Abstract
Theasaponin E1 (TSE1) has been suggested to have higher biological activity than other saponins present in tea seed. Saponins have recently been considered as a potential chemotherapeutic agent for treating cancer. We examined the anti-angiogenic and anti-obesity properties of TSE1 contributing to anti-cancer efficacy. Treating with a 10 μg/mL concentration of TSE1 completely inhibited tube formation in human umbilical vein endothelial cells (HUVECs). TSE1 showed toxicity toward cancer cells and inhibited in vivo growth of the tumor. The vascular endothelial growth factor (VEGF) receptor complex was suppressed, leading to the inhibition of protein kinase B (Akt) expression and down-regulation of nuclear factor-kappa B (NF-kB) activation. The differentiating 3T3-L1 cells treated with TSE1 had decreased lipid droplet formation measured by Oil Red O staining. Reduced weight was measured in mice fed with a TSE1 plus high-fat diet. The results taken together, and particularly the NF-kB inhibition, suggest that TSE1 may have multi-target action for treating cancer as a novel chemotherapeutic agent.
Graphical Abstract
Bioactive theasaponin E1 (TSE1) suppresses multi-component receptor complex by inhibition of AKT and PI3K, leading to suppressive effect on NF-κB expression.
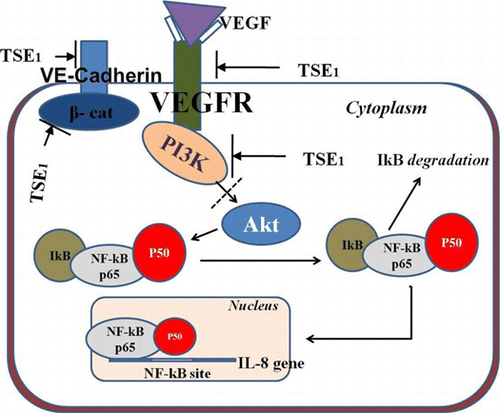
Food and natural bioactive compounds, referred as functional ingredients are of growing interest in the field of nutrition and complementary medicine to prevent chronic illness or as a treatment. Relevant information has been accumulated in the last few decades pointing to the critical role of tea components in having various biological and pharmacological functions ranging from weight loss to cancer.Citation1Citation−Citation3) These health benefits have resulted in tea products and compounds derived from them gaining significant attention, both by the scientific and consumer communities. Green tea (Camellia sinensis) contains many biologically active compounds like saponins, flavonoids, vitamins, and oil minerals, other than polyphenols.Citation4) Tea seed contains more than 10% in dry weight of saponins having various physiological functions including anti-microbial and anti-inflammatory activities.Citation5Citation−Citation7) Kawaguchi et al.Citation8) have reported that tea seed saponin had a 2.4 times lower cytotoxic effect than quillaja saponin, which is a permitted food additive, and that tea seed saponin showed no cytotoxic effect at a dose levels of 50 mg/kg/d in mice. The anti-lipogenic effect of tea saponin has been observed in mice models as inhibiting the pancreatic lipase activity without hemolysis of erythrocytes when orally administered.Citation9)
Neoadipogenesis is accompanied by an angiogenic response (endothelial cell proliferation and vessel sprouting). Therefore, the anti-angiogenic agents constitute a novel therapeutic option for preventing and treating disorders associated with human obesity and angiogenesis.Citation10CitationCitationCitationCitation−Citation15) Angiogenesis involves the formation of new blood vessels by the process of sprouting from pre-existing vasculature. It is an essential component of the metastatic pathway and is controlled under normal physiological process. It occurs in the healthy body to heal wounds and restore blood flow to tissue after injury, during embryonic development, and development of the corpus luteum. Blood vessels are essential to carry oxygen, nutrients, and hormones.Citation16) Angiogenesis and inflammation are necessary for the development of variety of disease conditions such as the proliferation and metastasis of cancer cells, rheumatoid arthritis, and diabetic blindness.Citation17) The vascular endothelial growth factor (VEGF) is one of the most common angiogenic factors regulating normal physiological and tumor angiogenesis.Citation18) VEGFR-2 (KDR/Flk-1) controls endothelial cell morphogenesis and when activated, regulates numerous processes required for the formation of blood capillaries, endothelial cell proliferation, migration, survival, and tube formation.Citation19) The transcription factor nuclear factor-kappa B (NF-kB) controls the expression of a wide variety of tumor angiogenic factors, and NF-kB p65 has been connected to multiple aspects of oncogenesis, including the inhibition of apoptosis by increasing the expression of survival factors.Citation20)
Inhibition of the angiogenesis process could inhibit the proliferation and metastasis of cancer cells. Since chemotherapeutic drugs produce several cytotoxic effects, nutritional and natural supplements are being considered as pharmacological agents to treat or support treatment for cancer by inhibiting angiogenesis and its associated symptoms. The anti-angiogenesis effects of theasaponin E1 (TSE1) have not previously been elucidated. Considering that bioactive TSE1 could inhibit the angiogenesis process, it could have functional properties to inhibit cancer cells. We have investigated in this present study the anti-angiogenesis and anti-obesity properties of TSE1 by in vitro and in vivo methods.
Materials and methods
Preparation of saponin E1
Tea seeds of Camellia sinensis were de-hulled, ground into powder (300 g), and extracted with 70% (v/v) fermented ethanol (1500 mL) by refluxing for 5 h at 60 °C. The suspension was passed through a membrane filter (Buonovino) and concentrated in an SB-100 rotary vacuum evaporator (Eyela). Theasaponin E1 (TSE1) was purified from the concentrated extract (10.05 g) according to the method described by Yamauchi et al.Citation21) The final yield obtained was 2.032 mg and identified by a comparison with 13C-NMR data. TSE1 was diluted and used for the subsequent experiments.
Cell culture
HUVECs (Young Science, Seoul, Korea) were cultured in EBM-2 (Clonetics, USA) supplemented with EGM-2, a SingleQuots kit (Clonetics, USA), at 37 °C in a humidified 5% CO2 incubator until reaching 3–5 passages. Murine (3T3-L1) fibroblast cells (Korean Cell Line Bank, Seoul, Korea) were cultured in DMEM (Gibco, NY, USA) supplemented with NaHCO3 (3.7 g/L), penicillin G (100,000 units/L), streptomycin (100 mg/L), and 10% (v/v) FBS. The cells were differentiated by adding 0.5 μM 3-isobutyl-3-methylxanthine (IBMX), 1 μM dexamethasone (DEX), and 10 μg/mL of insulin.
Human cancer cell lines (stomach, SNU-719; breast HCC-1428; liver, SNU-432; and uterus, SNU-1005) were purchased from Korean Cell Line Bank. The cell lines were cultured in RPMI-1640 containing penicillin (100,000 units/L), streptomycin (100 mg/L), and 10% (v/v) FBS in a humidified 5% CO2 incubator at 37 °C. Cells with 85–90% confluence were used for subsequent experiments.
MTT cytotoxicity assay
The cytotoxic effect of TSE1 on HUVECs was examined by an MTT assay. About 1 × 104 cells were seeded in a 96-well plate for 24 h and treated with TSE1 at various concentrations of 1 to 25 μg/mL for 48 h at 37 °C in a humidified 5% CO2 incubator. A 0.5% MTT solution (Sigma, MO, USA) was added and incubated for 3 h. DMSO (Sigma) was added to dissolve the formazan crystals for 15 min. The absorbance was measured at 540 nm with a microplate reader (Biochrom, Cambridge, UK). The most appropriate concentration (10 μg/mL) was determined and used for this study.
In vitro angiogenesis assay
Twenty-four-well culture plates were coated with 150 μL/well of Matrigel (BD Bioscience, Bedford, MA) which was then allowed to solidify at 37 °C. HUVEC suspensions (2.5 × 104 cells) in a medium were added to the Matrigel-coated wells and subsequently treated with TSE1 after 3 h of incubation. The cells were incubated for 4 h in 5% CO2 at 37 °C. Tube formation was observed and photographed with a digital camera (Nikon, Tokyo, Japan), and the tube lengths of five photographs obtained from a random field of the cell cultures in each well were analyzed by using Scion Image software (NIH, ML, USA).
Inhibition of growth in human cancer cell lines
Cancer cell lines (stomach, SNU-719; breast, HCC-1428; liver, SNU-432; and uterus, SNU-1005) were cultured up to confluency. A fresh medium containing various concentrations of TSE1 was added, and each culture was incubated for 24 h. The cells were then harvested by trypsinization and collected via centrifugation at 1000 rpm for 5 min. The live cells were examined by an MTT assay.
Evaluation of angiogenic protein expression
A Western blot analysis was used to detect the inhibitory effect of TSE1 on angiogenic protein expression. In brief, HUVECs grown to confluence in 100-mm Petri dishes were treated for 24 h with different doses of TSE1, and the cells were washed with a serum-free EBM-2 medium. Control and TSE1-treated cells were kept overnight on starvation, and the cells were then stimulated at 37 °C for 30 min with 50 ng/mL of recombinant human VEGF (Biosource, Invitrogen, USA). The cells were pretreated for 7 min prior to lysis with a dilution of 1:1,000 from an equal volume of a mixture of vandate (100 μmol/L) and hydrogen peroxide (200 μmol/L). The cells were collected with an ice-cold buffer containing Na3VO4 (0.1 mM) and then lysed for 20 min with an ice-cold buffer (of 150 mM NaCl, 10 mM Tris-HCl, pH 7.4, 1% Triton X-100, 1 mM Vandate, 1 mM EDTA, 1 mM FGTA, 0.2 mM PMSF, and 0.5% NP-40). Each cell lysate was centrifuged at 14,000 rpm for 10 min at 4 °C. The supernatant was collected and immunoprecipitated for 12 h at 4 °C with protein G-Sepharose coupled to the polyclonal antibody, VE- cadherin (1 μg/mL). The immunoprecipitate was washed four times with the Na3VO4 (1 mM) lysis buffer and boiled in Laemmli’s sample buffer. The total protein extract was separated by using 7.5% SDS-PAGE gel. The separated protein on the gel was then transferred on to a 0.2-μm nitrocellulose membrane (Bio-Rad, USA). The nitrocellulose membrane was first incubated with 5% skim milk in PBS containing 0.1% Tween-20 and separately probed for 1 h at room temperature with the following antibodies: VEGFR2, PI3 K, β-Catenin, and VE-Cadherin (Biosource, Invitrogen, USA). After 1 h of incubation, the immunoreactive bands were visualized by using horseradish-peroxidase-conjugated antigoat antibodies and then the Western Blotting Luminol reagent (Santa Cruz Biotechnology, USA).
RT-PCR analysis
HUVECs were seeded in a T-25 flask at a density of 1.25 × 105 cells per flask. The cells were maintained in an EBM-2 complete medium up to 70% confluency and then TSE1 was added to the medium to achieve working concentrations of 5, 10, and 25 μg/mL. The cells were incubated for 24 h. Total RNA was extracted from mono-layers of cells by the TRI reagent (Sigma, USA) according to the manufacturer’s instructions. A cDNA synthesis was performed by using RevertAid Premium First Strand cDNA synthesis kit (Thermo Scientific, EU) from 1 μg of total RNA. The level of gene expression was determined from cDNA by PCR for 30 cycles, using Ex Taq Hot Start (Takara, Japan). The sequences of the primers used are shown in Supplemental Table 1; see the Biosci. Biotechnol. Biochem. http://dx.doi.org/10.1080/09168451.2014.893183.
Oil red O staining
The differentiated 3T3-L1 cells were treated with TSE1 and cultured for an additional 2 d. The medium was changed to DMEM containing 10% FBS, 10 μg/mL of insulin, and TSE1. The medium was then replaced once more without insulin. The cells were washed with PBS and fixed with 10% formalin for 1 h. The cells were next washed to dryness with 60% 2-propanol and then incubated for 3 h with an oil red O working solution. The cells were washed four times with distilled water, and the completely dried cells were finally washed with 100% 2-propanol to extract the staining dye from the cells.Citation22) The absorbance of the extracted oil red O solution was measured at 520 nm by using a multi-well plate.
Animal experiments
Four-week-old male C57Bl/6 J-ob/ob mice (SLC, Shizuoka, Japan) were housed under standard conditions (12 h light and dark, at 22 °C). After one week’s acclimatization period, the mice were assigned to two groups of 10 individuals each. The control group was fed with a high-fat diet (45% kcal from fat; D12451 Research Diets, NJ, USA), and the TSE1 group was fed by oral gavage once a day with the high-fat diet plus 10 μg/g body weight of TSE1. The body weights were measured with an electronic scale at 5-d time intervals for 40 d.
Human cancer cells (2 × 106 cells in 0.1 mL of PBS) from each cell line were subcutaneously inoculated into the dorsal part of 6-week-old female nude mice, respectively, designated as each cancer cell group (n = 4). Cancer tissues were produced after 2 weeks of incubation. Ten microgram of TSE1 per body weight (g) was injected into the abdominal cavity of each mouse daily for 4 weeks, and injected directly into the cancer tissue once per week. All the mice in the study were treated according to the National Institutes of Health Guide for the Care and Use of Laboratory Animals, and the experimental protocol was approved by the Chonnam National University Ethical Community for Animal Studies.
Blood analysis
Blood samples were taken from the orbital venous plexus of the mice at the end of the treatment period for an analysis of blood glucose and lipids (total cholesterol, triglyceride, and high-density lipoprotein cholesterol), and for determining aspartate aminotransferase, alanine aminotransferase, and lactate dehydrogenase to assess the liver function. Blood samples were kept on ice, centrifuged, and stored at –80 °C until being analyzed.
Statistical analysis
Data are expressed as the means ± SEM. Statistical analyses were performed by using SPSS 21. Data were analyzed by one-way ANOVA followed by the Tukey-Kramer test for multiple comparisons. The level of significance was set at p < 0.05 for all statistical tests. All experiments were performed three times with similar results, except for the in vivo studies.
Results
Determination of TS1 cytotoxocity in HUVECs
The cytotoxicity of TSE1 in HUVECs was analyzed in a concentration range of 1−25 μg/mL (Fig. ). At 1−5 μg/mL, TSE1 showed no cytotoxicity toward HUVECs with a relative survival of 100% cells. At 10 and 25 μg/mL, 96 and 85% of HUVECs, respectively, survived. The concentration 10 μg/mL, for which survivability was 96%, demonstrated the nontoxic level of TSE1. At the concentration of 50 μg/mL, the cytotoxic effect was higher with lower survival of HUVECs (data not considered for the investigation). At 10 μg/mL, very less cytotoxic effect was measured, so this concentration was taken for further investigation to determine the anti-angiogenic and anti-lipogenic effects of TSE1.
Fig. 1. Cytotoxic effect of TSE1 on HUVECs.
Notes: The cells were incubated for 48 h in a concentration range of 1−25 μg/mL. Cell viability was assessed by an MTT assay, and the absorbance of 96-well plates was measured with a microplate reader at 540 nm. At 1−5 μg/mL, TSE1 showed no cytotoxicity toward HUVEC cells with a relative survival of 100% cells. At 10 μg/mL and 25 μg/mL, 96 and 85% of HUVEC cells, respectively, survived. Values are expressed as the mean ± SEM (n = 3).
*Significantly different from the control at p < 0.05 by the Tukey-Kramer multiple-comparison test.
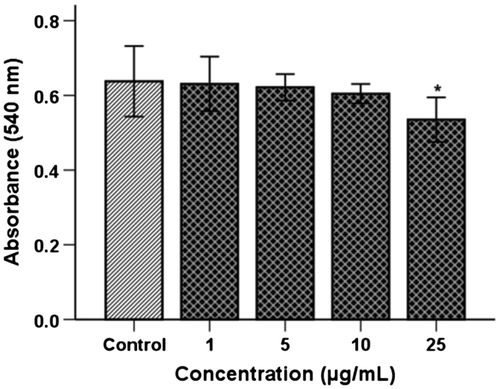
In vitro angiogenesis
The effect of TSE1 on endothelial cells tube formation was examined by a quantitative analysis of the tube length formed on Matrigel. We assessed the maximum safe and nontoxic doses (0.5 LD50) of 10 μg/mL of TSE1 for its anti-angiogenic effect. TSE1 caused massive inhibition of capillary tube formation, even at 5 μg/mL of TSE1 (Fig. (A)). No sprouting was apparent with a treating concentration of 10 μg/mL of TSE1 (Fig. (B)). The anti-angiogenic effect of TSE1 was higher than any other effects observed during this investigation.
Fig. 2. Inhibition of tube formation by TSE1.
Notes: HUVECs (2.5 × 104 cells/well) were plated on Matrigel pre-coated 24-well plates and treated with various concentrations of TSE1 for 4 h. A, The network for tubular structure formation was quantified by measuring the length of the tubular network in five photomicrographs randomly obtained from each well. Values are expressed as the mean ± SEM (n = 3).
* Significantly different from the control at p < 0.05 by the Tukey-Kramer multiple-comparison test. B, Representative images of HUVECs for the control (C) and after treating with 10 μg/mL of TSE1. Tube formation was observed under an inverted phase-contrast microscope and photographed at 100× magnification.
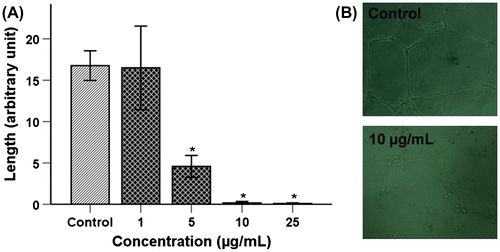
Cancer cell proliferation assay
Figure presents the effect of TSE1 on the proliferation of human cancer cell lines from the breast (Fig. (A)), uterus (Fig. (B)), liver (Fig. (C)), and stomach (Fig. (D)). Cancer cells were treated in the concentration range from 1−10 μg/mL. Proliferation for each cancer cell line was gradually decreased with increasing TSE1 concentration. As already noted, 5 μg/mL of TSE1 (for which no cytotoxicity toward HUVECs was apparent) had a significantly inhibitory effect on proliferation of the cancer cell lines. TSE1 produced the most effective inhibitory effect on stomach cancer cell proliferation (Fig. (D)). Collectively, all cancer cell lines were stressed, morphologically lengthened, pronouncedly inhibited with increasing exposure time, and no cancer cell survived at 10 μg/mL after 24 h of incubation. This result shows that TSE1 had a higher cytotoxic effect on the cancer cell lines.
Fig. 3. Effect of TSE1 on the proliferation of cancer cell lines.
Notes: (A) Breast cancer; (B) uterus cancer; (C) liver cancer; and (D) stomach cancer. Confluent cells were incubated with 1-5 μg/mL of TSE1 for 24 h. The cells were harvested, and live cells were examined by an MTT assay. Values are expressed as the mean ± SEM (n = 3).
*Significantly different from the control at p < 0.05 by the Tukey-Kramer multiple-comparison test.
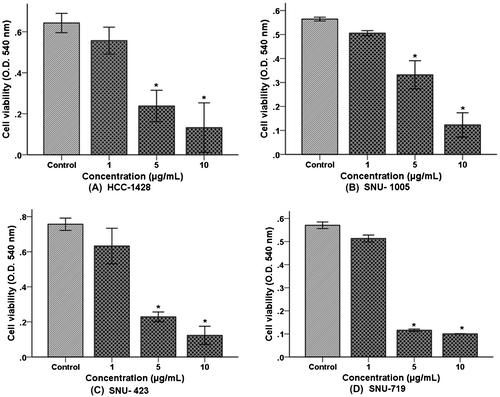
Evaluation of angiogenic protein and mRNA expression
TSE1 inhibited HUVEC tubular formation in Matrigel, so we evaluated the expression of signal molecules VEGFR-2, PI3 K, β-Catenin, and VE-Cadherin, respectively, using anti-signal molecule antibodies, anti-VEGFR-2, anti-PI3 K, anti-β-catenin, and anti-VE-cadherin. VE-Cadherin is considered to be an important mediator of angiogenesis, and we therefore tested its role in cell stimulation by VEGF. The protein expression of VEGFs was significantly increased, and with supplementation by TSE1, the expression of ligand-dependent interaction of VEGFR-2, PI3 K, β-Catenin, and VE-Cadherin was inhibited (Fig. ). Following western blotting, we measured the mRNA expression levels of VEGFR-2, PI3 K, β-catenin, VE-cadherin, Akt, and NF-ĸB, considering β-Actin as the control input, by RT-PCR using gene specific primers. We determined the effect of TSE1 on Akt activity, as the cellular level of pro-angiogenic receptor complex components was reduced. Phosphorylation of Akt is critical for activating the VEGF receptor complex, mainly for PI3-kinase. We also examined the activity of VEGF-induced NF-kB activation (Fig. ). The VEGF receptor complex decreased the phosphorylation of Akt, an important kinase responsible for a variety of signal transduction pathways, further leading to the down-regulation of NF-kB.
Fig. 4. Effects of TSE1 on the interaction of VE-Cadherin with VEGFR-2, PI3-Kinase, and β-Catenin upon cell activation with VEGF.
Notes: The HUVEC extract was immunoprecipitated with VE-cadherin anti-bodies to VEGFR-2 (1), PI3-kinase (2), β-catenin (3), and VE-cadherin (4). The cells were starved during the treatment with TSE1 (5-25 μg/mL) for 24 h before stimulating with VEGF (50 ng/mL).
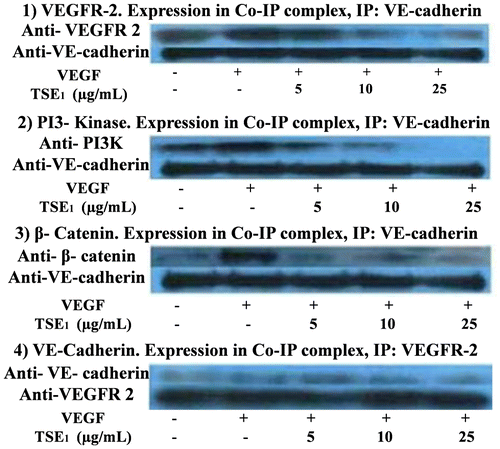
Fig. 5. Representative RT-PCR Analysis for VEGFR-2, PI3 K, β-Catenin, VE-Cadherin, Akt, and NF-kB.
Notes: HUVECs were seeded in T-25 flasks at a density of 1.25 × 105 cells per flask. The 70% confluent cells were treated with 5−25 μg/mL of TSE1 for 48 h. Total RNA was extracted by the TRI reagent, and the expression level of the gene was analyzed by RT-PCR, using a gene-specific primer. β-Actin was taken as the input.
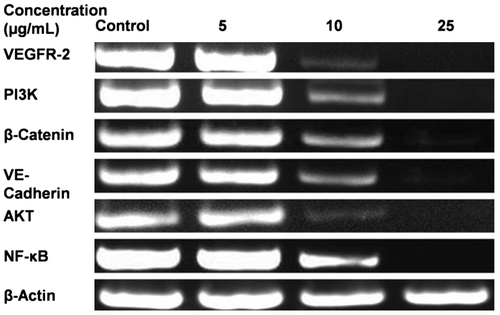
In vivo tumor efficacy study
In the in vivo animal experiment to test the anti-cancer effect of TSE1 on nude mice, tumors of the stomach, liver, uterus, and breast were produced after two weeks of incubation in each designated group. The diameter of the respective tumors was seen decreasing after two week of injecting TSE1 into nude mice. These results show that TSE1 inhibited the proliferation of tumors, thus ceasing tumor. However, the surface of the tumor area was hardened and reduced in size (Fig. ). This reduced size and TSE1 effect were also dependent on the cancer type, thus showing the preventive effect on cancer of TSE1.
Fig. 6. In Vivo animal experiment to test the anti-cancer effect of TSE1 on nude mice.
Note: Human cancer cells (2 × 106 cells in 0.1 mL of PBS) were inoculated subcutaneously into the dorsal part of respective 6-week-old female nude mice as designated for each cancer cell group. Cancer tissues were produced after 2 weeks of incubation. Ten micrograms of TSE1 per body weight (g) was injected into the abdominal cavity of each mouse daily for 4 weeks, and injected directly into the cancer tissue once per week. Surface area of a tumor (mm): (L/2 × W/2) × 3.14, where L is the length of the tumor and W is the width of the tumor. Values are expressed as the mean ± SEM (n = 4).
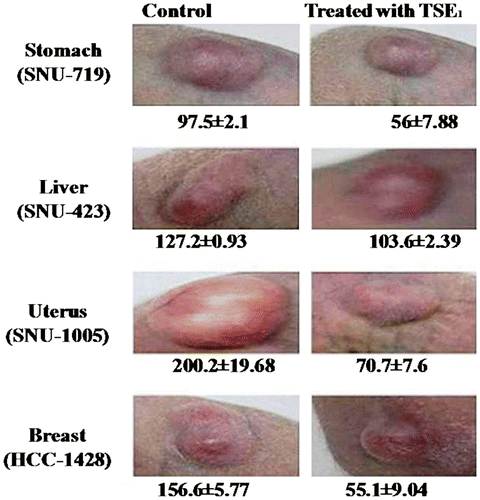
Adipogenesis inhibitory effect by oil red O staining
The differentiating 3T3-L1 cells treated with various concentrations (1, 5, 10, and 25 μg/mL) of TSE1 for 48 h showed, a visible decrease in lipid droplet accumulation measured by oil red O staining as shown in Fig. (A). Relative to the control cells, the TSE1 treatment reduced the intracellular lipid content in a dose-dependent manner, treatment at 10 μg/mL being found to be the most effective dosage for inhibiting adipogenesis. The control and cells with a 1 μg/mL treatment were morphologically similar to each other. With 25 μg/mL, almost no larger lipid droplets could be seen. A similar result was given when quantified with a microplate reader at 520 nm (Fig. (B)). These results suggest that TSE1 inhibited the lipid accumulation in 3T3-L1 adipocytes in a dose-dependent manner.
Fig. 7. Effect of TSE1 on lipid accumulation in 3T3-L1 Cells by oil red O staining.
Notes: After differentiation, 3T3-L1 cells were treated with TSE1 at concentrations of 1−25 μg/mL and then incubated for 48 h. A, Lipid accumulation was stained with an oil red O solution, and the morphological changes were observed by microscopy. Stained 3T3-L1 was observed under an inverted phase-contrast microscope and photographed at x100 magnification. B, The oil red O-stained lipid was quantified with a microplate reader at 520 nm. Values are expressed as the mean ± SEM (n = 3).
*Significantly different from the control at p < 0.05 by the Tukey-Kramer multiple-comparison test.
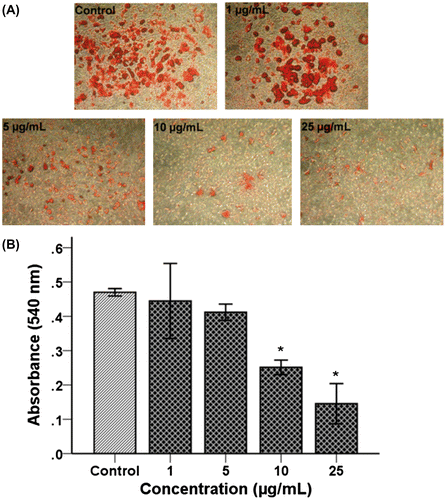
Anti-obesity effect
We measured the body weights of the mice for 40 d with an electronic scale at 5-d time intervals. Feeding the high-fat diet increased the body weight of the mice. The body weight gain was significantly lower for TSE1-treated group after 40 d than for the control group (Fig. ). It was noted that there was significant weight reduction in 2nd and 3rd weeks, and then the weight of test group steadily increased. This result demonstrates that TSE1 induced a body weight loss in comparison to the control group.
Fig. 8. Effect of TSE1 supplementation on diet-induced obesity in mice.
Note: C57Bl/6 J-ob/ob mice were randomly assigned to two groups of 10 each after 1 week of acclimatization. The control group was fed with a high-fat diet, and the TSE1 group was fed with the high-fat diet plus 10 μg/g body weight of TSE1 by oral gavage once a day. (A) Body weight was measured with an electronic scale at 5-d time intervals for 40 d. Values are expressed as the mean ± SEM (n = 10). (B) Weight loss of obese mice fed TSE1 (left), control (right).
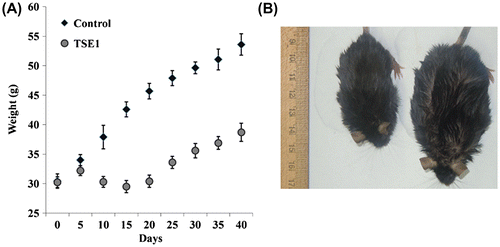
The blood parameters were measured at the end of the treatment period, the plasma lipid content of the TSE1-fed mice being reduced, except for HDL-cholesterol. The AST, ALT, cholesterol, glucose, LDH, triglyceride, and HDL levels of both groups are shown in Supplemental Table 2. Plasma triglyceride was decreased by 1.51-fold in comparison with the positive control groups, total body cholesterol was decreased by 1.34-fold, and HDL cholesterol was increased by 1.41-fold. The plasma glucose level was slightly increased. AST and LDH levels were decreased. The plasma transaminase (ALT) level was dramatically decreased by 1.86-fold.
Discussion
The anti-angiogenic effects of saponins in green tea seed had not previously been studied. Reports on saponins from green tea have ascribed that saponin E1 to have higher biological activity than other tea saponins.Citation5Citation−Citation7) We purified TSE1 by a convenient method already reportedCitation21) and confirmed the product by comparing its 13C-NMR data with those published. The cytotoxicity of saponins occurs through cell membrane permeabilization and associated molecules. The biological actions of saponin are due to the affinity of the aglycone moiety to membrane cholesterols Citation23) and its activity depends upon the amount of cholesterol present on a biological membrane.Citation24) Angiogenesis and obesity are considered risk factors associated with the development of illnesses like cardiovascular disease, diabetes, and cancer. In this study, we first defined the maximum safe and nontoxic level of TSE1 by a cytotoxic assay of HUVECs. We used the nontoxic level of TSE1 and found it to have superior angiogenic inhibitory effects. It is important to note that VEGF induces cell migration, tube formation, and proliferation of endothelial cells which are necessary steps in angiogenesis.Citation25) An in vitro angiogenesis experiment with HUVECs on matrigel showed that TSE1 inhibited the tube formation, thereby indicating an anti-angiogenic mechanism.
VEGF-induced angiogenesis involves the formation of a multi-component receptor complex composed of VEGFR-2, PI3 K, β-Catenin, and VE-Cadherin, which is essential for the downstream activation of Akt and subsequent endothelial cell migration and tube formation.Citation26) TSE1 in our study, inhibited the expression of potential signal molecules VEGFR-2, PI3 K, β-Catenin, and VE-Cadherin, these being responsible for receptor complex formation, which gave us insights into the molecular mechanisms of TSE1 for cancer prevention through the suppression of angiogenesis. Akt regulates NF-kB,Citation27) and NF-kB regulates the expression of a battery of genes involved in tumor cell survival, proliferation, metastasis, invasion, and angiogensis. Constitutively active NF-kB has been found in the tissues of most cancer patients, including those with leukemia, lymphoma, and cancers of the prostate, breast, oral cavity, liver, pancreas, colon, and ovary.Citation4) Targeting the VEGF receptor complex to Akt as well as NF-kB could be a potential means to controlling solid tumor growth and metastasis. Since NF-kB was inhibited, TSE1 has a multi-factorial target which is required to treat a multi-factorial disease like cancer. Angiogenesis and cancer-related disorders are also associated with the expression of NF-kB-regulated IL-8.Citation28) The RT-PCR results for the p65 unit of NF-kB confirmed that TSE1 suppressed the expression of NF-kB. Down-regulation of the angiogenic receptor complex to the NF-kB (RelA) gene supported the hypothesis that TSE1 inhibition of VEGF-induced NF- ĸB activation led to the inhibition of IL-8 mRNA translocation and thus angiogenic protein expression (Fig. ). Following the anti-angiogenenic effect, we examined the effect of TSE1 on cancer cells proliferation and found it to have a significant inhibitory effect on the cancer cell lines, particularly on stomach cancer cells (SNU-719). It is important to note that the 10 μg/mL concentration, at which HUVECs survived, was toxic to cancer cells. The toxic level of TSE1 toward cancer cells was measured at 5 μg/mL. The higher cytotoxicity of TSE1 to the cancer cells might have been due to the presence of a higher level of cholesterol on the membrane of the cancer cells than HUVECs. Cancer cells have been reported to have a more elevated level of cholesterol-rich lipidCitation29,Citation30) than normal cells. This observation in relation to foregoing reportCitation24) provided insight that TSE1 could be used for tumor therapy. The major problem in our study was the dose dependent cytotoxicity, i.e. singularity in toxicity; for example, a higher dose of TSE1 could have a cytotoxic effect even on normal cells. We examined the anti-cancer effect of TSE1 on nude mice, and found that the given concentration of TSE1 reduced the size of tumors and hardened the tumor surface. We observed in the in vivo animal experiment that TSE1 suppressed tumor growth, but did not attack any other normal tissues. This showed the dose-dependent concentration of TSE1 to have a cancer-preventive effect with reduced chances of cytotoxicity to the animals, or that TSE1 may have an anti-cancer effect when treating in combination with other conventional drugs.
Fig. 9. Hypothetical signal pathway of TSE1 anti-angiogenesis effect from VEGFR-2, β-catenin and PI3-K to NF-kB.
Note: These signal molecules were inhibited by TSE1, which lead to inhibition of Akt pathway, subsequently blocked downstream activation and nuclear translocation of NF-ĸB.
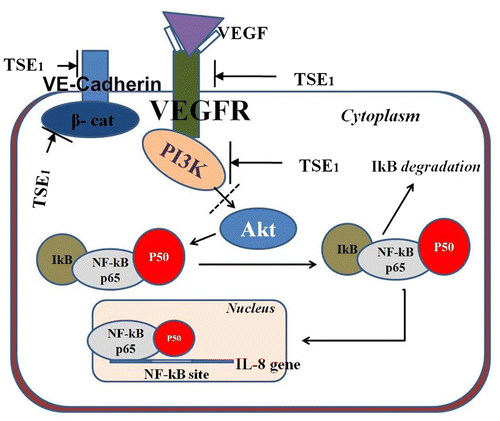
Various reports have suggested that neoplastic and non-neoplastic tissue growth were dependent on angiogenesis.Citation31) Neovascularization and adipogenic related processes occur during prenatal life, and they continue to reciprocally interact throughout adult life via the paracrine signaling system.Citation10) Activated adipocytes generate multiple pro-angiogenic factors like leptin, angiopoietins, HGF, GM-CSF, VEGF, FGF-2, and TGF-beta which, either alone or collectively, stimulate neovascularization during fat mass expansion. We therefore, also examined the adipogenic inhibitory effect of TSE1 under both in vitro and in vivo conditions. The differentiating 3T3-L1 cells treated with various concentrations of TSE1 showed a visible decrease in lipid droplet formation measured by oil Red O staining. TSE1 also decreased the body weight of the mice and had a high anti-obesity effect. The plasma glucose and HDL levels were significantly higher in the TSE1-treated mice than in the control groups. The reduction of AST, ALT, total cholesterol, LDH, and triglyceride indicates the hypolipidemic activity of TSE1. Correlating the results of the cancer cell proliferation assay and lipogenesis assay, and the action mechanism of saponinCitation23) raises the hypothesis that TSE1 can be use for preventing obesity-related inflammation and disorders. It thus seems worth further investigating the mechanism by which TSE1 exerts the anti-angiogenic effect and its role in preventing cancer, and whether it could be incorporated into a diet to intervene in carcinogen metabolism.
In conclusion, the present investigation has shown the anti-angiogenic and anti-obesity potency of TSE1. As TSE1 showed potential activity to prevent cancer cell proliferation and had an adipogenic inhibitory effect, the use of TSE1 as an effective ingredient to cure neoadipogenic-related disorders seems promising. We studied the effect of TSE1 and the results suggest that TSE1 could be applied to modulating angiogenesis and obesity. Further clinical trials and in vivo studies on a routine basis are required to clarify the mechanistic and clinical significance of TSE1.
Supplemental material
The supplemental material for this paper is available at http://dx.doi.org/10.1080/09168451.2014.893183.
Supplementary Fig. 1 caption
Download MS Word (13.3 KB)Supplementary Table 1
Download PDF (149.7 KB)Supplementary Fig. 1
Download MS Power Point (1.3 MB)Acknowledgment
This research was funded by Korean Institute of Planning and Evaluation for Technology in Food, Agriculture, Forestry and Fisheries (112075-3).
Notes
Abbreviations: Akt, Protein kinase B; ALT, Alanine transaminase; AST, Aspartate transaminase; FGF-2, Basic fibroblast growth factor; GM-CSF, Granulocyte-macrophage colony-stimulating factor; HDL, High density lipoprotein; HGF, Hepatocyte growth factor; HUVECs, Human umbilical vein endothelial cells; LDH, Lactate dehydrogenase; NF-kB, Nuclear factor-kappaB; PI3 K, Phosphoinositide 3-kinase;VE-cadherin, Vascular endothelial-cadherin; TGF-beta, Transforming growth factor beta; TSE1, Theasaponin E1; VEGF, Vascular endothelial growth factor; VEGFR-2, Vascular endothelial growth factor receptor-2.
References
- Fujiki F, Suganuma M, Okabe S, Sueoka N, Komori A, Sueoka E, Kozu T, Tada Y, Suga K, Imai K. Cancer inhibition by green tea. Mutat. Res. 1998;402:307–310.10.1016/S0027-5107(97)00310-2
- Lin JK, Lin-Shiau SY. Mechanisms of hypolipidemic and anti-obesity effects of tea and tea polyphenols. Mol. Nutr. Food. Res. 2006;50:211–217.10.1002/(ISSN)1613-4133
- Moyers SB, Kumar NB. Green tea polyphenols and cancer chemoprevention: multiple mechanisms and endpoints for phase II trials. Nutr. Rev. 2004;62:204–211.10.1111/nure.2004.62.issue-5
- Maria L, Urte L, Volker B, Roland B. Antioxidant properties and total phenolics content of green and black tea under different brewing conditions. Z. Lebensm Unters Forsch A. 1999;208:217–220.
- Kohata K, Yamauchi Y, Ujihara T, Horie H. Growth inhibitory activity of Tea-seeds Saponins and Glyphosate to Weed Seedlings. Japan Int. Res. Cent. Agric. Sci. 2004;38:267–270.
- Tomita M, Yamamoto S, Yamaguchi K, Ohigashi H, Yagi T, Kohata K, Berden JA. Theasaponin E1 destroys the salt tolerance of yeast. J. Biosci. Bioeng. 2000;90:637–642.
- Sagesaka Y, Uemura T, Suzuki Y, Sugiura T, Yoshida M, Kyuki K. Antimicrobial and anti-inflammatory actions of tea-leaf saponin. Yakugaku Zasshi. 1996;116:238–243.
- Kawaguchi M, Kato T, Kamada S, Yahata A. Three-month oral repeated administration toxicity study of seed saponins of Thea sinensis L. (Ryokucha saponin) in rats. Food Chem. Toxicol. 1994;32:431–442.10.1016/0278-6915(94)90041-8
- Han LK, Kimura Y, Kawashima M, Takaku T, Taniyama T, Hayashi T, Zeng YN, Okuda H. Anti-obesity effects in rodents of dietary teasaponin, a lipase inhibitor. Int. J. Obes. 2001;25:1459–1464.10.1038/sj.ijo.0801747
- Cao Y. Angiogenesis modulates adipogenesis and obesity. J. Clin. Invest. 2007;117:2362–2368.10.1172/JCI32239
- Crandall DL, Hausman GJ, Kral JG. A review of the microcirculation of adipose tissue: Anatomic, metabolic, and angiogenic perspectives. Microcirculation. 1997;4:211–232.10.3109/10739689709146786
- Hausman GJ, Richardson RL. Adipose tissue angiogenesis. J. Anim. Sci. 2004;82:925–934.
- Neels JG, Thinnes T, Loskutoff DJ. Angiogenesis in an in vivo model of adipose tissue development. FASEB J. 2004;18:983–985.
- Voros G, Maquoi E, Demeullemeester D, Clerx N, Collen D, Lijnen HR. Modulation of angiogenesis during adipose tissue development in murine models of obesity. Endocrinology. 2005;146:4545–4554.10.1210/en.2005-0532
- Christiaens V, Lijnen HR. Angiogenesis and development of adipose tissue. Mol. Cell. Endocrinol. 2010;318:2–9.10.1016/j.mce.2009.08.006
- Folkman J. Angiogenesis. Annu. Rev. Med. 2006;57:1–18.10.1146/annurev.med.57.121304.131306
- Zetter BR. Angiogenesis and metastatis. Annu. Rev. Med. 1998;49:407–427.10.1146/annurev.med.49.1.407
- Ferrara N. VEGF and the quest for tumor angiogenesis factors. Nat. Rev. Cancer. 2002;2:795–803.10.1038/nrc909
- Olsson AK, Dimberg A, Kreuger J, Claesson-Weish L. VEGF receptor signalling—in control of vascular function. Nat. Rev. Mol. Cell Biol. 2006;7:359–371.10.1038/nrm1911
- Karin M, Cao Y, Greten FR, Li ZW. NF-κB in cancer: From innocent bystander to major culprit. Nat. Rev. Cancer. 2002;2:301–310.10.1038/nrc780
- Kitagawa I, Hori K, Motozawa T, Murakami T, Yoshikawa M. Structures of new acylated oleanene-type triterpene oligoglycosides, theasaponins E1 and E2, from the seeds of tea plant, Camellia sinensis (L.) O. Kuntze. Chem. Pharm. Bull. 1998;46:1901–1906.10.1248/cpb.46.1901
- Ramírez-Zacarías JL, Castro-Muñozledo F, Kuri-Harcuch K. Quantitation of adipose conversion and triglycerides by staining intracytoplasmic lipids with Oil red O. Histochemistry. 1992;97:493–497.10.1007/BF00316069
- Glauert AM, Dingle JT, Lucy JA. Action of saponin on biological membranes. Nature. 1962;196:953–955.10.1038/196953a0
- Go-gelein H, Huby A. Interaction of saponin and digitonin with black lipid membranes and lipid monolayers. Biochim. Biophys. Acta. 1984;773:32–38.10.1016/0005-2736(84)90547-9
- Lamalice L, Boeuf FL, Huot J. Endothelial cell migration during angiogenesis. Circ. Res. 2007;100:782–794.10.1161/01.RES.0000259593.07661.1e
- Carmeliet P, Lampugnani MG, Moons L, Breviario F, Compernolle V, Bono F, Balconi G, Spagnuolo R, Oosthuyse B, Dewerchin M, Zanetti A, Angellio A, Mattot V, Nuyens D, Lutgens E, Clotman F, de Ruiter MC, Gittenberger-de Groot A, Poelmann R, Lupu F, Herbert JM, Collen D, Dejana E. Targeted Deficiency or Cytosolic Truncation of the VE-cadherin Gene in Mice Impairs VEGF-Mediated Endothelial Survival and Angiogenesis. Cell. 1999;98:147–157.10.1016/S0092-8674(00)81010-7
- Jeong J, Pise-Masison CA, Radonovich MF, Park HU, Brady JN. Activated Akt regulates NF-kB activation, p53 inhibition and cell survival in HTLV-1-transformed cells. Oncogene. 2005;24:6719–6728.10.1038/sj.onc.1208825
- Karashima T, Sweeney P, Kamat A, Huang S, Kim SJ, Bar-Eli M, McConkey DJ, Dinney CP. Nuclear factor-kappaB mediates angiogenesis and metastasis of human bladder cancer through the regulation of interleukin-8. Clin. Cancer Res. 2003;9:2786–2797.
- Duncan RE, El-Sohemy A, Archer MC. Mevalonate promotes the growth of tumors derived from human cancer cells in vivo and stimulates proliferation in vitro with enhanced cyclin-dependent kinase-2 activity. J. Biol. Chem. 2004;279:33079–33084.10.1074/jbc.M400732200
- Li YC, Park MJ, Ye SK, Kim CW, Kim YN. Elevated Levels of Cholesterol-Rich Lipid Rafts in Cancer Cells Are Correlated with Apoptosis Sensitivity Induced by Cholesterol-Depleting Agents. Am. J. Pathol. 2006;168:1107–1118.10.2353/ajpath.2006.050959
- Doldi N, Bassan M, Gulisano M, Broccoli V, Boncinelli E, Ferrari A. Vascular endothelial growth factor messenger ribonucleic acid expression in human ovarian and endometrial cancer. Gynecol. Endocrinol. 1996;10:375–382.10.3109/09513599609023600