificity, and surfactants and inhibitors, should assess whether these new lipases can find biotechnological applications, e.g. in the manufacture of fine chemicals and detergents. Moreover, the different features of these novel lipases secreted by P. solitum 194A and C. cladosporioides 194B make the two strains suitable candidates for the treatment of lipid-rich waste, in which they can complement each other in hydrolyzing a broad spectrum of substrates.
Acknowledgments
We thank the BCCM/MUCL (Agro) Industrial Fungi and Yeasts Collection (Louvain-la-Neuve, Belgium) for molecular analysis of the strains studied here. This work was supported by the Lombardy Region ECO-BRICK Project and by Grants from the University of Pavia under the program “Fondi per Ricerca di Ateneo” (FAR).
References
- Jaeger KE, Eggert T. Lipases for biotechnology. Curr. Opin. Biotechnol. 2002;13:390–397.10.1016/S0958-1669(02)00341-5
- Hasan F, Shah AA, Hameed A. Industrial applications of microbial lipases. Enzyme Microb. Technol. 2006;39:235–251.10.1016/j.enzmictec.2005.10.016
- Sharma D, Sharma B, Shukla AK. Biotechnological approach of microbial lipase: a review. Biotechnology. 2011;10:23–40.
- Singh AK, Mukhopadhyay M. Overview of fungal lipase: a review. Appl. Biochem. Biotechnol. 2012;166:486–520.
- Chahinian H, Vanot G, Ibrik A, Rugani N, Sarda L, Comeau LC. Production of extracellular lipases by Penicillium cyclopium purification and characterization of a partial acylglycerol lipase. Biosci., Biotechnol., Biochem. 2000;64:215–222.10.1271/bbb.64.215
- Pandey A, Benjamin S, Soccol CR, Nigam P, Krieger N, Soccol VT. The realm of microbial lipases in biotechnology. Biotechnol. Appl. Biochem. 1999;2:119–131.
- Jaeger KE, Reetz MT. Microbial lipases form versatile tools for biotechnology. Trends Biotechnol. 1998;16:396–403.10.1016/S0167-7799(98)01195-0
- Gupta R, Gupta N, Rathi P. Bacterial lipases: an overview of production, purification and biochemical properties. Appl. Microbiol. Biotechnol. 2004;64:763–781.10.1007/s00253-004-1568-8
- Nardini M, Dijkstra BW. Alpha/beta hydrolase fold enzymes: the family keeps growing. Curr. Opin. Struct. Biol. 1999;9:732–737.10.1016/S0959-440X(99)00037-8
- Arpigny JL, Jaeger KE. Bacterial lipolytic enzymes: classification and properties. Biochem. J. 1999;343:177–183.10.1042/0264-6021:3430177
- Chipasa KB, Mędrzycka K. Behavior of lipids in biological wastewater treatment processes. J. Ind. Microbiol. Biotechnol. 2006;33:635–645.10.1007/s10295-006-0099-y
- Lemus GR, Lau AK. Biodegradation of lipidic compounds in synthetic food wastes during composting. Can. Biosys. Eng. 2002;44:33–39.
- Aikaitè-Stanaitienè J, Grigiškis S, Levišauskas D, Čipinytė V, Baškys E, Kačkytė V. Development of fatty waste composting technology using bacterial preparation with lipolytic activity. J. Environ. Eng. Landsc. Manage. 2010;18:296–305.
- Yan J, Liu S, Hu J, Gui X, Wang G, Yan Y. Enzymatic enrichment of polyunsaturated fatty acids using novel lipase preparations modified by combination of immobilization and fish oil treatment. Bioresour. Technol. 2011;102:7154–7158.10.1016/j.biortech.2011.04.065
- Čipinytė V, Grigiškis S, Baškys E. Selection of fat-degrading microorganisms for the treatment of lipid-contaminated environment. Biologija. 2009;55:84–92.10.2478/v10054-009-0014-3
- Caretta G, Del Frate G, Della Franca P, Guglielminetti M, Mangiarotti AM, Savino E. Mesophilic, thermophilic and keratinophilic fungi in a rice field soil and phylloplane fungi. Bol. Micológ. 1987;3:117–127.
- Gopinath SCB, Hilda A, Anbu P. Extracellular enzymatic activity profiles in fungi isolated from oil-rich environments. Mycoscience. 2005;46:119–126.10.1007/S10267-004-0221-9
- Pitt JI. The genus Penicillium and its teleomorphic states Eupenicillium and Talaromyces. New York (NY): Academic Press; 1979.
- Domsch KH, Gams W, Anderson TH. Compendium of soil fungi. London: Academic Press; 1980.
- Sierra G. A simple method for detection of lipolytic activity of microorganisms and some observations on the influence of the contact between cells and fatty substrates. Anton. van Leeuw. 1957;23:15–22.10.1007/BF02545855
- Anderson JA. The use of tributyrin agar in dairy bacteriology. Ber. lird. Int. Mikrobiol. Kongr. 1939;3:726–728.
- Ko WH, Wang IT, Ann PS. A simple method for detection of lipolytic microorganisms in soils. Soil Biol. Biochem. 2005;37:557–599.
- Fickers P, Marty A, Nicaud JM. The lipases from Yarrowia lipolytica: genetics, production, regulation, biochemical characterization and biotechnological applications. Biotechnol. Adv. 2011;29:632–644.
- Janda K. The lipolytic activity of Thermomyces lanuginosus strains isolated from different natural sources. Int. Biodeterior. Biodegrad. 2005;55:149–152.10.1016/j.ibiod.2004.09.003
- Cho HY, Bancerz R, Ginalska G, Leonowicz A, Cho NS, Ohga S. Culture conditions of psychrotrophic fungus, Penicillium chrysogenum and its lipase characteristics. J. Fac. Agric. Kyushu Univ. 2007;52:281–286.
- Lowry OH, Rosebrough NJ, Farr AL, Randall RJ. Protein measurement with folin phenol reagent. J. Biol. Chem. 1951;193:265–275.
- Winkler UK, Stuckmann M. Glycogen, hyaluronate, and some other polysaccharides greatly enhance the formation of exolipase by Serratia marcescens. J. Bacteriol. 1979;138:663–670.
- Gupta R, Rathi P, Gupta N, Bradoo S. Lipase assays for conventional and molecular screening: an overview. Biotechnol. Appl. Biochem. 2003;37:63–71.
- Li N, Zong MH. Lipases from the genus Penicillium: production, purification, characterization and applications. J. Mol. Catal. B: Enzym. 2010;66:43–54.10.1016/j.molcatb.2010.05.004
- Hamlyn PF, Wales DS, Sagar BF. Extracellular enzymes of Penicillium. In: Peberdy JF, editor. Penicillium and Acremonium. New York (NY): Plenum Press; 1987. p. 245–284.10.1007/978-1-4899-1986-1
- Fapohunda SO. Production of lipase and toxic metabolites by Cladosporium cladosporioides under varied conditions. Mycol. Balc. 2006;3:89–93.
- Saxena RK, Sheoran A, Giri B, Davidson WS. Purification strategies for microbial lipases. J. Microbiol. Methods. 2003;52:1–18.10.1016/S0167-7012(02)00161-6
- Sharma R, Chisti Y, Banerjee UC. Production, purification, characterization and applications of lipases. Biotechnol. Adv. 2001;19:627–662.
- Dheeman DS, Antony-Babu S, Frías JM, Henehan GTM. Purification and characterization of an extracellular lipase from a novel strain Penicillium sp. DS39 (DSM 23773). J. Mol. Catal. B: Enzym. 2011;72:256–262.10.1016/j.molcatb.2011.06.013
- Ibrik A, Chahinian H, Rugani N, Sarda L, Comeau LC. Biochemical and structural characterization of triacylglycerol lipase from Penicillium cyclopium. Lipids. 1998;33:377–383.10.1007/s11745-998-0218-6
- Isobe K, Aumann KD, Schmid RD. A structural model of mono- and diacylglycerol lipase from Penicillium camembertii. J. Biotechnol. 1994;32:83–88.10.1016/0168-1656(94)90123-6
- Gulomova K, Ziomek E, Schrag JD, Davranov K, Cygler M. Purification and characterization of a Penicillium sp. lipase which discriminates against diglycerides. Lipids. 1996;31:379–384.10.1007/BF02522923
- Toskueva EP, Araviĭskiĭ RA, Efimova TP. Cytophysiology of Penicillium solitum: a producer of lipase. Antibiot. Khimioter. 1988;33:647–650.
- Dharmsthiti S, Pratuangdejkul J, Theeragool GT, Luchai S. Lipase activity and gene cloning of Acinetobacter calcoaceticus LP009. J. Gen. Appl. Microbiol. 1998;44:139–145.10.2323/jgam.44.139
- Dharmsthiti S, Luchai S. Production, purification and characterization of thermophilic lipase from Bacillus sp. THL027. FEMS Microbiol. Lett. 1999;179:241–246.10.1111/fml.1999.179.issue-2
- Lee D, Koh Y, Kim K, Kim B, Choi H, Kim D, Suhartono MT, Pyun Y. Isolation and characterization of a thermophilic lipase from Bacillus thermoleovorans ID-1. FEMS Microbiol. Lett. 1999;179:393–400.10.1111/fml.1999.179.issue-2
- Schmidt-Dannert C, Sztajer H, Stöcklein W, Menge U, Schmid RD. Screening, purification and properties of a thermophilic lipase from Bacillus thermocatenulatus. Biochim. Biophys. Acta, Lipids Lipid Metab. 1994;1214:43–53.10.1016/0005-2760(94)90008-6
- Kanwar L, Gogoi BK, Goswami P. Production of a Pseudomonas lipase in n-alkane substrate and its isolation using an improved ammonium sulfate precipitation technique. Bioresour. Technol. 2002;84:207–211.10.1016/S0960-8524(02)00061-5
- Cherif S, Mnif S, Hadrich F, Abdelkafi S, Sayadi S. A newly high alkaline lipase: an ideal choice for application in detergent formulations. Lipids Health Dis. 2011;10:221–228.10.1186/1476-511X-10-221
- Shangguan JJ, Liu YQ, Wang FJ, Zhao J, Fan LQ, Li SX, Xu JH. Expression and characterization of a novel lipase from Aspergillus fumigatus with high specific activity. Appl. Biochem. Biotechnol. 2011;3–4:949–962.
- Lailaja VP, Chandrasekaran M. Detergent compatible alkaline lipase produced by marine Bacillus smithii BTMS 11. World J. Microbiol. Biotechnol. 2013;29:1349–1360.
- Hou CT. pH dependence and thermostability of lipases from cultures from the ARS Culture Collection. J. Ind. Microbiol. 1994;13:242–248.10.1007/BF01569756
- Gutarra MLE, Godoy MG, Maugeri F, Rodrigues MI, Freire DMG, Castilho LR. Production of an acidic and thermostable lipase of the mesophilic fungus Penicillium simplicissimum by solid-state fermentation. Bioresour. Technol. 2009;100:5249–5254.10.1016/j.biortech.2008.08.050
- Jaeger KE, Dijkstra BW, Reetz MT. Bacterial biocatalysts: molecular biology, three-dimensional structures, and biotechnological applications of lipases. Annu. Rev. Microbiol. 1999;53:315–351.10.1146/annurev.micro.53.1.315
- Salameh MA, Wiegel J. Purification and characterization of two highly thermophilic alkaline lipases from Thermosyntropha lipolytica. Appl. Environ. Microbiol. 2007;73:7725–7731.10.1128/AEM.01509-07
- Hasan F, Shah AA, Hameed A. Methods for detection and characterization of lipases: a comprehensive review. Biotechnol. Adv. 2009;27:782–798.
- Kumar S, Mathur A, Singh V, Nandy S, Khare SK, Negi S. Bioremediation of waste cooking oil using a novel lipase produced by Penicillium chrysogenum SNP5 grown in solid medium containing waste grease. Bioresour. Technol. 2012;120:300–304.10.1016/j.biortech.2012.06.018
- Lima VMG, Krieger N, Mitchell DA, Fontana JD. Activity and stability of a crude lipase from Penicillium aurantiogriseum in aqueous media and organic solvents. Biochem. Eng. J. 2004;18:65–71.10.1016/S1369-703X(03)00165-7
- Yu M, Qin S, Tan T. Purification and characterization of the extracellular lipase Lip2 from Yarrowia lipolytica. Process Biochem. 2007;42:384–391.10.1016/j.procbio.2006.09.019