Abstract
The plant steroid hormones brassinosteroids (BRs) play important roles in plant growth and responses to stresses. The up-regulation of pathogen resistance by BR signaling has been analyzed, but the relationship between BR and insect herbivores remains largely unclear. BIL1/BZR1 is a BR master transcription factor known to be involved in the regulation of plant development through work conducted on a gain of function mutation. Here, we analyzed the function of BIL1/BZR1 in response to insect feeding and demonstrated that resistance against thrip feeding was increased in the bil1-1D/bzr1-1D mutant compared to wild-type. We generated Lotus japonicus transgenic plants that over-express the Arabidopsis bil1/bzr1 mutant, Lj-bil1/bzr1-OX. The Lj-bil1/bzr1-OX plants showed increased resistance to thrip feeding. The expression levels of the jasmoninc acid (JA)-inducible VSP genes were increased in both Arabidopsis bil1-1D/bzr1-1D mutants and L. japonicus Lj-bil1/bzr1-OX plants. The resistance to thrip feeding caused by the BIL1/BZR1 gene may involve JA signaling.
Graphical Abstract
Over-Expression mutated bil1/bzr1 that is brassinosteroid master transcription factor gives resistance against the thrips feeding to plants.
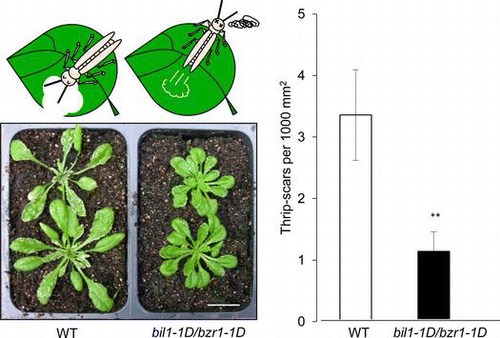
Brassinosteroids (BRs) are plant steroid hormones that regulate a variety of plant developmental processes, e.g., the regulation of cell elongation and division. BRs have also been implicated in plant responses to biotic and abiotic stresses.Citation1) BRs are perceived through the plasma membrane receptor BRASSINOSTEROID INSENSITIVE1 (BRI1) and the co-receptor BRI1-Assosiated Receptor Kinase 1 (BAK1).Citation2−4) The positive regulators BR SIGNALING KINASE 1 (BSK1),Citation5) BRI1-SUPPRESSOR 1 (BSU1),Citation6) and protein phosphatase 2A (PP2A)Citation7) and the negative regulators BRI1-KINASE INHIBITOR 1 (BKI1),Citation8) BRASSINOSTEROID INSENSITIVE 2 (BIN2)Citation9), and 14-3-3Citation10) function downstream of BRI1. BR signaling eventually activates the BRZ-INSENSITIVE-LONG HYPOCOTYL 1/BRASSINAZOLE RESISTANT 1 (BIL1/BZR1)Citation11−13) and BRI1-EMS SUPRESSOR 1 (BES1)Citation14,15) transcription factors.Citation16)
The bil1-1D/bzr1-1D was identified via a chemical biology approach using the BR biosynthesis inhibitor Brz. It is a gain of function mutant in which a point mutation causes the stabilization and accumulation of the BIL1/BZR1 protein in the nucleus. BIL1/BZR1 is considered as a master regulator in the downstream of BR signaling, because BIL1/BZR1 regulates the expression of thousands of BR-responses genes. The bil1-1D/bzr1-1D mutant has the strongest phenotype of the Brz-insensitive long hypocotyl mutants,Citation17,18) and suppressed the BR-insensitive bri1 and bin2 mutant phenotypes.Citation11,13) Recent research has revealed that BIL1/BZR1 is involved in development and in the responses to light, stress, and other hormones including auxin, giberellin, abscisic acid, ethylene (ET), jasmoninc acid (JA), and cytokinin.Citation19)
BR signaling is also involved in innate immunity. BR treatment up-regulated the resistance to plant pathogens, e.g. Pseudomonas syringae pv.tabaci in tobacco and rice.Citation20) BAK1 and BSK1, the BRI1 co-receptor and a BR signaling positive regulator, respectively, were found to be physically associated with the pathogen-associated molecular pattern (PAMPs) receptor FLAGELLIN SENSING2 (FLS2) and caused resistance against to pathogen infection.Citation21−23) Although not only pathogens but also insects are the severe biotic stress against plants, the possible role of BR signaling that involves in resistance to insects has not been well analyzed.
Insect herbivores retard plant growth and decrease crop productivity. Thrips are one of the most hazardous insect herbivores and are known to be a serious pest to a wide range of fruits, vegetables, flowers, and agronomic crops in many countries.Citation24) Thrips are known for not only causing direct plant damage during feeding but also acting as vectors that carry serious plant diseases.Citation25) In many species of thrips, onion thrips (Thrips tabaci) and western flower thrips (Frankliniella occidentalis) have a wide host range compared with the other thrip species.Citation24) To date, the most common method used to prevent thrips is the application of insecticides, but thrips often acquire resistance. Therefore, trial to develop a novel strategy to prevent thrip damage, such as an application of insect-feeding resistance is promised.Citation24,26)
In this study, we analyzed the effects of the Arabidopsis thaliana (hereafter Arabidopsis) BIL1/BZR1 gene, a master trans-acting factor in BR signaling, on onion thrips. We generated transgenic Lotus japonicus plants that over-express the Arabidopsis bi1l/bzr1 and analyzed the physiological function of this gene in development and resistance of onion thrip. The molecular mechanisms of thrip resistance that involves the bil1/bzr1 gene were analyzed and discussed.
Materials and methods
Plant materials and growth conditions
The Arabidopsis ecotype Columbia (Col-0) was used as the wild-type (WT) plant. The seeds were germinated on medium containing 1/2 Murashige and Skoog medium (Duchefa, Haarlem, The Netherlands) and 0.8% phytoagar (Duchefa) with 1.5% sucrose, and 10 days after germination, the seedlings were transferred to soil. The plants were grown at 22 °C under white light (a 16 h light/8 h dark cycle for long-day conditions).
The L. japonicus ecotype Gifu B-129 was used as the WT plant. The sterilized seeds were germinated on 0.8% phytoagar (Duchefa) medium, and 1 week after germination, the seedlings were transferred to soil. The plants were grown at 25 °C under white light (a 16 h light/8 h dark cycle for long-day conditions).
Generating Lj-bil1/bzr1-OX transgenic plants
The mutated bil1/bzr1 was amplified from Arabidopsis bil1-1D/bzr1-1D cDNA with the primers bil1/bzr1-Forward (5′-CACCATGACTTCGGATGGAGCTAC-3′) and bil1/bzr1-Reverse (5′-TCAACCACGAGCCTTCCCAT-3′) and cloned into the pENTR/D-TOPO vector (Invitrogen, Carlsbad, CA, USA). This gene was then transferred into the binary vector pGWB2,Citation27) which contains a CaMV35S promoter, using a Gateway strategy. The resulting p35S-bil1/bzr1 construct was introduced into Agrobacterium tumefaciens C58. The L. japonicus ecotype Gifu hypocotyls were transformed with this Agrobacterium according to the method described by Murakami et al. Citation28) with slight modifications. The transgenic lines were identified according to the results of a PCR assay using primers for the bil1/bzr1 gene (5′-CGACACACTTGTCTACTCCA-3′ and 5′-CCCAACCAGCTTCAACACAA-3′) and were propagated to homozygosity.
Thrip feeding assay
The adult onion thrips, T. tabaci Lindeman, were reared in a mesh cage (20–25 °C) for 2 months. Two-week-old Arabidopsis plants or 40-day-old L. japonicus plants grown in soil were alternately placed in the cage. The scars from thrip feeding were evaluated by counting the number of scars per plant; they were visible as white spots on the leaves 2 weeks after the Arabidopsis plants or the leaves 30 days after the L. japonicus plants were placed in the cage.
Scanning electron microscopy (SEM)
Arabidopsis rosette leaves of approximately the same size were collected from 30-day-old WT and bil1-1D/bzr1-1D plants. The top leaflets of L. japonicus were collected from 50-day-old WT and Lj-bil1/bzr1-OX plants. The adaxial leaf surface of each sample was observed using a Scanning Electron Microscope (SEM, JEOL LSM-5600LV, Tokyo, Japan).
Quantitative real-time PCR
Total RNA was extracted from Arabidopsis and L. japonicus leaves with an RNeasy Plant Mini Kit (Qiagen, Hilden, Germany). The first-strand cDNA was synthesized with PrimeScript (Takara, Kyoto, Japan) and was used as the RT-PCR template. Quantitative real-time PCR was performed according to the instructions provided for the Thermal Cycler Dice (Takara) with the SYBR Premix Ex Taq system (Takara). The sequences of gene-specific primers for RT-PCR were as follows: for bil1/bzr1, 5′-CGCCACCAGTTTCATACCC-3′ and 5′-AGGAGAGGTTGGCACCATAGAG-3′, for VSP2, 5′-GTTAGGGACCGGAGCATCAA-3′ and 5′-AACGGTCACTGAGTATGATGGGT-3′, for PDF1.2, 5′-CCATCATCACCCTTATCTTCGC-3′ and 5′-TGTCCCACTTGGCTTCTCG-3′, for LOX2, -5′ TTGCTCGCCAGACACTTGC-3′ and 5′-GGGATCACCATAAACGGCC-3′, for AOS, 5′-TCTTCTCTTCGCCACGTGC-3′ and 5′-GGT- TATGAACTTGATGACCCGC-3′, for AOC2, 5′-CGGCAAGAAACCAACAGAGC-3′ and 5′-GACCTGCCGTGATTCCCAC-3′, for chiB, 5′-ACGGAAGAGGACCAATGCAA-3′ and 5′-GTTGGCAACAAGGTCAGGGT-3′, for BGL.2, 5′-GCCGACAAGTGGGTTCAAGA-3′ and 5′-AACCCCCCAACTGAGGGTT-3′,Citation29) for LjVSP1B, 5′-TCAAGGCATGGATCATGAAG -3′ and 5′-CTTAAACAATCCTAATATGGCCTGA -3′,Citation30) for LjPDF1.2, 5′-GTGATCAGAGGTGTAAAGCC-3′ and 5′-AGTTATCACTGCACTGGAAG-3′,Citation30) for the constitutively expressed Arabidopsis gene ACT2, 5′-CGCCATCCAAGCTGTTCTC-3′ and 5′-TCACGTCCAGCAAGGTCAAG-3′, and for the constitutively expressed L. japonicus gene, LjATPsyn, 5′-ACATGCTTGCACCATACCAA-3′ and 5′-TCCCCAACTCCAGCAAATAC-3′.Citation31)
Results
The Arabidopsis bil1-1D/bzr1-1D mutant displays resistance to thrip feeding
Plant biotic stress is mainly caused by plant pathogens and insect feeding. The molecular mechanism of BR signaling in plant pathogen defenses has been elucidated, but the relationship between BR signaling and insect feeding has not been well analyzed. To determine the effect of BR signaling on thrip feeding, we compared the feeding damage between an Arabidopsis BR signaling mutant and the WT. As Arabidopsis BIL1/BZR1 is a master transcription factor in the BR signaling pathway, we analyzed the phenotype of bil1-1D/bzr1-1D mutant in response to thrip feeding. Two-week-old bil1-1D/bzr1-1D plants and WT plants were exposed to approximately 100 thrips for 2 weeks, and the scar number on the rosette leaves per plant was counted. In the bil1-1D/bzr1-1D plants, the number of thrip scars per 1000 mm2 of leaf area decreased about one-third to WT on average (Fig. (A) and (B)). The number of plants without scars in bil1-1D/bzr1-1D increased more than twice as much as that of WT plants (Fig. (A) and (C)). Arabidopsis plants that overexpress the BR receptor BRI1-OXCitation32) and the BRI1 deficient bri1-5 mutantCitation33) showed the same scar numbers upon thrip feeding as untreated WT (Fig. (D)). These results suggest that BR signaling, which is enhanced in the bil1-1D/bzr1-1D mutant, plays an important role in thrip feeding resistance in Arabidopsis.
Fig. 1. The Arabidopsis bil1-1D1/bzr1-1D mutant showed resistance to thrip feeding.
Notes: (A) The typical appearance of four-week-old Arabidopsis WT and bil1-1D/bzr1-1D seedlings 2 weeks after thrip feeding. The thrip scars are visible as white spots on the leaves. Scale bar, 2 cm. (B) and (C) The number of thrip scars per 1000 mm2 of leaf area for Arabidopsis WT and bil1-1D1/bzr1-1D plants exposed to thrips for 2 weeks. Thirty plants were analyzed for each genotype. The double asterisks indicate significant differences relative to WT at p < 0.01, based on Student’s t test. B, Overall average of the number of thrip scars. (C) Plants were separated to four groups (0, over 0—for 5, over 5—for 10, over 10) according to scar number and the number of plant in the each group was counted. (D) The number of thrip scars per plant for Arabidopsis WT, BRI1-OX and bri1-5 plants exposed to thrips for 3 weeks. Five plants were analyzed for each genotype.
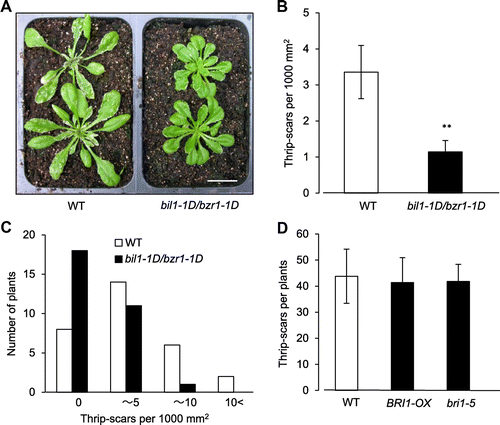
The transformation of the Arabidopsis bil1/bzr1 gene in L. japonicus recapitulated the Brz-insensitive phenotype
L. japonicus is a major model bean plant in agriculture. One amino acid substitution in the Arabidopsis WT BIL1/BZR1 protein caused an increase in its stability. In Arabidopsis, the overexpression of WT BIL1/BZR1 cDNA resulted in a weak phenotype consisting of Brz-insensitive hypocotyl elongation, but the overexpression of mutated bil1/bzr1 cDNA clearly recapitulated the Brz-insensitive long hypocotyl elongation of the bil1-1D/bzr1-1D mutant.Citation11) We used mutated bil1/bzr1 cDNA for the following experiments in L. japonicus. To gain information on thrip feeding resistance that may be relevant to other plant species, mutated bil1/bzr1 cDNA was placed immediately downstream of the CaMV35S promoter and transferred into the WT L. japonicus cultivar Gifu. The transgenic plants were designated as Lj-bil1/bzr1-OX, and T2 homozygous plants were produced by self-pollination and identified using a bil1/bzr1-specific PCR assay. Real-time PCR (RT-PCR) assays showed that bil1/bzr1 was highly expressed in the Lj-bil1/bzr1-OX plants but not in WT L. japonicus (Fig. (A)).
Fig. 2. The transformation of the Arabidopsis bil1/bzr1 gene in L. japonicus recapitulated the brz-insensitive phenotype.
Notes: (A) RT-PCR analysis of Arabidopsis bil1/bzr1 expression in 23-day-old L. japonicus WT and Lj-bil1/bzr1-OX plants. (B) and (C) L. japonicus seedlings grown on medium containing 0, 1.0, and 2.0 μm Brz with 0, 10, and 100 nm BL in the dark for 7 days. (B) Phenotype and (C) Hypocotyl length. Scale bar, 10 mm. The results are presented as the means ± s.e. (n > 17 seedlings). The double and triple asterisks indicate significant differences relative to BL 0 nm treatment plant at p < 0.01 and p < 0.001, respectively, based on Student’s t test. D, Phenotypes and (E) Hypocotyl lengths of L. japonicus WT and Lj-bil1/bzr1-OX seedlings grown on medium containing 0, 0.5, and 1.0 μm Brz in the dark for 7 days. Scale bar, 10 mm. The results are presented as the means ± s.e. (n > 20 seedlings). The triple asterisks indicate significant differences relative to the WT at p < 0.001, based on Student’s t test.
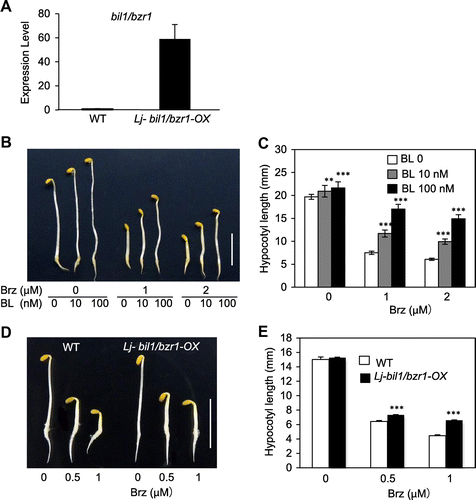
WT Arabidopsis plants usually show hypocotyl elongation in the dark. Brz, an inhibitor of the BR biosynthesis enzyme DWF4, caused shortened hypocotyls in the dark, and the phenotype was rescued by exogenous brassinolide (BL).Citation34,35) The bil1-1D/bzr1-1D Arabidopsis mutant showed a longer hypocotyl than WT in the dark when treated with Brz.Citation11,13) In WT L. japonicus grown in the dark, the Brz treatment reduced the hypocotyl length compared to untreated plants, and the phenotype was rescued by exogenous BL treatment (Fig. (B) and (C)). The Lj-bil1/bzr1-OX seedlings had longer hypocotyls than WT L. japonicus when grown in the dark on medium containing Brz (Fig. (D) and (E)). These results indicated that the overexpression of bil1/bzr1 from Arabidopsis results in the same phenotype in Arabidopsis and L. japonicus.
L. japonicus Lj-bil1/bzr1-OX plants acquired resistance to thrip feeding
To investigate the effect of mutant bil1/bzr1 on thrip feeding, 40-day-old Lj-bil1/bzr1-OX and WT L. japonicus were exposed to approximately 100 thrips for 30 days, and the number of leaf scars per plant was counted. Leaf size of Lj-bil1/bzr1-OX was not significantly different from that of WT L. japonicus. In WT, the number of thrip scars per plant increased about 1.8-fold to Lj-bil1/bzr1-OX plants on average (Fig. (A) and (B)). The number of plants without scars in Lj-bil1/bzr1-OX increased more than twice as much as that of WT (Fig. (A) and (C)). These results indicate that mutant bil1/bzr1 confers resistance to thrip feeding in L. japonicus.
Fig. 3. Lj-bil1/bzr1-OX shows resistance to thrip feeding.
Notes: (A) Typical appearance of six-week-old L. japonicus WT and Lj-bil1/bzr-OX plants 30 days after thrip feeding. Thrip scars are visible as white spots on the leaves. Scale bar, 2 cm. (B) and (C) The number of thrip scars per plant of L. japonicus WT and Lj-bil1/bzr-OX plants exposed to thrips for 30 days. Twenty-four plants were analyzed for the WT and Lj-bil1/bzr1-OX genotypes, respectively. (B) Overall average of the number of thrip scars. (C) Plants were separated to 4 groups (0, over 0—for 5, over 5—for 10, over 10) according to scar number and the number of plant in the each group was counted. The single asterisks indicate significant differences relative to the WT at p < 0.05, based on Student’s t test.
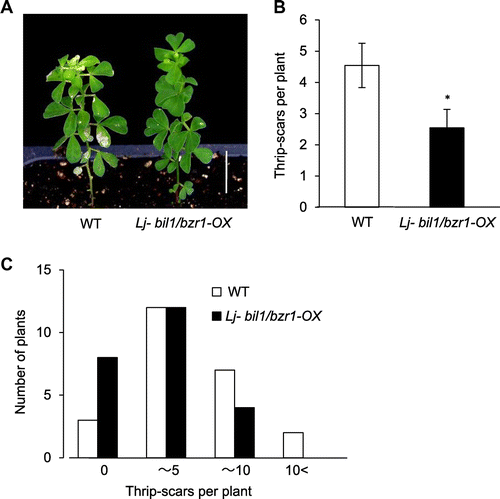
The cell surface structures of the bil1-1D/bzr1-1D mutant and the Lj-bil1/bzr1-OX leaves are similar to those of the WT
Insect feeding starts with contact between the leaf and the insect’s mouth. If the physical structure of the leaf is modified, insect feeding might be affected. In our first experiment to analyze the physiological role of mutant bil1/bzr1 in the insect-feeding response, we observed the leaf surface by SEM. Our data revealed that the shapes and densities of the epidermal leaf cells in the bil1-1D/bzr1-1D mutant and WT Arabidopsis are similar (Fig. (A)). Likewise, the Lj-bil1/bzr1-OX plants and WT L. japonicus showed no significant differences in the epidermal cells (Fig. (B)). Trichomes play an important role in defenses against many insects.Citation36) Observing the trichomes by stereoscopic microscopy revealed that the density, shape, and length of the trichomes in the bil1-1D/bzr1-1D mutant and WT Arabidopsis were similar (Fig. (C)). These observations suggested that the thrip feeding resistance caused by mutant bil1/bzr1 did not result from a difference in leaf surface appearance.
Fig. 4. The epidermal cells and the trichomes on the leaf surfaces of Arabidopsis and L. japonicus are not affected by BIL1/BZR1 over-expression.
Notes: (A) and (B) SEM images of the epidermal cells of the leaf surfaces. Scale bar, 100 μm. (A) 30-day-old Arabidopsis WT and bil1-1D/bzr1-1D plants and (B) 50-day-old L. japonicus WT and Lj-bil1/bzr1-OX plants. (C) Rosette leaves of three-week-old Arabidopsis WT and bil1-1D/bzr1-1D plants. Scale bar, 5.0 mm.
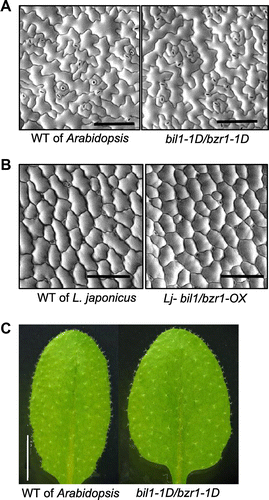
VSP gene expression was increased in mutated bil1/bzr1 plants compared to WT in Arabidopsis and L. japonicus
JA biosynthesis and signaling are induced by plant wounding and insect feeding.Citation37) Because BIL1/BZR1 is a bHLH-type transcription factor, we analyzed the effect of BIL1/BZR1 expression on JA-related gene expression. The inducible genes by JA but not by ET, vegetative storage protein 2 (VSP2) and lipoxygenase 2 (LOX2), were induced by thrips in Arabidopsis.Citation29,38) In bil1-1D/bzr1-1D without thrip administration, the expression levels of VSP2 and LOX2 were increased 3- and 1.4-fold, respectively, compared to WT Arabidopsis (Fig. (A)). Conversely, the expression of the beta-chitinase (chiB) gene, which are induced by thrip feeding as well as JA and ET,Citation29,38) and beta-1,3-glucanase-encoding PR gene (BGL2),Citation29) which are induced by salicylic acid (SA) and insects, were decreased in bil1-1D/bzr1-1D compared to WT Arabidopsis (Fig. (A)). The expression of plant defensin (PDF1.2), which are induced by thrip feeding as well as JA and ET,Citation29,38) was same level between bil1-1D/bzr1-1D and WT Arabidopsis (Fig. (A)).
Fig. 5. The expression of JA- and insect-induced genes in bil1-1D/bzr1-1D and Lj-bil1/bzr1-OX plants.
Notes: (A) RT-PCR analysis of genes known to be inducible by JA, ET, and SA in Arabidopsis in 30-day-old Arabidopsis WT and bil1-1D/bzr1-1D plants. VSP2 and LOX2 (inducible by JA but not by ET), chiB and PDF1.2 (inducible by JA or ET), and BGL2 (inducible by SA) were tested. (B) RT-PCR analysis of Arabidopsis JA biosynthesis genes AOC and AOC2 in 30-day-old Arabidopsis WT and bil1-1D/bzr1-1D plants. (C) RT-PCR analysis of L. japonicus genes that are homologous to Arabidopsis JA-related genes in 23-day-old L. japonicus WT and Lj-bil1/bzr1-OX plants.
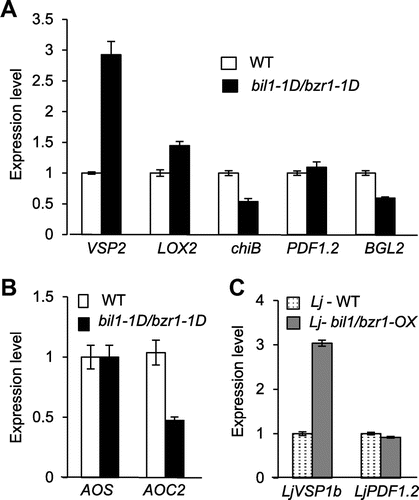
To investigate the relationship between JA biosynthesis and bil1-1D/bzr1-1D, we analyzed the expression of the JA biosynthesis genes, allene oxide synthase (AOS) and allene oxide cyclase 2 (AOC2).Citation39) The AOS gene was expressed at the same level in bil1-1D/bzr1-1D and WT Arabidopsis, and the AOC2 gene was decreased in bil1-1D/bzr1-1D (Fig. (B)). Although the JA content in bil1-1D/bzr1-1D and WT was not analyzed, these results suggest that the bil1/bzr1 mutation might not induce JA biosynthesis.
The L. japonicus genes with similarity to Arabidopsis VSP1 and PDF1.2 are LjVSP1b and LjPDF1.2, respectively, and the expression levels of these genes increased in response to MeJA treatment.Citation30) Arabidopsis VSP1 and VSP2 share high sequence identity and were induced in response to MeJA.Citation40) To analyze the function of mutant bil1/bzr1 in L. japonicus, the expression levels of LjVSP1b and LjPFD1.2 were analyzed. In Lj-bil1/bzr1-OX transformants, the expression of LjVSP1b increased 3-fold, but LjPDF1.2 expression did not increase compared to WT L. japonicus (Fig. (C)). These results are similar to those for Arabidopsis bil1-1D/bzr1-1D and suggest that the resistance to thrip feeding caused by mutant bil1/bzr1 could be related to VSP expression.
Discussion
The functional and molecular mechanisms of BR in plant development have been well analyzed. The relationship between BR and pathogen resistance is being clarified, but the effects of BR on insect feeding have not yet been well explored. We analyzed and proposed possible regulatory mechanisms of BR signaling in insect resistance. BIL1/BZR1 is a master transcription factor in the BR signaling pathway, with roles in BR-induced plant growth responses; it regulates the expression of approximately 3500 Arabidopsis genes.Citation11,19) One amino acid substitution in the WT BIL1/BZR1 protein caused higher stability.Citation11)
Two previous studies have suggested a role for BR signaling in plant defenses against insects. Campos et al.Citation41) showed that the BR biosynthesis-deficient mutant dpy showed enhanced trichome density and defense-related metabolite accumulation in tomatoes and that the mortality of Tuna absoluta larvae on dpy plants was higher than on WT plants. Yang et al.Citation42) showed that silencing BAK1 in Nicotiana attenuata reduced Manduca sexta herbivory-induced JA accumulation but did not analyze the resistance to insect feeding in tobacco BAK1-silenced plants. These reports suggested that BR is involved in plant defense responses to insects, but a detailed molecular mechanism has not been clarified. Our analysis demonstrated that BIL1/BZR1 confers resistance to thrip feeding (Fig. (A)–(C)).
Our experiments indicated that plants overexpressing the BR receptor BRI1 (BRI1-OX)Citation32) and bri1-5 plants did not show thrip feeding resistance (Fig. (D)). bil1-1D/bzr1-1D showed extremely late flowering and may repressed FLOWERING LOCUS D (FLD) expression. However, the bri1 and bak1 mutants showed late flowering. BIL1/BZR1 and BRI1 regulate flowering time through distinct mechanisms.Citation43) Moreover, bil1-1D/bzr1-1D showed a stronger hypocotyl elongation phenotype when treated with Brz than BRI1-OX (date not shown). VSP2 expression did not increase upon BR treatment in WT Arabidopsis (date not shown). These data indicated that the higher stability of the mutant bil1/bzr1 protein might have an ectopic and/or specific function in regulating gene expression.
Legumes (beans) are important sources of food for humans and livestock, and nitrogen fixation by legumes is also critical. L. japonicus is an important model species in legume research because a study of the genome is underway.Citation44) Although many legumes are difficult to transform, L. japonicus is suitable for Agrobacterium-mediated transformation.Citation44)
The garden pea (Pisum sativum L.) lka mutant carries a mutation in PsBRI1, the pea homolog of Arabidopsis BRI1 and is dwarf.Citation45) This result suggests that BRs play important roles in legumes, but little is known regarding the physiological response of L. japonicus to BL or Brz treatment. Our analysis demonstrated that L. japonicus seedlings had shorter hypocotyls upon Brz treatment in the dark compared to untreated plants and that this phenotype was rescued by BL treatment (Fig. (B) and (C)). Transgenic L. japonicus plants overexpressing mutant bil1/bzr1 Arabidopsis cDNA showed longer hypocotyls compared to WT plants when grown in the dark with Brz (Fig. (D) and (E)). This phenotype also occurs in bil1-1D/bzr1-1D Arabidopsis seedlings. These results suggested that BRs and the mutant bil1/bzr1 allele function similarly in L. japonicus and Arabidopsis. Moreover, we demonstrated that L. japonicus plants that overexpress mutant bil1/bzr1 cDNA showed resistance to thrip feeding, similarly to Arabidopsis bil1-1D/bzr1-1D plants (Fig. (A)–(C)). These facts suggest that the higher stability of the mutant bil1/bzr1 protein could function in thrip feeding resistance in multiple plant species. The use of the mutant bil1/bzr1 cDNA may prevent crops from thrip feeding damage.
In this study, we tried to reveal the molecular mechanism of the mutant bil1/bzr1 protein in the resistance to thrip feeding. We first analyzed the leaf structure of the bil1-1D/bzr1-1D mutant and the bil1/bzr1 transformants. No significant differences in either epidermal cell surface appearance or trichome density were observed between bil1-1D/bzr1-1D and WT Arabidopsis (Fig. (A) and (C)). From these results, we hypothesized that the thrip resistance in bil1-1D/bzr1-1D plants was not caused by differences in leaf surface morphology.
In the second experiment, we analyzed gene expression upon thrip feeding because BIL1/BZR1 is a transcription factor (Fig. (A)–(C)). In plant defenses against insects, a signaling network of plant hormones mediated by JA, SA, and ET plays an important role.Citation36) Abe et al.Citation26,29) reported that the expression of VSP2 and LOX2, marker genes in the JA pathway, of chiB and PDF1.2, marker genes in the JA/ET pathway, and of BGL2, a marker gene in the SA pathway in Arabidopsis are induced by thrip feeding. The responses of the JA-insensitive mutant coronatin-insensitive1-1 (coi1-1), ET-insensitive mutants ethylene-insensitive2-1 (ein2-1), ethylene-insensitive3-1 (ein3-1), and the SA-deficient mutant enhanced disease susceptibility16-1 (eds16-1) demonstrated that JA plays a greater role than ET or SA in Arabidopsis in thrip feeding resistance.Citation29)
We then analyzed the expression levels of these marker genes in bil1-1D/bzr1-1D plants without insect feeding. VSP2 was significantly increased, and LOX2 was slightly increased in bil1-1D/bzr1-1D plants compared to WT Arabidopsis. Conversely, the expression level of PDF1.2 was not increased, and the levels of chiB and BGL2 were decreased in bil1-1D/bzr1-1D compared to WT Arabidopsis. In L. japonicus without thrip feeding, the expression of LjVSP1b, but not LjPDF1.2, increased in Lj-bil1/bzr1-OX plants compared to WT. These results implied that the thrip feeding resistance conferred by overexpressing the mutant bil1/bzr1 cDNA was related to JA signaling.
VSPs encode acid phosphatases. Recombinant Arabidopsis VSP2 significantly delayed insect development and increased insect mortality.Citation40) Therefore, VSPs could play a role in the defense against herbivorous insects.Citation36,40) The function of BIL1/BZR1 in thrip feeding might be strongly related to the functions of the JA marker VSP genes.
In WT plants, the expression levels of JA biosynthesis genes and JA-responsive genes were induced after thrip feeding.Citation29) Additionally, JA-treated plants exhibited enhanced feeding resistance compared to untreated plants.Citation29) However, in bil1-1D/bzr1-1D plants, the expression of the JA biosynthesis genes AOS and AOC2 did not increase in comparison to WT. Constitutive activation of BIL1/BZR1 could activate JA signaling without activating JA biosynthesis in plants not exposed to thrips.
The insensitivity of coi1 to root growth inhibition by JA treatment was partially suppressed by a leaky mutation in the BR biosynthesis gene DWF4.Citation46) The overexpression of DWF4 resulted in resistance to root growth inhibition by JA treatment.Citation47) Future research will provide more details of the possible cross-talk mechanism between BR signaling and JA signaling.
Acknowledgments
We thank Dr. Tsuyoshi Nakagawa (Shimane University) for the gift of the gateway vector pGWB2. This work was supported in part by funding from CREST, Japan Science and Technology Agency to T.N. and T.A.
Notes
Abbreviations: BR, brassinosteroid; JA, jasmonic acid; ET, ethylene; SA, salicylic acid; BL, brassinolide; BIL1/BZR1, BRZ-INSENSITIVE-LONG HYPOCOTYL1/BRASSINAZOLE RESISTANT 1; BRI1, BRASSINOSTEROID INSENSITIVE 1; BIN2, BRASSINOSTEROID INSENSITIVE 2; VSP2, vegetative strong protein 2; LOX2, lipoxygenase 2; PDF1.2, plant defensin; BGL2, beta-1,3-glucanase-encoding PR gene; AOS, allene oxide synthase; AOC2, allene oxide cyclase 2.
References
- Bajguz A, Hayat S. Effects of brassinosteroids on the plant responses to environmental stresses. Plant Physiol. Biochem. 2009;47:1–8.10.1016/j.plaphy.2008.10.002
- Li J, Wen J, Lease KA, Doke JT, Tax FE, Walker JC. BAK1, an Arabidopsis LRR receptor-like protein kinase, interacts with BRI1 and modulates brassinosteroid signaling. Cell. 2002;110:213–222.10.1016/S0092-8674(02)00812-7
- Nam KH, Li J. BRI1/BAK1, a receptor kinase pair mediating brassinosteroid signaling. Cell. 2002;110:203–212.10.1016/S0092-8674(02)00814-0
- Kinoshita T, Caño-Delgado A, Seto H, Hiranuma S, Fujioka S, Yoshida S, Chory J. Binding of brassinosteroids to the extracellular domain of plant receptor kinase BRI1. Nature. 2005;433:167–171.10.1038/nature03227
- Tang W, Kim TW, Oses-Prieto JA, Sun Y, Deng Z, Zhu S, Wang R, Burlingame AL, Wang ZY. BSKs mediate signal transduction from the receptor kinase BRI1 in Arabidopsis. Science. 2008;321:557–560.10.1126/science.1156973
- Mora-Garcia S, Vert G, Yin Y, Cano-Delgado A, Cheong H, Chory J. Nuclear protein phosphatases with Kelch-repeat domains modulate the response to brassinosteroids in Arabidopsis. Genes Dev. 2004;18:448–460.10.1101/gad.1174204
- Tang W, Yuan M, Wang R, Yang Y, Wang C, Oses-Prieto JA, Kim TW, Zhou HW, Deng Z, Gampala SS, Gendron JM, Jonassen EM, Lillo C, DeLong A, Burlingame AL, Sun Y, Wang ZY. PP2A activates brassinosteroid-responsive gene expression and plant growth by dephosphorylating BZR1. Nat. Cell. Biol. 2010;13:124–131.
- Wang X, Chory J. Brassinosteroids regulate dissociation of BKI1, a negative regulator of BRI1 signaling, from the plasma membrane. Science. 2006;313:1118–1122.10.1126/science.1127593
- Li J, Nam KH. Regulation of brassinosteroid signaling by a GSK3/SHAGGY-like kinase. Science. 2002;295:1299–1301.
- Gampala SS, Kim TW, He JX, Tang W, Deng Z, Bai MY, Guan S, Lalonde S, Sun Y, Gendron JM, Chen H, Shibagaki N, Ferl RJ, Ehrhardt D, Chong K, Burlingame AL, Wang ZY. An essential role for 14-3-3 proteins in brassinosteroid signal transduction in Arabidopsis. Dev. Cell. 2007;13:177–189.10.1016/j.devcel.2007.06.009
- Wang ZY, Nakano T, Gendron J, He J, Chen M, Vafeados D, Yang Y, Fujioka S, Yoshida S, Asami T, Chory J. Nuclear-localized BZR1 mediates brassinosteroid-induced growth and feedback suppression of brassinosteroid biosynthesis. Dev. Cell. 2002;2:505–513.10.1016/S1534-5807(02)00153-3
- Asami T, Nakano T, Fujioka S. Plant brassinosteroid hormones. Vitam. Horm. 2005;72:479–504.10.1016/S0083-6729(05)72014-8
- He JX, Gendron JM, Sun Y, Gampala SS, Gendron N, Sun CQ, Wang ZY. BZR1 is a transcriptional repressor with dual roles in brassinosteroid homeostasis and growth responses. Science. 2005;307:1634–1638.10.1126/science.1107580
- Yin Y, Wang ZY, Mora-Garcia S, Li J, Yoshida S, Asami T, Chory J. BES1 accumulates in the nucleus in response to brassinosteroids to regulate gene expression and promote stem elongation. Cell. 2002;109:181–191.10.1016/S0092-8674(02)00721-3
- Yin Y, Vafeados D, Tao Y, Yoshida S, Asami T, Chory J. A new class of transcription factors mediates brassinosteroid-regulated gene expression in Arabidopsis. Cell. 2005;120:249–259.10.1016/j.cell.2004.11.044
- Ye H, Li L, Yin Y. Recent advances in the regulation of brassinosteroid signaling and biosynthesis pathways. J. Integr. Plant Biol. 2011;53:455–468.10.1111/jipb.2011.53.issue-6
- Yamagami A, Nakazawa M, Matsui M, Tujimoto M, Sakuta M, Asami T, Nakano T. Chemical genetics reveal the novel transmembrane protein BIL4, which mediates plant cell elongation in brassinosteroid signaling. Biosci. Biotechnol. Biochem. 2009;73:415–421.10.1271/bbb.80752
- Bekh-Ochir D, Shimada S, Yamagami A, Kanda S, Ogawa K, Nakazawa M, Matsui M, Sakuta M, Osada H, Asami T, Nakano T. A novel mitochondrial DnaJ/Hsp40 family protein BIL2 promotes plant growth and resistance against environmental stress in brassinosteroid signaling. Planta. 2013;237:1509–1525.10.1007/s00425-013-1859-3
- Sun Y, Fan XY, Cao DM, Tang W, He K, Zhu JY, He JX, Bai MY, Zhu S, Oh E, Patil S, Kim TW, Ji H, Wong WH, Rhee SY, Wang ZY. Integration of brassinosteroid signal transduction with the transcription network for plant growth regulation in Arabidopsis. Dev. Cell. 2010;19:765–777.10.1016/j.devcel.2010.10.010
- Nakashita H, Yasuda M, Nitta T, Asami T, Fujioka S, Arai Y, Sekimata K, Takatsuto S, Yamaguchi I, Yoshida S. Brassinosteroid functions in a broad range of disease resistance in tobacco and rice. Plant J. 2003;33:887–898.10.1046/j.1365-313X.2003.01675.x
- Chinchilla D, Zipfel C, Robatzek S, Kemmerling B, Nürnberger T, Jones JD, Felix G, Boller T. A flagellin-induced complex of the receptor FLS2 and BAK1 initiates plant defence. Nature. 2007;448:497–500.10.1038/nature05999
- Schulze B, Mentzel T, Jehle AK, Mueller K, Beeler S, Boller T, Felix G, Chinchilla D. Rapid heteromerization and phosphorylation of ligand-activated plant transmembrane receptors and their associated kinase BAK1. J. Biol. Chem. 2010;285:9444–9451.10.1074/jbc.M109.096842
- Shi H, Shen Q, Qi Y, Yan H, Nie H, Chen Y, Zhao T, Katagiri F, Tang D. BR-SIGNALING KINASE1 physically associates with FLAGELLIN SENSING2 and regulates plant innate immunity in Arabidopsis. Plant Cell. 2013;25:1143–1157.10.1105/tpc.112.107904
- Diaz-Montano J, Fuchs M, Nault BA, Fail J, Shelton AM. Onion thrips (Thysanoptera: Thripidae): a global pest of increasing concern in onion. J. Econ. Entomol. 2011;104:1–13.10.1603/EC10269
- Hogenhout SA, Ammar El-D, Hitfield AE, Redinbaugh MG. Insect vector interactions with persistently transmitted viruses. Annu. Rev. Phytopathol. 2008;46:327–359.10.1146/annurev.phyto.022508.092135
- Abe H, Ohnishi J, Narusaka M, Seo S, Narusaka Y, Tsuda S, Kobayashi M. Arabidopsis-thrips system for analysis of plant response to insect feeding. Plant Signal. Behav. 2008;3:446–447.10.4161/psb
- Nakagawa T, Kurose T, Hino T, Tanaka K, Kawamukai M, Niwa Y, Toyooka K, Matsuoka K, Jinbo T, Kimura T. Development of series of gateway binary vectors, pGWBs, for realizing efficient construction of fusion genes for plant transformation. J. Biosci. Bioeng. 2007;104:34–41.10.1263/jbb.104.34
- Murakami Y, Yokoyama H, Fukui R, Kawaguchi M. Down-regulation of NSP2 expression in developmentally young regions of Lotus japonicus roots in response to rhizobial inoculation. Plant Cell Physiol. 2013;54:518–527.10.1093/pcp/pct008
- Abe H, Ohnishi J, Narusaka M, Seo S, Narusaka Y, Tsuda S, Kobayashi M. Function of jasmonate in response and tolerance of Arabidopsis to thrip feeding. Plant Cell Physiol. 2008;49:68–80.10.1093/pcp/pcm168
- Suzuki A, Suriyagoda L, Shigeyama T, Tominaga A, Sasaki M, Hiratsuka Y, Yoshinaga A, Arima S, Agarie S, Sakai T, Inada S, Jikumaru Y, Kamiya Y, Uchiumi T, Abe M, Hashiguchi M, Akashi R, Sato S, Kaneko T, Tabata S, Hirsch AM. Lotus japonicus nodulation is photomorphogenetically controlled by sensing the red/far red (R/FR) ratio through jasmonic acid (JA) signaling. Proc. Natl. Acad. Sci. USA. 2011;108:16837–16842.
- Nakagawa T, Kawaguchi M. Shoot-applied MeJA suppresses root nodulation in Lotus japonicus. Plant Cell Physiol. 2006;47:176–180.
- Wang ZY, Seto H, Fujioka S, Yoshida S, Chory J. BRI1 is a critical component of a plasma-membrane receptor for plant steroids. Nature. 2001;410:380–383.10.1038/35066597
- Noguchi T, Fujioka S, Choe S, Takatsuto S, Yoshida S, Yuan H, Feldmann KA, Tax FE. Brassinosteroid-insensitive dwarf mutants of Arabidopsis accumulate brassinosteroids. Plant Physiol. 1999;121:743–752.10.1104/pp.121.3.743
- Asami T, Min YK, Nagata N, Yamagishi K, Takatsuto S, Fujioka S, Murofushi N, Yamaguchi I, Yoshida S. Characterization of brassinazole, a triazole-type brassinosteroid biosynthesis inhibitor. Plant Physiol. 2000;123:93–100.10.1104/pp.123.1.93
- Asami T, Mizutani M, Fujioka S, Goda H, Min YK, Shimada Y, Nakano T, Takatsuto S, Matsuyama T, Nagata N, Sakata K, Yoshida S. Selective interaction of triazole derivatives with DWF4, a cytochrome P450 monooxygenase of the brassinosteroid biosynthetic pathway, correlates with brassinosteroid deficiency in planta. J. Biol. Chem. 2001;276:25687–25691.10.1074/jbc.M103524200
- War AR, Paulraj MG, Ahmad T, Buhroo AA, Hussain B, Ignacimuthu S, Sharma HC. Mechanisms of plant defense against insect herbivores. Plant Signal. Behav. 2012;7:1306–1320.10.4161/psb
- Howe GA, Jander G. Plant immunity to insect herbivores. Annu. Rev. Plant Biol. 2008;59:41–66.10.1146/annurev.arplant.59.032607.092825
- Lorenzo O, Solano R. Molecular players regulating the jasmonate signalling network. Curr. Opin. Plant Biol. 2005;8:532–540.10.1016/j.pbi.2005.07.003
- Delker C, Stenzel I, Hause B, Miersch O, Feussner I, Wasternack C. Jasmonate biosynthesis in Arabidopsis thaliana—enzymes, products, regulation. Plant Biol. 2006;8:297–306.10.1055/s-2006-923935
- Liu Y, Ahn JE, Datta S, Salzman RA, Moon J, Huyghues-Despointes B, Pittendrigh B, Murdock LL, Koiwa H, Zhu-Salzman K. Arabidopsis vegetative storage protein is an anti-insect acid phosphatase. Plant Physiol. 2005;139:1545–1556.10.1104/pp.105.066837
- Campos ML, de Almeida M, Rossi ML, Martinelli AP. Brassinosteroids interact negatively with jasmonates in the formation of anti-herbivory traits in tomato. J. Exp. Bot. 2009;60:4347–4361.10.1093/jxb/erp270
- Yang DH, Hettenhausen C, Baldwin IT, Wu J. BAK1 regulates the accumulation of jasmonic acid and the levels of trypsin proteinase inhibitors in Nicotiana attenuata’s responses to herbivory. J. Exp. Bot. 2010;62:641–652.
- Zhang Y, Li B, Xu Y, Li H, Li S, Zhang D, Mao Z, Guo S, Yang C, Weng Y, Chong K. The cyclophilin CYP20-2 modulates the conformation of BRASSINAZOLE-RESISTANT1, which binds the promoter of FLOWERING LOCUS D to regulate flowering in Arabidopsis. Plant Cell. 2013;25:2504–2521.10.1105/tpc.113.110296
- Udvardi MK, Tabata S, Parniske M, Stougaard J. Lotus japonicus: legume research in the fast lane. Trends Plant Sci. 2005;10:222–228.10.1016/j.tplants.2005.03.008
- Nomura T, Bishop GJ, Kaneta T, Reid JB, Chory J, Yokota T. The LKA gene is a BRASSINOSTEROID INSENSITIVE 1 homolog of pea. Plant J. 2003;36:291–300.10.1046/j.1365-313X.2003.01863.x
- Ren C, Han C, Peng W, Huang Y, Peng Z, Xiong X, Zhu Q, Gao B, Xie D. A leaky mutation in DWARF4 reveals an antagonistic role of brassinosteroid in the inhibition of root growth by jasmonate in Arabidopsis. Plant Physiol. 2009;151:1412–1420.10.1104/pp.109.140202
- Kim B, Fujioka S, Kwon M, Jeon J, Choe S. Arabidopsis brassinosteroid-overproducing gulliver3-D/dwarf4-D mutants exhibit altered responses to jasmonic acid and pathogen. Plant Cell Rep. 2013;32:1139–1149.10.1007/s00299-012-1381-2