Abstract
12-Oxo-phytodienoic acid (OPDA) is biosynthesized in the octadecanoid pathway and is considered to be a signaling molecule in plants. In Physcomitrella patens, OPDA is induced by bacterial infection and mechanical stress and is known to suppress growth; however, the functional mechanism of OPDA signaling remains elusive. In this study, we performed a proteomic analysis of P. patens treated with OPDA and found that the expression of 82 proteins was significantly altered, with approximately 80% of these proteins being downregulated by OPDA. The identified proteins were mainly categorized as being involved in photosynthesis, metabolism, and protein synthesis, and most of the proteins that were upregulated by OPDA are involved in light-dependent reactions, suggesting that OPDA regulates a function in chloroplasts. Additionally, OPDA induced the expression of an allene oxide cyclase (PpAOC1) in the octadecanoid pathway, demonstrating positive feedback regulation by OPDA in P. patens.
Graphical Abstract
In the moss P. patens, OPDA mainly suppresses the expression of a broad range of proteins, but stimulates the expression of proteins involved in light-dependent reactions of photosynthesis.
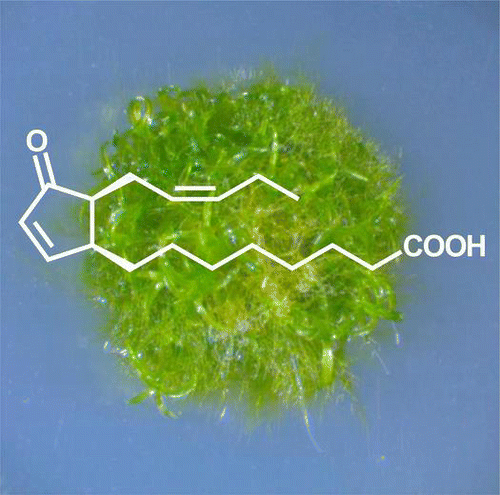
12-Oxo-phytodienoic acid (OPDA) is an important intermediate in the octadecanoid pathway, which leads to the biosynthesis of jasmonic acid (JA), and has been shown to exert both JA-independent and JA-dependent biological activities.Citation1) A transcriptome analysis in Arabidopsis showed that many genes were induced by OPDA, but not by JA.Citation2) OPDA has also recently been shown to be required for embryo development in tomato.Citation3) However, because there is scant information regarding the mechanism of OPDA-dependent physiological events, it is important to elucidate the functions of OPDA as a signaling molecule in plants.
OPDA is biosynthesized via the octadecanoid pathway, which begins with peroxidation at position 13 of α-linolenic acid by lipoxygenase (LOX). The subsequent enzymatic reactions by allene oxide synthase (AOS) and allene oxide cyclase (AOC) generate (+)-cis-OPDA, a naturally occurring form. OPDA is converted to JA after the reduction of the olefin of cyclopentenone and three rounds of β-oxidation, and the conjugation of JA with isoleucine produces jasmonoyl-isoleucine as the active molecule in the JA signaling pathway.Citation4)
Bryophytes are considered to be the first terrestrial plant group and show characteristic physiological processes of early land plants that differ from those of flowering plants. The genome of the moss Physcomitrella patens has been sequenced,Citation5) and the existence of databases and facile gene transformation methods have established P. patens as a model plant.
Many studies examining the functions of plant hormones, such as auxin, cytokinin, and abscisic acid, have been reported in P. patens, and several reports have described the functions of oxylipins, such as OPDA, in this moss. The genes encoding LOX, AOS, and AOC in the octadecanoid pathway have been cloned, and these proteins were shown to exert enzymatic activities similar to those in flowering plants.Citation6–9) Increases in OPDA are induced by bacterial infection and wounding in P. patens,Citation10,11) and OPDA was recently shown to inhibit the growth of P. patens.Citation12) Accordingly, OPDA is suggested to be an important oxylipin involved in P. patens physiology.
In this study, we performed a proteomic analysis of P. patens treated with OPDA. Our data suggest that OPDA plays a role in photosynthesis and energy metabolism in P. patens.
Materials and methods
Plant growth conditions and treatment
The wild-type strain of P. patens subsp. patens was used in this study.Citation13) P. patens was propagated on BCDATG agar medium under continuous white fluorescent light at 25 °C.Citation14) The synthesis of (+)-cis-OPDA was performed according to the method of Kajiwara et al.Citation15) The spectral data of synthetic OPDA are shown in Supplemental Figs. 1–3 (see website).
Analysis of the OPDA concentration in P. patens
P. patens was grown on BCDATG agar medium for 20 days, and the concentration of endogenous OPDA was analyzed. Gametophores (approximately 200 mg) were frozen in liquid nitrogen and extracted with 10 mL of ethanol. The OPDA analysis was performed as described by Matsuura et al. using OPC-8:0-d6 as an internal standard.Citation16,17) For mechanical stress, the gametophores (approximately 200 mg) were soaked in 2 mL of water and subsequently treated with ultrasonication (Sonifier 250, Branson, USA). The samples were directly extracted with 10 mL of ethanol.
Protein extraction
P. patens grown on BCDATG agar for 20 days was treated with 10 μM OPDA for 24 h. The OPDA-treated and -untreated plants were homogenized to a fine powder in liquid nitrogen and subsequently extracted in 5 mL of an ice-cold buffer (50 mM Tris-HCl [pH 7.5] and 1 mM dithiothreitol [DTT]). The resulting protein solution was centrifuged to remove the cellular debris at 15,000 × g for 10 min. The supernatant was used for the proteomic analysis.
Trypsin digestion of proteins
For the in-solution digestion, 50 μg of protein was subjected to TCA precipitation to concentrate the samples and remove any contaminants. The precipitated proteins were rinsed twice with acetone; the dried pellet was resuspended in 50 mM NH4HCO3, reduced with 50 mM DTT, and alkylated with 50 mM iodoacetamide. The proteins were digested using trypsin at a 1:100 enzyme:protein ratio at 37 °C for 16 h. The resulting peptide solution was desalted with SPE C-Tip (Nikkyo Technos, Tokyo, Japan).
Nano-liquid chromatography-tandem MS analysis
A nanospray LTQ XL Orbitrap MS (Thermo Fisher Science, San Jose, CA, USA) was operated in the data-dependent acquisition mode with the installed XCalibur software (ver. 2.0.7, Thermo Fisher Science). Using an Ultimate 3000 nano-LC (Dionex, Germering, Germany), peptides suspended in 0.1% formic acid were loaded onto a C18 PepMap trap column (300 μm ID × 5 mm; Dionex). The peptides were eluted from the trap column, and separation and spraying were performed using 0.1% formic acid in acetonitrile at a flow rate of 200 nL/min with a C18 Tip column (NTTC-360/75-3, Nano-HPLC Capillary Column [75 μm ID × 120 mm]; Nikkyo Technos, Japan) with a spray voltage of 1.8 kV. The elution was performed with a linear acetonitrile gradient (8–30% over 90 min) in 0.1% formic acid. Full-scan mass spectra were acquired in the Orbitrap over 400–1500 m/z, with a resolution of 30,000. A lock mass function was used to obtain high mass accuracy.Citation18) The top six most intense precursor ions were selected for collision-induced fragmentation in the linear ion trap at a normalized collision energy of 35%. Dynamic exclusion was employed within 90 s to prevent the repetitive selection of peptides.Citation19)
Protein identification using MASCOT
The MS/MS spectra acquired were converted to individual DTA files using BioWorks software (version 3.3.1, Thermo Fisher Science). The file format of the DTA files was converted to a MASCOT generic file, and a P. patens database (38,480 protein sequences) and contaminant database (262 protein sequences) were searched using MASCOT software (ver. 2.3.01, Matrix Science). The P. patens database was obtained from Phytozome ver. 8.0 (http://www.phytozome.org/). The parameters used in the MASCOT searches were as follows: cysteine carbamidomethylation was set as a fixed modification, and methionine oxidation was set as a variable modification. Trypsin was specified as the proteolytic enzyme, and one missed cleavage was allowed. The peptide mass tolerance was set at 5 ppm, fragment mass tolerance was set at 0.5 Da, and peptide charge was set at +2, +3, and +4. An automatic decoy database search was performed in the search. The MASCOT results were filtered with MASCOT percolator to improve the accuracy and sensitivity of the peptide identification.Citation20) The false discovery rate for peptide identification in all searches was less than 1.0%, and peptides with a percolator ion score of more than 13 (p < 0.05) were used for protein identification. The MASCOT results were exported in XML format for SIEVE analysis.
Semi-quantitative RT-PCR
P. patens gametophores (approximately 200 mg), which were grown on BCDATG agar medium for 20 days, were treated with 10 μM of (+)-cis-OPDA for 0, 1, 3, 6, 12, and 24 h, and total RNA was extracted. A reverse transcription reaction was performed using M-MLV reverse transcriptase (Invitrogen, USA) according to the manufacturer’s instructions, and semi-quantitative PCR was performed using the resulting cDNA as the template. To estimate the expression level of psaA, psaB, psbC, psbD, and psbE, PCR was optimized for a 12.5 μL reaction mixture containing 6.2 μL of Go Taq® (Promega, USA), 0.3 μL of each 5 μM primer, 0.2 μL of cDNA, and 5.5 μL of Milli-Q water. The reaction conditions were as follows: 95 °C for 2 min (initial denaturation); 95 °C for 30 s, 55 °C for 30 s, and 72 °C for 30 s (suitable cycles for each gene); and a final extension at 72 °C for 10 min. The number of PCR cycles was set to 26 for psaA, 26 for psaB, 37 for psbC, 29 for psbD, 29 for psbD, and 24 for the actin gene.Citation21) To estimate the expression level of PpAOC1, PCR was optimized for a 15 μL reaction mixture containing 7.5 μL of PCR buffer (2×), 0.9 μL of each 5 μM primer, 0.2 μL of cDNA, 1.5 μL of dNTPs, 0.3 μL of KOD-FX neo (Toyobo, Japan), and 3.7 μL of Milli-Q water. The reaction conditions were as follows: 95 °C for 2 min followed by 32 cycles of 98 °C for 10 s, 55 °C for 30 s, and 68 °C for 30 s with a final extension at 68 °C for 10 min. The PCR products were analyzed by gel electrophoresis and visualized by staining with ethidium bromide. The primers used are listed in Supplemental Table 1.
Results and discussion
OPDA is transiently induced by mechanical stress
OPDA is known to be induced by biotic and abiotic stresses and plays an important role in flowering plants, such as Arabidopsis.Citation1) In P. patens, 10 μM OPDA inhibits growth after 20 days of propagation.Citation12) Accordingly, we attempted to determine whether OPDA was also transiently induced by mechanical stress. However, due to the diminutive size of the P. patens gametophore, it is difficult to subject this moss to mechanical stress by cutting or pressing. Therefore, P. patens was subjected to mechanical stress in water by sonication, and the OPDA concentration was then analyzed by UPLC-MS/MS. The results revealed that OPDA was transiently increased after 1 h of mechanical stress and gradually decreased thereafter up to 8 h (Fig. ). The pattern of OPDA concentration in P. patens was similar to that reported in flowering plants.
Fig. 1. Accumulation of OPDA in P. patens after mechanical stress.
Notes: P. patens was grown on BCDATG agar medium for 20 days, and the concentration of endogenous OPDA was analyzed. After P. patens was subjected to mechanical stress for the indicated times, the gametophores were harvested, and the OPDA concentration in each plant was analyzed using UPLC-MS/MS. The values are the means ± SD (n = 5).
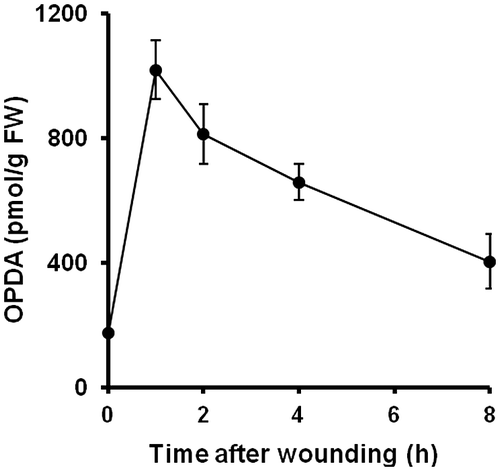
Identification of proteins with altered expression in P. patens treated with OPDA
OPDA was found to inhibit growth at a concentration of 10 μM,Citation12) and mechanical stress transiently elevated the level of OPDA. Considering these data, we collected P. patens grown on BCDATG agar medium for 20 days, predominantly at the gametophore stage, and treated the material with 10 μM OPDA for 24 h. The protein extraction buffer in this study did not contain any denaturation reagents, therefore soluble proteins were mainly included in this analysis. The extracted proteins were digested with trypsin, and the resulting peptides were analyzed by quantitative proteomics using nano-LC-MS/MS. The protein levels were compared based on the area under the curve of each matched peptide using SIEVE software; the number of proteins, which were matched with two peptides, was 1574. A subsequent comparative analysis of the OPDA-treated P. patens and untreated P. patens showed that 82 proteins were differentially expressed, with a significant change (p < 0.05) in quantity of more than 2.5-fold (Table ). To obtain a better understanding of the response to OPDA, the proteins with changes in relative abundance were classified into six groups according to their functions in plants: defense, metabolism, photosynthesis, protein metabolism (protein synthesis, folding and degradation), others, and unknown (Fig. ). Of the 82 proteins exhibiting OPDA-regulated expression in P. patens, only 18 were upregulated by OPDA; therefore, approximately 80% of the OPDA-regulated proteins were downregulated.
Table 1. The identified proteins responsive to OPDA in P. patens.
Upregulated proteins involved in light-dependent reactions and the octadecanoid pathway
Among the 18 proteins upregulated by OPDA, 15 were found to be involved in light-dependent reactionsCitation22); these proteins included chlorophyll a/b-binding proteins, photosystem I reaction center, photosystem I P700 chlorophyll a apoprotein A1 (psaA), photosystem I P700 chlorophyll a apoprotein A2 (psaB), photosystem II 44 kDa protein (psbC), photosystem II protein D2 (psbD), and photosystem II protein V (psbE). These results suggest that OPDA treatment stimulates light-dependent reactions in P. patens. It is interesting that five of the OPDA-induced proteins are encoded by the chloroplast genome; thus, OPDA has the capability of regulating the expression of chloroplast proteins, although the detailed mechanism is not still explored. Moreover, the oxygen produced by light-dependent reactions leads to the production of reactive electrophile species (RES). A recent study suggested that RES and lipid peroxidation are beneficial to plant cells during stress, and RES is considered to upregulate many genes involved in cell survival.Citation23) It is possible that the proteins involved in light-dependent reactions induced by OPDA confer stress adaptation.
AOC, an enzyme involved in the octadecanoid pathway, was induced by OPDA treatment in P. patens; therefore, positive feedback regulation by OPDA also functions in moss. It is suggested that JA, but not OPDA is the signal molecule of a feedback regulation in Arabidopsis.Citation24) Signal transduction to the feedback regulation in P. patens might be different from that in Arabidopsis. The expression of proteins related to light-dependent reactions may lead to the production of reactive oxygen species (ROS), which oxidize polyunsaturated lipids in plastid membranes (such as α-linolenic acid). Given that OPDA biosynthesis is regulated by substrate availability,Citation4) it is reasonable to speculate that OPDA-induced proteins, which are associated with light-dependent reactions, might be involved in supplying oxygen for OPDA production.
Downregulated proteins involved in carbon fixation and glycolysis
OPDA was found to inhibit the synthesis of enzymes involved in carbon fixation, such as ribulose bisphosphate carboxylase (RuBisCO), glyceraldehyde 3-phosphatase dehydrogenase, phosphoribulokinase, and sedoheptulose-bisphosphatase. This result suggests that OPDA represses carbon fixation in P. patens. Photosynthesis occurs in two stages.Citation22) In the first stage, light-dependent reactions trap energy from light and utilize this energy to produce the energy storage molecules ATP and NADPH; in the second stage, light-independent reactions consume ATP and NADPH to assimilate and reduce carbon dioxide for carbohydrate production. Therefore, the OPDA-induced downregulation of proteins involved in carbon fixation might suppress carbohydrate production and energy consumption for adaptation to adverse environmental conditions. In association with a decrease in the expression of proteins related to carbon fixation, it is likely that OPDA depresses the expression of enzymes related to glycolysis, such as pyruvate kinase and fructose-6-phosphate 1-phosphotransferase.
Downregulated proteins involved in protein synthesis
OPDA was found to suppress the expression of ribosomal proteins, RNA-binding proteins, translation initiation factors, elongation factors, and a splicing factor. These proteins participate in the translation of RNA, RNA splicing, and editing,Citation25,26) thereby suggesting that OPDA suppresses protein synthesis. Furthermore, these proteins may exert a level of developmental control over cellular fate and function. A chaperonin, late embryogenesis abundant (LEA), two peptidyl-prolyl cis-trans isomerases, and proteolytic enzymes including an aminopeptidase and a cysteine proteinase were downregulated by OPDA. Chaperonin and LEA facilitate the native folding of proteins,Citation27,28) and peptidyl-propyl cis-trans isomerase is involved in protein folding through the cis-trans isomerization of proline.Citation29) These proteins are involved in spatial protein structural changes, which can trigger signaling that activates and/or deactivates a function, whereas proteolytic enzymes are involved in intracellular protein catabolism. Similar to other proteins related to protein synthesis and structural change, OPDA repressed the expression of proteins involved in amino acid metabolism, including glutamate decarboxylase, glutamate-1-semialdehyde 2,1-aminomutase, and asparagine synthase. These results suggest that OPDA suppresses protein synthesis by downregulating the expression of proteins related to translation and amino acid synthesis. It is therefore possible that OPDA suppresses protein synthesis to avoid unnecessary energy consumption in response to adverse environmental conditions. The expression of proteins involved in protein structural changes (a chaperone, proteinase, and peptidyl prolyl cis-trans isomerase) was also inhibited. The suppression of protein expression might be correlated with the repression of amino acid synthesis.
Semi-quantitative PCR analysis of genes involved in photosynthesis after OPDA treatment
Fifteen proteins functioning in light-dependent reactions were induced by OPDA in P. patens, and we examined whether the transcript levels of the genes encoding these proteins were also increased. P. patens grown on BCDATG medium for 20 days, predominantly at the gametophore stage, was treated with 10 μM OPDA for 1, 3, 6, 12, and 24 h, and total RNA was prepared. We analyzed the gene expression of psaA (PhpapaCp039), psaB (PhpapaCp040), psbC (PhpapaCp043), psbD (PhpapaCp044), and psbE (PhpapaCp020), which are encoded by the chloroplast genome and for which the corresponding proteins were induced by OPDA. The results showed that the expression of these five chloroplast genes was transiently increased by OPDA treatment (Fig. ), suggesting an OPDA-mediated regulatory system for chloroplast gene expression in P. patens. However, the increased expression of proteins corresponding to the five genes was observed 24 h after OPDA treatment. The time gap of expression level between the genes and the proteins might be caused by length of translation process.
Fig. 3. Semi-quantitative RT-PCR analysis of psaA, psaB, psbC, psbD, psbE, and PpAOC1 in P. patens treated with OPDA.
Notes: PCR was performed using cDNA that was prepared from the total RNA of P. patens treated with 10 μM OPDA, and specific primer sets for psaA, psaB, psbC, psbD, psbE, and PpAOC1. The gels were visualized via ethidium bromide staining.
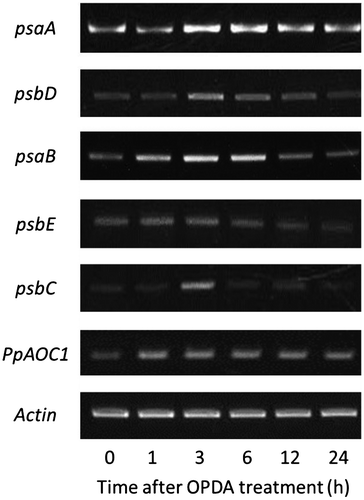
OPDA also induced proteins related to light-dependent reactions, including light-harvesting chlorophyll a/b-binding proteins and photosystem I reaction center, which are encoded by the nuclear genome. In contrast, the transcript level of the genes encoding light-harvesting chlorophyll a/b-binding proteins and photosystem I reaction center was not significantly affected by OPDA treatment (Supplemental Fig. 4); however, OPDA might stimulate the expression of these proteins in a post-translational manner.
Semi-quantitative PCR analysis of PpAOC1 after OPDA treatment
In P. patens, PpAOC1 is considered to function as an AOC enzyme in the octadecanoid pathway.Citation8) Our proteome analysis revealed that the expression of PpAOC1 was induced at 24 h after OPDA treatment. The transcript level of the PpAOC1 gene (Pp1s378_11V6.1) was then analyzed by semi-quantitative PCR and found to be induced in P. patens by OPDA treatment (Fig. ), and this positive feedback regulation by OPDA was also confirmed transcriptionally. This regulatory system for the octadecanoid pathway at the transcritional level was most likely conserved during land plant evolution. In the other hand, JA did not induce PpAOC1 expression (data not shown).
Comparison of proteome data between P. patens and Arabidopsis
It is likely that the protein expression profiles influenced by OPDA are completely different between P. patens and Arabidopsis. In Arabidopsis, half of the OPDA-induced proteins were found to be involved in the response to biotic and abiotic stresses; moreover, OPDA stimulated the expression of proteins associated with protein synthesis and amino acid metabolism.Citation30) Conversely, the proteins induced by OPDA in Arabidopsis were not upregulated by OPDA in P. patens. Induction of elongation factor EF-Tu has been shown to increase tolerance to stress in maize and wheat.Citation30) OPDA might induce physiological responses to stress more actively in flowering plants. Interestingly, some of the OPDA-inducible proteins in P. patens are encoded by the chloroplasts and are correlated with light-dependent reactions. Because OPDA biosynthesis occurs in chloroplasts, it is reasonable to predict that OPDA functions as a regulatory signal for chloroplast gene expression in P. patens. To our knowledge, the effects of OPDA on chloroplast genes have not been examined to date in flowering plants. Thus, a comparison of the effects of OPDA on chloroplast gene expression in flowering plants and P. patens might offer new insight into the mechanism of gene expression in chloroplasts. Nonetheless, the different OPDA treatment conditions between our study (10 μM of (+)-cis-OPDA for 24 h) and the Arabidopsis study (100 μM of racemic OPDA for 6 h)Citation30) might have resulted in an entirely different protein expression pattern. Alternatively, given that mosses are considered to be one of ancestors of vascular plants, OPDA functions might partially change during plant evolution. Regardless, OPDA caused similar alterations in the protein expression patterns of chlorophyll a/b-binding proteins (upregulation) and RubisCo (downregulation), both of which are involved in photosynthesis, in Arabidopsis and P. patens.
Conclusions
This is the first report describing a proteomic analysis of P. patens treated with OPDA. The present proteomic investigation revealed that OPDA mainly suppressed the expression of a broad range of proteins. The OPDA-mediated suppression of proteins involved in carbon fixation, metabolism, and protein biosynthesis has been suggested to inhibit the growth of P. patens. It is interesting that the expression of proteins and genes involved in light-dependent reactions was stimulated by OPDA in P. patens, and OPDA might have specific functions in chloroplasts for stress adaptation in this moss. The present data enhance our understanding of signaling networks in P. patens, an organism that is responsive to OPDA, which is an important oxylipin in plants. Considering the limitations of proteomic studies, complementary transcriptome analysis and proteomic analysis in different developmental stages of P. patens are needed to obtain more information regarding OPDA signaling.
Supplemental material
The supplemental material for this paper is available at http://dx.doi.org/10.1080/09168451.2014.912112.
Supplemental Table 1 and Figs. 1-4
Download PDF (325.4 KB)Supplemental text
Download MS Word (13.8 KB)Acknowledgements
We are grateful to Dr. Shinano at the National Agriculture and Food Research Organization, National Agricultural Research Center for the Hokkaido Region, and Dr. Takabayashi at Hokkaido University for their valuable advice.
Notes
Abbreviations: AOC, allene oxide cyclase; AOS, allene oxide synthase; JA, jasmonic acid; LC-MS/MS, liquid chromatography tandem mass spectroscopy; LOX, lipoxygenase; OPDA, 12-oxo-phytodienoic acid; RES, reactive electrophile species; ROS, reactive oxygen species; UPLC-MS/MS, ultra-performance liquid chromatography tandem mass spectroscopy.
References
- Böttcher C, Pollmann S. Plant oxylipins: Plant responses to 12-oxo-phytodienoic acid are governed by its specific structural and functional properties. FEBS J. 2009;276:4693–4704.10.1111/j.1742-4658.2009.07195.x
- Taki N, Sasaki-Sekimoto Y, Obayashi T, Kikuta A, Kobayashi K, Ohta H. 12-oxo-phytodienoic acid triggers expression of a distinct set of genes and plays a role in wound-induced gene expression in Arabidopsis. Plant Physiol. 2005;139:1268–1283.10.1104/pp.105.067058
- Goetz S, Hellwege A, Stenzel I, Kutter C, Hauptmann V, Forner S, McCaig B, Hause G, Miersch O, Wasternack C, Hause B. Role of cis-12-oxo-phytodienoic acid in tomato embryo development. Plant Physiol. 2012;158:1715–1727.10.1104/pp.111.192658
- Wasternack C, Kombrink E. Jasmonates: structural requirements for lipid-derived signals active in plant stress responses and development. ACS Chem. Biol. 2010;5:63–77.10.1021/cb900269u
- Rensing SA, Lang D, Zimmer AD, Terry A, Salamov A, Shapiro H, Nishiyama T, Perroud PF, Lindquist EA, Kamisugi Y, Tanahashi T, Sakakibara K, Fujita T, Oishi K, Shin-I T, Kuroki Y, Toyoda A, Suzuki Y, Hashimoto S, Yamaguchi K, Sugano S, Kohara Y, Fujiyama A, Anterola A, Aoki S, Ashton N, Barbazuk WB, Barker E, Bennetzen JL, Blankenship R, Cho SH, Dutcher SK, Estelle M, Fawcett JA, Gundlach H, Hanada K, Heyl A, Hicks KA, Hughes J, Lohr M, Mayer K, Melkozernov A, Murata T, Nelson DR, Pils B, Prigge M, Reiss B, Renner T, Rombauts S, Rushton PJ, Sanderfoot A, Schween G, Shiu SH, Stueber K, Theodoulou FL, Tu H, Van de Peer Y, Verrier PJ, Waters E, Wood A, Yang L, Cove D, Cuming AC, Hasebe M, Lucas S, Mishler BD, Reski R, Grigoriev IV, Quatrano RS, Boore JL. The Physcomitrella genome reveals evolutionary insights into the conquest of land by plants. Science. 2008;319:64–69.10.1126/science.1150646
- Anterola A, Göbel C, Hornung E, Sellhorn G, Feussner I, Grimes H. Physcomitrella patens has lipoxygenases for both eicosanoid and octadecanoid pathways. Phytochemistry. 2009;70:40–52.10.1016/j.phytochem.2008.11.012
- Bandara PKGSS, Takahashi K, Sato M, Matsuura H, Nebata K. Cloning and functional analysis of an allene oxide synthase in Physcomitrella patens. Biosci., Biotechnol., Biochem. 2009;73:2356–2359.
- Stumpe M, Göbel C, Faltin B, Beike AK, Hause B, Himmelsbach K, Bode J, Kramell R, Wasternack C, Frank W, Reski R, Feussner I. The moss Physcomitrella patens contains cyclopentenones but no jasmonates: mutations in allene oxide cyclase lead to reduced fertility and altered sporophyte morphology. New Phytol. 2010;188:740–749.10.1111/j.1469-8137.2010.03406.x
- Hashimoto T, Takahashi K, Sato M, Bandara PKGSS, Nabeta K. Cloning and characterization of an allene oxide cyclase, PpAOC3, in Physcomitrella patens. Plant Growth Regul. 2011;65:239–245.10.1007/s10725-011-9592-z
- Oliver JP, Castro A, Gaggero C, Cascón T, Schmelz EA, Castresana C, Ponce de León I. Pythium infection activates conserved plant defense responses in mosses. Planta. 2009;230:569–579.10.1007/s00425-009-0969-4
- Scholz J, Brodhun F, Hornung E, Herrfurth C, Stumpe M, Beike AK, Faltin B, Frank W, Reski R, Feussner I. Biosynthesis of allene oxides in Physcomitrella patens. BMC Plant Biol. 2012;12:228. doi:10.1186/1471-2229-12-22810.1186/1471-2229-12-228
- Ponce De León I, Schmelz EA, Gaggero C, Castro A, Álvarez A, Montesano M. Physcomitrella patens activates reinforcement of the cell wall, programmed cell death and accumulation of evolutionary conserved defense signals, such as salicylic acid and 12-oxo-phytodienoic acid, but not jasmonic acid, upon Botrytis cinerea infection. Mol. Plant Pathol. 2012;13:960–974.10.1111/mpp.2012.13.issue-8
- Ashton NW, Cove D. The isolation and preliminary characterization of auxotrophicmutants of the moss, Physcomitrella patens. MGG Mol. Gen. Genet. 1977;154:87–95.10.1007/BF00265581
- Nishiyama T, Hiwatashi Y, Sakakibara I, Kato M, Hasebe M. Tagged mutagenesis and gene-trap in the moss, Physcomitrella patens by shuttle mutagenesis. DNA Res. 2000;7:9–17.10.1093/dnares/7.1.9
- Kajiwara A, Abe T, Hashimoto T, Matsuura H, Takahashi K. Efficient synthesis of (+)-cis-12-oxo-phytodienoic acid by in vitro enzymatic reaction. Biosci., Biotechnol., Biochem. 2012;76:2325–2328.10.1271/bbb.120506
- Sato C, Aikawa K, Sugiyama S, Nabeta K, Masuta C, Matsuura H. Distal transport of exogenously applied jasmonoyl-isoleucine with wounding stress. Plant Cell Physiol. 2011;52:509–517.10.1093/pcp/pcr011
- Matsuura H, Ohmori F, Kobayashi M, Sakurai A, Yoshihara T. Qualitative and quantitative analysis of endogenous jasmonoids in potato plant (Solanum tuberosum L.). Biosci., Biotechnol., Biochem. 2000;64:2380–2387.10.1271/bbb.64.2380
- Olsen JV, de Godoy LM, Li G, Macek B, Mortensen P, Pesch R, Makarov A, Lange O, Horning S, Mann M. Parts per million mass accuracy on an orbitrap mass spectrometer via lock mass injection into a C-trap. Mol. Cell. Proteomics. 2005;4:2010–2021.10.1074/mcp.T500030-MCP200
- Zhang L, Tian LH, Zhao JF, Song Y, Zhang CJ, Guo Y. Identification of an apoplastic protein involved in the initial phase of salt stress response in rice root by two-dimensional electrophoresis. Plant Physiol. 2009;149:916–928.
- Brosch M, Yu L, Hubbard T, Choudhary J. Accurate and sensitive peptide identification with Mascot Percolator. J. Proteome Res. 2009;8:3176–3181.10.1021/pr800982s
- Aoki S, Kato S, Ichikawa K, Shimizu M. Circadian expression of the PpLhcb2 gene encoding a major light-harvesting chlorophyll a/b-binding protein in the moss Physcomitrella patens. Plant Cell Physiol. 2004;45:68–76.10.1093/pcp/pch006
- Vass I, Cser K. Janus-faced charge recombinations in photosystem II photoinhibition. Trends Plant Sci. 2009;14:200–205.
- Farmer EE, Mueller MJ. ROS-mediated lipid peroxidation and RES-activated signaling. Ann. Rev. Plant Biol. 2013;64:429–450.10.1146/annurev-arplant-050312-120132
- Stenzel I, Hause B, Miersch O, Kurz T, Maucher H, Weichert H, Ziegler J, Feussner I, Wasternack C. Jasmonate biosynthesis and the allene oxide cyclase family of Arabidopsis thaliana. Plant Mol. Biol. 2003;51:895–911.10.1023/A:1023049319723
- Hossain Z, Amyot L, McGarvey B, Gruber M, Jung J, Hannoufa A. The translation elongation factor eEF-1Bβ1 is involved in cell wall biosynthesis and plant development in Arabidopsis thaliana. Plos One. 2012;7:e30425. doi:10.1371/journal.pone.003042510.1371/journal.pone.0030425
- Carrington JC, Ambros V. Role of microRNAs in plant and animal development. Science. 2003;301:336–338.10.1126/science.1085242
- Fenton WA, Horwich AL. Chaperonin-mediated protein folding: fate of substrate polypeptide. Q. Rev. Biophys. 2003;36:229–256.10.1017/S0033583503003883
- Goyal K, Walton LJ, Tunnacliffe A. LEA proteins prevent protein aggregation due to water stress. Biochem. J. 2005;388:151–157.
- Balbach J, Schmid FX. Proline isomerizarion and its catalysis in protein folding. In: Pain RH, editor. Mechanisms of protein folding. 2nd ed. Oxford University Press: Oxford; 2000. p. 212–249.
- Dueckershoff K, Mueller S, Mueller MJ, Reinders J. Impact of cyclopentenone-oxylipins on the proteome of Arabidopsis thaliana. Biochim. Biophys. Acta – Proteins Proteomics. 2008;1784:1975–1985.10.1016/j.bbapap.2008.09.003