Abstract
Yamogenin is a diastereomer of diosgenin, which we have identified as the compound responsible for the anti-hyperlipidemic effect of fenugreek. Here, we examined the effects of yamogenin on the accumulation of triacylglyceride (TG) in hepatocytes, because yamogenin is also contained in fenugreek. It was demonstrated that yamogenin also inhibited TG accumulation in HepG2 hepatocytes and suppressed the mRNA expression of fatty acid synthesis-related genes such as fatty acid synthase and sterol response element-binding protein-1c. Indeed, yamogenin also antagonized the activation of the liver X receptor (LXR) in luciferase ligand assay similar to diosgenin. However, yamogenin could not exert such effects in the presence of T0901713, a potent agonist of LXR. These findings indicate that the effects of yamogenin on TG accumulation would be weaker than those of diosgenin, suggesting that the structural difference between yamogenin and diosgenin would be important for the inhibition of LXR activation.
Graphical Abstract
Yamogenin inhibited lipid accumulation in HepG2 hepatocytes and suppressed the mRNA expression of fatty-acid-synthesis-related genes through inhibition of ligand-dependent LXR activation.
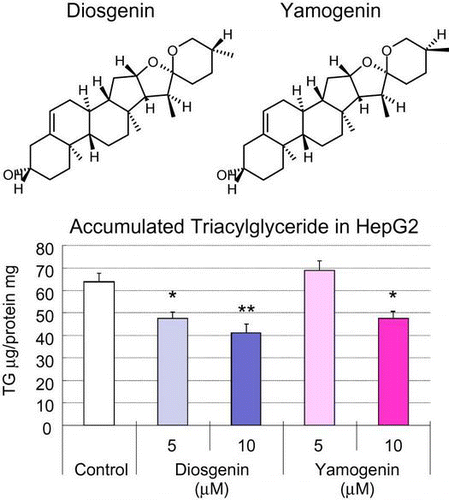
Excessive hepatic lipid accumulation is a serious metabolic disease contributing to the development of hepatic steatosis, diabetic mellitus, and obesity. Hepatic lipid is accumulated owing to the abnormalities of hepatic lipid metabolism such as the inhibition of fatty acid oxidation and stimulation of fatty acid synthesis.Citation1,2) Therefore, it is indispensable for preventing the development of steatosis to improve hepatic lipid metabolism. Hepatic lipid metabolism is regulated by various factors including nuclear receptors. Peroxisome proliferator-activated receptor-α, a nuclear receptor, activated by fatty acids and food-derived lipophilic compounds, stimulates fatty acid oxidation through the increase in mRNA expression levels of fatty acid oxidation-related genes such as Acyl-CoA oxidase and carnitine-palmitoyl transferase-1a in the liver and skeletal muscle.Citation3,4) On the other hand, the liver X receptor (LXR) promotes hepatic fatty acid synthesis through the induction of fatty acid synthase (FAS) and sterol response element-binding protein-1c (SREBP1c) in the liver.Citation5,6) The activities of these nuclear receptors change under diabetic and/or obese conditions. Hepatic LXR activity increases with the development of diabetes and obesity, resulting in hepatic fatty acid synthesis, which is being excessively stimulated.Citation7) Thus, the inhibition of the excessive activation of LXR in the liver is one of the adequate targets for improving hepatic lipid metabolism.
It has been demonstrated that fenugreek and its main aglycone, diosgenin, improve various pathological conditions such as hyperglycemia and hypercholesterolemia.Citation8–11) Recently, we have found in a study that fenugreek feeding improves hyperlipidemia through the inhibition of the hyperactivation of hepatic fatty acid synthesis and that the responsible compound is diosgenin.Citation12) In that study, we have identified the responsible compound by screening using T0901317 (a potent agonist of LXR)-stimulated lipid accumulation in HepG2 hepatocytes. Only diosgenin has shown the inhibitory effect on lipid accumulation. However, here we report that yamogenin, another aglycone in fenugreek, could suppress lipid accumulation in HepG2 hepatocytes in the absence of the potent agonist of LXR. Yamogenin suppressed the mRNA expression of fatty acid synthesis-related genes such as SREBP1c and FAS in HepG2 hepatocytes, although this inhibitory effect of yamogenin disappeared in the presence of a potent agonist, T0901713. Thus, our findings suggest that the position of the 25-methyl group is important for the inhibition of LXR activation, that is, the structural difference between yamogenin and diosgenin.
Materials and methods
Chemicals
Yamogenin, which was purified using fenugreek extract, was a kind gift from House Foods Group Inc. Diosgenin was from Wako (Osaka, Japan). Their chemical structures are shown in Fig. . All other chemicals were from Sigma (MO, USA) or Nacalai Tesque (Kyoto, Japan) and were guaranteed to be of reagent or tissue-culture grade.
Cell culture
Mouse hepatocytes were isolated and cultured as previously described.Citation13) Briefly, 5–6-week-old C57/BL/6J male mice (Nihon SLC, Shizuoka, Japan) were anesthetized in the presence of CO2. The livers were obtained and then perfused with 40 ml of liver perfusion medium (Invitrogen, Corp., CA, USA) followed by 30 ml of liver digestion medium (Invitrogen, Corp.), both at a flow rate of 5 ml/min. Hepatocytes were dispersed in hepatocyte wash medium (Invitrogen, Corp.) supplemented with 1% P/S by dissection and gentle shaking. After filtration through a 150-μm nylon mesh filter, hepatocytes were isolated by repeated centrifugation (3–5 times) at 50 g for 3 min. The isolated hepatocytes were resuspended in William’s E medium (Invitrogen, Corp.) supplemented with 10% FBS, 0.1 μM insulin, 1 μM dexamethasone, and 1% P/S, and cultured in Type-1-collagen-coated 12-well plates (Iwaki, Chiba, Japan) at a density of 3 × 105 cells/well. After a 2-h incubation at 37 °C in 5% CO2 atmosphere, the hepatocytes were used for viability assay (cell viability was usually more than 90%) and TG accumulation assay.
HepG2, a human hepatoma cell line, was purchased from ATCC. HepG2 hepatocytes were cultured in 10% FBS/DMEM at 37 °C in 5% CO2 atmosphere before their use for assay. TG accumulated in HepG2 hepatocytes was measured as previously described.Citation13) Briefly, HepG2 hepatocytes were plated on 12-well plates or 60-mm dishes and incubated for 24 h. Then, the cells were treated with yamogenin or diosgenin at various concentrations and/or with T0901317 (1 μM) for 24 or 48 h in serum-free DMEM. The treated cells were washed with PBS and placed immediately in a lysis buffer containing 50 mM PIPES (pH 6.5) and 1% Triton X-100. The lysate was centrifuged at 10,000 rpm for 5 min, and the supernatant was collected. The TG content was measured by a TG E-test (Wako).
Quantitative RT-PCR analysis
RNA samples from differentiated human adipocytes were prepared using cells cultured on six-well tissue culture plates 12 days after differentiation induction. Total RNA samples were prepared from cells cultured on six-well tissue culture plates and from mouse fat tissues using Sepasol Super-I (Nacalai Tesque) and a SV total RNA isolation system (Promega, WI, USA), respectively, in accordance with each manufacturer’s protocol as previously described.Citation13) To quantify mRNA expression, PCR analysis was performed using a fluorescence temperature cycler (LightCycler System: Roche Diagnostics, Mannheim, Germany), as described previously.Citation14,15) Primer sets were designed using a PCR primer selection program available at the website of the Virtual Genomic Center from the GenBank database as previously described.Citation12,13) To compare mRNA expression level among samples, the copy number of each transcript was divided by that of 36B4, showing a constant expression level. All data on mRNA expression level are presented as ratio with respect to the mRNA expression level in a control in each experiment.
Luciferase assay
We used a GAL4-chimera protein system and a dual luciferase assay kit (Promega) and performed luciferase assay as previously described.Citation12) Briefly, we transfected p4xUASg-tk-luc (a reporter plasmid), pCMX-GAL4/human LXRα (an expression vector for the chimera protein for a GAL4-DNA-binding domain and an LXRα-ligand-binding domain), and pRL-CMV (an internal control) into HepG2 cells cultured on 96-well plates. The transfection was performed using LipofectAMINE (Invitrogen Corp.) in accordance with the manufacturer’s protocol. Twenty-four hours after transfection, the cells were cultured in a medium treated with yamogenin for an additional 24 h. The expression vector for the LXR-GAL4 chimera protein was kindly provided by Dr Makoto Makishima of Nippon University.
Statistical analysis
The data are presented as means ± S.E.M. and statistically analyzed by one-way ANOVA when their variances were heterogeneous and the unpaired t-test. Differences were considered significant at p < 0.05.
Results
Yamogenin inhibited TG accumulation in HepG2 and mouse primary hepatocytes
We measured TG accumulation in hepatocytes to examine the effects of yamogenin on hepatic lipid metabolism. Yamogenin inhibited TG accumulation in both mouse primary hepatocytes (Fig. (A)) and HepG2 hepatocytes (Fig. (B)), although the effects of yamogenin were smaller than those of diosgenin in HepG2 hepatocytes. In addition, the inhibitory effect of yamogenin was dose-dependent (Fig. (C)). Under our experimental conditions, yamogenin showed no cytotoxic effect on both mouse primary hepatocytes (Fig. (D)) and HepG2 hepatocytes (data not shown).
Fig. 2. TG accumulation was suppressed by yamogenin in mouse primary and HepG2 hepatocytes.
Notes: The effects of yamogenin on TG accumulation in mouse primary (A) and HepG2 (B and C) hepatocytes were examined. Cells were treated with diosgenin or yamogenin at the indicated concentrations for 24 h. Cell viability was examined using the yamogenin-treated HepG2 hepatocytes (D). The values are means ± S.E.M. of four samples in the measurement of TG accumulation or cell viability. *p < 0.05 and **p < 0.01 compared with each vehicle control and ##p < 0.01 compared between the indicated groups.
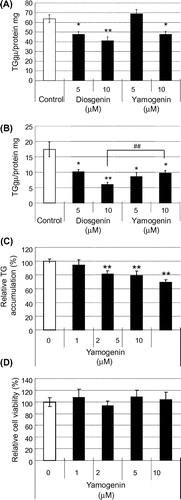
Yamogenin suppressed mRNA expression of fatty acid synthesis-related genes in HepG2 hepatocytes
We examined the mRNA expression levels of fatty acid synthesis-related genes in HepG2 hepatocytes to elucidate the details of the inhibitory effect of yamogenin on hepatic TG accumulation. As shown in Fig. , yamogenin suppressed the mRNA expression of FAS, SCD-1, and ACC. For all these genes, the inhibitory effect of yamogenin was smaller than that of diosgenin as observed in TG accumulation. In addition, the inhibitory effect of yamogenin was dose-dependent, as shown in Fig. .
Fig. 3. Yamogenin decreased mRNA expression levels of fatty acid synthesis-related genes in HepG2 hepatocytes.
Notes: The mRNA expression levels of FA synthesis-related genes, FAS (A), SCD-1 (B), and ACC (C), were measured using HepG2 hepatocytes treated with diosgenin or yamogenin at the indicated concentrations for 24 h. The value of a vehicle control was set at 100% and relative value is presented as fold induction with respect to that of each vehicle control. The values are means ± S.E.M. of four samples in the measurement of TG accumulation. *p < 0.05 and **p < 0.01 compared with each vehicle control and ##p < 0.01 compared between the indicated groups.
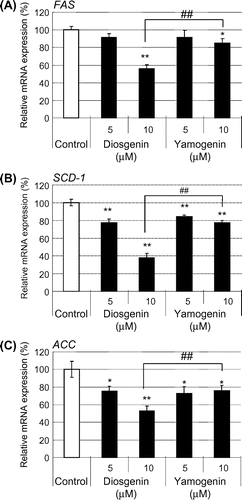
Fig. 4. The effects of yamogenin on the suppression of the mRNA expression levels were dose-dependent.
Notes: The mRNA expression levels of FA synthesis-related genes, FAS (A), SCD-1 (B), and ACC (C), were measured using HepG2 hepatocytes treated with different concentrations of yamogenin for 24 h. The value of a vehicle control was set at 100% and relative value is presented as fold induction with respect to that of each vehicle control. The values are means ± S.E.M. of 3–4 samples in the measurement of TG accumulation. *p < 0.05 and **p < 0.01 compared with each vehicle control.
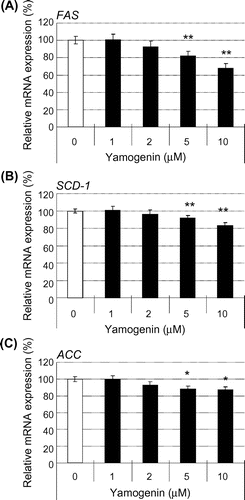
Yamogenin inhibited the activation of LXR
Next, we examined the effects of yamogenin on LXR activation because the expression of fatty acid synthesis-related genes is regulated by the activity of LXR. As shown in Fig. , yamogenin suppressed the agonist-dependent activation of LXR in luciferase ligand assay. The inhibitory effect of yamogenin was smaller than that of diosgenin as observed in TG accumulation and mRNA expression.
Fig. 5. Yamogenin inhibited ligand-dependent activation of LXR in luciferase assay.
Notes: The inhibitory effect of yamogenin on ligand-induced LXR activation was examined using HepG2 hepatocytes. Reporter plasmids were transfected in HepG2 hepatocytes, and then 24 h after the transfection, the cells were treated with diosgenin or yamogenin at the indicated concentrations for 24 h in the presence of an LXR agonist (500 nM T0901317). The value of a vehicle control was set at 100% and relative value is presented as fold induction with respect to that of each vehicle control. The values are means ± S.E.M. of 3–4 samples in the measurement of TG accumulation. *p < 0.05 and **p < 0.01 compared with T0901317 treatment and #p < 0.05 and ##p < 0.01 compared between indicated groups.
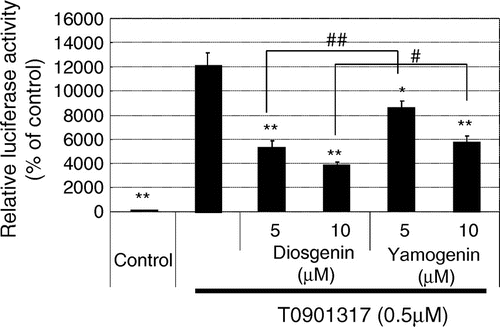
To examine the details of the inhibitory effect of yamogenin in comparison with those of diosgenin, we measured TG accumulation and examined the mRNA expression of fatty acid synthesis-related genes in the presence of T0901317, a potent agonist of LXR. As shown in Fig. (A), yamogenin did not suppress the T0901317-dependent TG accumulation in HepG2 hepatocytes, although diosgenin significantly suppressed the accumulation. The mRNA expression levels of fatty acid synthesis-related genes, such as FAS, SCD-1, and ACC, similarly showed no changes following the yamogenin treatment in the presence of T0901317 (Fig. (B)–(D)). These findings indicate that the inhibitory effect of yamogenin is smaller than that of diosgenin.
Fig. 6. No effect of yamogenin was observed in the presence of an LXR agonist.
Notes: HepG2 hepatocytes were treated with diosgenin or yamogenin at the indicated concentrations for 24 h in the presence of an LXR agonist (500 nM T0901317). TG accumulation (A) and mRNA expression level (B–D) were measured. The values are means ± S.E.M. of 3–4 samples in the measurement of TG accumulation. In the measurement of mRNA expression level, the value of a vehicle control was set at 100% and relative value is presented as fold induction with respect to that of each vehicle control. The values are means ± S.E.M. of 3–4 samples. **p < 0.01 compared with each T0901317 treatment and ##p < 0.01 compared between indicated groups.
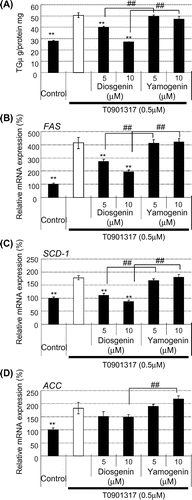
Yamogenin suppressed mRNA expression of SREBP-1c in HepG2 hepatocytes
Finally, we examined the effects of yamogenin on the expression level of SREBP-1c, a master gene of fatty acid synthesis in the liver. Yamogenin suppressed the mRNA expression of SREBP-1c in HepG2 hepatocytes and the inhibitory effect was dose-dependent (Fig. (A) and (B)). In addition, as expected, the inhibitory effect of yamogenin disappeared in the presence of T0901317, as shown in Fig. (C).
Fig. 7. Yamogenin inhibited SREBP-1c induction in HepG2 hepatocytes.
Notes: SREBP-1c mRNA expression level was quantified using HepG2 hepatocytes in the absence (A and B) or presence (C) of T0901317 (1 μM). The value of a vehicle control was set at 100% and relative value is presented as fold induction with respect to that of each vehicle control. The values are means ± S.E.M. of 3–4 samples. *p < 0.05 and **p < 0.01 compared with each vehicle control (A and B) or T0901317 treatment (C) and ##p < 0.01 compared between the indicated groups.
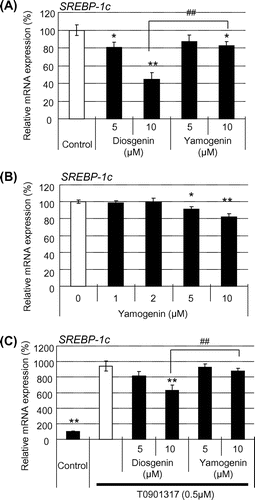
Discussion
It has been reported that fenugreek feeding suppresses hepatic lipid accumulation and fatty acid synthesis through the inhibition of the mRNA expression of fatty acid synthesis-related genes such as SREBP-1c.Citation12) In our previous study, diosgenin has been identified as the compound responsible for the anti-hyperlipidemic effect, as determined by measuring TG accumulation in HepG2 hepatocytes, although yamogenin, a diastereomer of diosgenin, is contained in fenugreek. In that previous study, T0901317, a potent agonist of LXR, has been used for sufficient accumulation of TG in HepG2 hepatocytes. However, as shown in this study, yamogenin could not suppress the T0901317-dependent TG accumulation, whereas the TG accumulation in the absence of T0901317 was suppressed in HepG2 hepatocytes. This is the reason why yamogenin has not been identified in the previous study. Here, we examined the effects of yamogenin on TG accumulation in hepatocytes and found that yamogenin also inhibited TG accumulation and suppressed the mRNA expression of fatty acid synthesis-related genes, although these effects were smaller than those of diosgenin. However, fenugreek contains a non trivial amount of yamogenin (approximately 50% of diosgenin).Citation16) Thus, there is a possibility that yamogenin contributes to the anti-fatty liver and anti-hyperlipidemic effects of fenugreek as well as diosgenin. This possibility should be examined in future experiments using an obesity mouse model fed a yamogenin-containing diet.
Yamogenin is a diastereomer of diosgenin, as mentioned above. Yamogenin and diosgenin are (25S)- and (25R)-spirost-5-en-3β-ol, respectively. Thus, their difference is only in the position of the methyl group at the 25th asymmetric carbon (Fig. ). Although X-ray crystallography is necessary for confirmation, our findings suggest that the methyl group is very important for binding to the LXR ligand-binding domain. Such information is likely to be significant for screening other compounds and designing chemicals that inhibit the ligand-dependent activation of LXR. Indeed, diastereomers/enantiomers show different activities in the activation of nuclear receptors such as the estrogen receptor, peroxisome proliferator-activated receptor, and pregnane-X-receptor.Citation17–19) In addition, a natural flavonone hesperetin (an aglycone of hesperidin), which is the major flavonoid present in sweet oranges, exists as S- and R-enantiomers. Interestingly, the enantiomers show stereoselective differences in their metabolism, transport, and transcriptional factor activation.Citation20) Thus, there is a possibility that yamogenin would show some differences in its in vivo metabolism and transport in comparison with diosgenin. Further investigations that elucidate the stereoselective differences among food-derived compounds such as yamogenin and diosgenin are required to clarify the bioactivities of their racemic mixtures, which may lead to the development of compounds with beneficial bioactivities.
In conclusion, yamogenin suppressed the TG accumulation and mRNA expression of fatty acid synthesis-related genes such as FAS, SCD-1, ACC, and SREBP-1c in hepatocytes. In particular, the inhibitory effect of yamogenin on the mRNA expression of SREBP-1c is important because SREBP-1c is a key regulator in fatty acid synthesis in the liver.Citation21) However, the inhibitory effect of yamogenin was smaller than that of diosgenin and disappeared in the presence of a potent LXR agonist. The yamogenin content in fenugreek is as much as about 50% of that of diosgenin. Thus, our findings suggest that yamogenin contributes to the beneficial effects of fenugreek in terms of the prevention of hepatic TG accumulation similar to diosgenin and that the stereoselective difference between yamogenin and diosgenin is important for the inhibition of LXR activation.
Acknowledgements
The authors thank S. Shinotoh for secretarial support and Y. Tada for technical support.
Funding
This work was supported by Grants-in-Aid for Scientific Research from the Ministry of Education, Culture, Sport, Science and Technology of Japan [grant number 22228001] and [grant number 22380075].
References
- Kahn BB, Flier JS. Obesity and insulin resistance. J. Clin. Invest. 2000;106:473–481.10.1172/JCI10842
- Lewis GF, Carpentier A, Adeli K, Adria K. Disordered fat storage and mobilization in the pathogenesis of insulin resistance and type 2 diabetes. Endocr. Rev. 2002;23:201–229.10.1210/edrv.23.2.0461
- Roepstorff C, Halberg N, Hillig T, Saha AK, Ruderman NB, Wojtaszewski JF, Richter EA, Kiens B. Malonyl-CoA and carnitine in regulation of fat oxidation in human skeletal muscle during exercise. Am. J. Physiol. Endocrinol. Metab. 2005;288:133–142.
- Tugwood JD, Issemann I, Anderson RG, Bundell KR, McPheat WL, Green S. The mouse peroxisome proliferator activated receptor recognizes a response element in the 5′ flanking sequence of the rat acyl CoA oxidase gene. EMBO J. 1992;11:433–439.
- Chen G, Liang G, Ou J, Goldstein JL, Brown MS. Central role for liver X receptor in insulin-mediated activation of Srebp-1c transcription and stimulation of fatty acid synthesis in liver. Proc. Natl. Acad. Sci. USA, 2004;101:11245–11250.10.1073/pnas.0404297101
- Edwards PA, Kast HR, Anisfeld AM. BAREing it all: the adoption of LXR and FXR and their roles in lipid homeostasis. J. Lipid Res. 2002;43:2–12.
- Brown MS, Goldstein JL. A proteolytic pathway that controls the cholesterol content of membranes, cells, and blood. Proc. Natl. Acad. Sci. USA, 1999;96:11041–11048.10.1073/pnas.96.20.11041
- Morton JF. Mucilaginous plants and their uses in medicine. J. Ethnopharmacol. 1990;29:215–266.
- Basch E, Ulbricht C, Kuo G, Szapary P, Smith M. Therapeutic applications of fenugreek. Altern. Med. Rev. 2003;8:20–27.
- Trinh HT, Han SJ, Kim SW, Lee YC, Kim DH. Bifidus fermentation increases hypolipidemic and hypoglycemic effects of red ginseng. J. Microbiol. Biotechnol. 2007;17:1127–1133.
- Li H, Wang QJ, Zhu DN, Yang Y. Reinioside C, a triterpene saponin of Polygala aureocauda Dunn, exerts hypolipidemic effect on hyperlipidemic mice. Phytother. Res. 2008;22:159–164.10.1002/(ISSN)1099-1573
- Uemura T, Goto T, Kang MS, Mizoguchi N, Hirai S, Lee JY, Nakano Y, Shono J, Hoshino S, Tsuge N, Narukami T, Makishima M, Takahashi N, Kawada T. Diosgenin, the main aglycon of fenugreek, inhibits LXRα activity in HepG2 cells and decreases plasma and hepatic triglycerides in obese diabetic mice. J. Nutr. 2011;141:17–23.10.3945/jn.110.125591
- Kim IL, Hirai S, Takahashi H, Goto T, Ohyane C, Tsugane T, Konishi C, Fujii T, Inai S, Iijima Y, Aoki K, Shibata D, Takahashi N, Kawada T. 9-oxo-10(E),12(E)-Octadecadienoic acid derived from tomato is a potent PPAR α aganist to decrease triglyceride accumulation in mouse primary hepatocytes. Mol. Nutr. Food Res. 2011;55:585–593.10.1002/mnfr.v55.4
- Takahashi N, Kawada T, Yamamoto T, Goto T, Taimatsu A, Aoki N, Kawasaki H, Taira K, Yokoyama KK, Kamei Y, Fushiki T. Overexpression and ribozyme-mediated targeting of transcriptional coactivators CREB-binding protein and p300 revealed their indispensable roles in adipocyte differentiation through the regulation of peroxisome proliferator-activated receptor gamma. J. Biol. Chem. 2002;277:16906–16912.10.1074/jbc.M200585200
- Takahashi N, Kawada T, Goto T, Yamamoto T, Taimatsu A, Kimura K, Saitoh M, Hosokawa T, Miyashita K, Fushiki T. Dual action of isoprenols from herbal medicines on both PPARgamma and PPARalpha in 3T3-L1 adipocytes and HepG2 hepatocytes. FEBS Lett. 2002;514:315–322.10.1016/S0014-5793(02)02390-6
- Taylor WG, Elder JL, Chang PR, Rixhards KW. Microdetermination of diosgenin from fenugreek (Trigonella foenum-graecum) seeds. J. Agric. Food Chem. 2000;48:5206–5210.10.1021/jf000467t
- Carroll VM, Jeyakumar M, Carlson KE, Katzenellenbogen JA. Diarylpropionitrile (DPN) enantiomers: synthesis and evaluation of estrogen receptor β-selective ligands. J. Med. Chem. 2012;55:528–537.10.1021/jm201436k
- Pochetti G, Godio C, Mitro N, Caruso D, Galmozzi A, Scurati S, Loiodice F, Fracchiolla G, Tortorella P, Laghezza A, Lavecchia A, Novellino E, Mazza F, Crestani M. Insights into the mechanism of partial agonism: crystal structures of the peroxisome proliferator-activated receptor gamma ligand-binding domain in the complex with two enantiomeric ligands. J. Biol. Chem. 2007;282:17314–17324.10.1074/jbc.M702316200
- Ying M, Stephenson CRJ, Kendall C, Saini SPS, Toma D, Ren S, Cai H, Strom SC, Day BW, Wipf P, Xie W. A pregnane X receptor agonist with unique species-dependent stereoselectivity and its implications in drug development. Mol. Pharmacol. 2005;68:403–413.
- Brand W, Shao J, Hoek-van den Hil EF, van Elk KN, Spenkelink B, de Haan LH, Rein MJ, Dionisi F, Williamson G, van Bladeren PJ, Rietjens IM. Stereoselective conjugation, transport and bioactivity of s- and R-hesperetin enantiomers in vitro. J. Agric. Food Chem. 2010;58:6119–6125.
- Horton JD, Goldstein JL, Brown MS. Activation of cholesterol synthesis in preference to fatty acid synthesis in liver and adipose tissue of transgenic mice overproducing sterol regulatory element-binding protein-2. J. Clin. Invest. 2002;101:2331–2339.