Abstract
Pyrroloquinoline quinone-dependent quinoprotein alcohol dehydrogenases (PQQ-ADH) require ammonia or primary amines as activators in in vitro assays with artificial electron acceptors. We found that PQQ-ADH from Pseudomonas putida KT2440 (PpADH) was activated by various primary amines, di-methylamine, and tri-methylamine. The alcohol oxidation activity of PpADH was strongly enhanced and the affinity for substrates was also improved by pentylamine as an activator.
Quinoprotein alcohol dehydrogenases (ADHs) containing pyrroloquinoline quinone (PQQ) as a prosthetic group mainly catalyze the dehydrogenation of alcohols in the periplasm of Gram-negative bacteria. In addition to the PQQ-dependent methanol dehydrogenase (MDH) of methylotrophs,Citation1) ADHs are categorized into three groups (type I, II, and III ADHs) according to their domain structure and localization.Citation2,Citation3) Type I ADHs are soluble quinoproteins such as ethanol dehydrogenase (QEDH) from Pseudomonas aeruginosa ATCC17933Citation4,Citation5) and ADH I from Pseudomonas putida HK5.Citation6) These type I ADHs have molecular and enzymatic properties that are very similar to those of MDHs, but they have a low affinity for methanol. Type II ADH is a monomeric soluble quinohemoprotein that contains a cytochrome c domain and a PQQ-binding domain that is very similar to that of type I ADH.Citation7,Citation8) Type III ADH is a membrane-bound quinohemoprotein that consists of several subunits and is found in the cytoplasmic membrane of acetic acid bacteria.Citation9)
MDH and type I ADHs have a unique feature; it is necessary to add ammonia, methylamine, or ethylamine as essential activators in standard assays using an artificial electron acceptor such as cationic dyes, phenazine methosulfate (PMS), or phenazine ethosulfate.Citation4,Citation10) These activators are also inhibitory at high concentrations.Citation11)
The requirement for essential activators for MDH activity has been well studied. For the MDH isolated from Pseudomonas sp M27, ammonia can be replaced by methylamine but not di-methylamine, tri-methylamine, and long-chain alkylamines,Citation12) while the MDH from Metylomonas P11 is activated by ammonia but not methylamine.Citation13) Hyphomicrobium X and Methylophilus methylotrophus MDHs are activated by not only ammonia or methylamine but also by glycine esters; however, aliphatic amines and amino acids are not activators.Citation13) In an exceptional case, the enzyme from Methylobacterium organophilum does not require ammonia or methylamine for enzyme activity.Citation14) Goodwin and Anthony have shown an effect of ammonia on the activation of the PQQ reduction step by examining the deuterium isotope effect.Citation15,Citation16) Parvider et al. reported that the MDH from M. methylotrophus has stimulatory and inhibitory activator-binding sites that are located in the active site cavity.Citation17) Furthermore, kinetic studies and molecular modeling of MDH with the alternative activator glycine esters indicated that MDH has two spatially distinct inhibitory sites, suggesting a close relationship between multiple ligand sites and the catalytic binding site.Citation18) In addition, molecular dynamics (MD) studies of the mechanism of ammonia activation suggest that the intermediate complex MDH-PQQ of the >C5(H)NH2 derivative of the PQQ imine may be formed in the oxidation of methanol.Citation19) A great deal of interest has focused on the requirement for an essential activator in methanol oxidation by MDH.
Type I ADHs, on the other hand, generally use ethylamine or methylamine as essential activators instead of ammonia, and other activators have not been widely discussed. In an earlier study, dye-linked ADH from Rhodopseudomonas acidophila, which is considered a type I ADH, has been reported to be activated by primary alkyl amines as well as ammonia.Citation20) However, few reports are available on other type I ADHs, and no adequate studies have been performed on the amine activator. In the present study, we examined various amines as activators of the type I ADH from P. putida KT2440 (PpADH). Kinetic analysis indicated that alcohol-oxidizing activity in PpADH was improved in the presence of pentylamine.
PpADH was heterologously expressed and purified as described previously.Citation21) Assays of the catalytic activity of PpADH were measured at 600 nm and 25 °C with the artificial electron acceptors PMS and 2,6-dichlorophenolindophenol (DCPIP) according to previous studies.Citation4,Citation21) PMS was used as the primary electron acceptor, and the re-oxidation of reduced PMS was coupled to reduction in DCPIP. The assay mixture contained 50 mM CHES (pH = 9.0), 0.1 mM DCPIP, 1 mM PMS, 1 mM CaCl2, 10 mM of various amines, and 10 mM propanol. Enzyme activities are expressed in units mg−1, where one unit corresponds to the reduction of 1 μmol DCPIP per min. The PpADH concentration was estimated from the absorbance at 280 nm (ε = 162.1 mM−1cm−1) as described previously. To obtain the steady-state kinetic parameters of ethylamine and pentylamine as activators, the reduction of 0.1 mM DCPIP was carried out with ethylamine (1–200 mM) and pentylamine (1–200 mM) in the assay. Because the addition of amines to reaction mixtures resulted in a reaction pH higher than 9.0, stock solutions of various concentrations of amines and 100 mM CHES were titrated with NaOH or HCl to pH 9.0. Initial velocity data collected with different individual activator concentrations ([S]) were fit to the equation for substrate inhibition using Origin 8.1 (OriginLab, Inc.)(1)
where KA and Ki are the activation and inhibition constants for amines, respectively. v0 and Vmax are the initial velocity and the theoretical maximum rate for the reduction in DCPIP, respectively. To obtain the steady-state kinetic parameters for ethanol and propanol oxidation, the reduction rate with 0.1 mM DCPIP was plotted against various concentrations of ethanol (0.0625–1 mM) and propanol (0.045–10 mM) using 30 mM ethylamine and 10 mM pentylamine as activators, respectively. The Km and kcat values of the substrate oxidation were estimated by the linear fitting of the Lineweaver-Burk equation to the data using Origin 8.1 (OriginLab, Inc.)
The alcohol oxidation activity of PpADH was remarkably increased in the presence of ethylamine, which is similar to the results of other PQQ-ADHs. Fig. shows the effects of various amines on the activity of PpADH. A wide range of primary C1–C8, amines in addition to iso-butylamine, di-methylamine, and tri-methylamine, activated PpADH. The oxidation activity was approximately 7.5 times higher with pentylamine than ethylamine at 10 mM. Pentylamine was the best activator. It is interesting that activation occurred with secondary or tertiary amines such as di-methylamine and tri-methylamine. In a similar earlier report, a dye-linked ADH from R. acidophila was activated by primary alkyl amines (C1–C9), and nonylamine had the highest affinity. Conversely, secondary and tertiary amines were not activators of the dye-linked ADH. The enzyme activity was higher with C4–C6 primary amines than with C1–C3 amines. Di-methylamine (pKa = 11), which is more basic than primary amines, was an activator; however, pyrrolidine (pKa = 11.4) and piperidine (pKa = 11.24), which are basically the same as di-methylamine, were not activators of PpADH (data not shown). Because the basicity of primary amines is rarely different from the length of the carbon number, the basicity was not related to the effect on the activated PpADH. These results suggest that the hydrophobic interaction is important for amine activators of this enzyme. However, the low enzyme activity with C7–C8 primary amines indicates that they have a very long alkyl chain, which makes it difficult to access the binding site cavity on the enzyme or reduces their solubility in the reaction solvent.
Fig. 1. Effects of various amines on the activity of PpADH. The final concentration of each amine used in the reaction mixture was 10 mM.
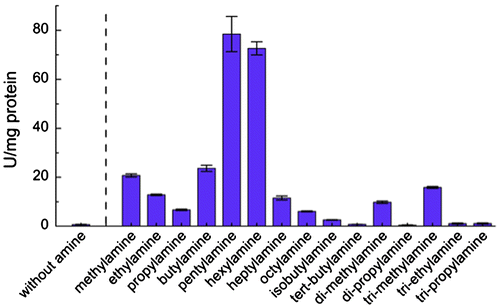
Fig. shows the effect of pentylamine and ethylamine concentrations on the activity of PpADH, which was measured with propanol at a saturation concentration of 10 mM. The enzyme activity was elevated at low concentrations of both amines, whereas higher concentrations partly inhibited the activity of PpADH at concentrations greater than 10 mM pentylamine or 30 mM ethylamine (Fig. ). Because of this inhibition, the data in Fig. were fit to the equation for substrate inhibition (Equation (1)). The Vmax value for pentylamine (Vmax = 107.8 (±17.8) U/mg) was two times higher than that for ethylamine (Vmax = 48.3 (±1.7) U/mg). The activation constants (KA) values for pentylamine and ethylamine were determined to be KA = 5.1 (±1.8) mM and KA = 14.9 (±0.7) mM, respectively. The inhibition constants (Ki) values for pentylamine and ethylamine were determined to be Ki = 37.6 (±10.3) mM and Ki = 112.5 (±11.3) mM, respectively. The KA or Ki value with ethylamine was approximately threefold higher than that with pentylamine. This result indicates that PpADH has specificity for amines as an activator, and the hydrophobic interaction contributes to the binding of amine activators to the binding site in the enzyme. It is likely that the amine affinity is dependent on the size and hydrophobicity of the active site cavity, and further research with other type I ADHs that have substrate specificities different from PpADH is needed to elucidate the effect of amines on type I ADHs.
Fig. 2. Effect of the ethylamine and pentylamine concentrations on the activity of PpADH. Concentration dependence on pentylamine (open square) and ethylamine (closed square) for propanol oxidation activity.
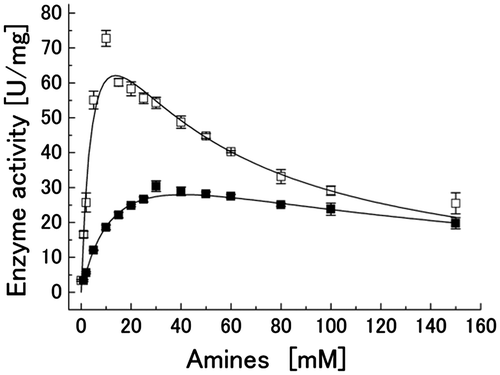
The results shown in Fig. indicate that the concentration of amines as activators was optimized. On the basis of these results, the kinetic parameters for ethanol and propanol were determined in the presence of an optimum pentylamine concentration of 10 mM (Table ) and compared to the kinetic data when using ethylamine as an activator.Citation21) In the presence of pentylamine, the affinity for substrates increased slightly, and kcat values were approximately twofold greater than those in the presence of ethylamine. These data suggest that pentylamine is directly involved in the catalytic cycle of alcohol oxidation by PpADH. There was no large difference with either ethanol or propanol. Similar results were also obtained using 10 mM pentanol as a substrate. The enzyme activities for pentanol oxidation were 57 ± 3 U/mg with 10 mM pentylamine and 21 ± 1 U/mg with 30 mM ethylamine. These results revealed that the alcohol-oxidizing activity of PpADH was facilitated by pentylamine as an activator regardless of the chain lengths of the alcohol substrates.
Table 1. Kinetic parameters of alcohol oxidation by PpADH in the presence of 30 mM ethylamine and 10 mM pentylamine.
In conclusion, we demonstrated that alcohol oxidation by PpADH was activated by a wide variety of amines, of which pentylamine had the highest activity. It is noteworthy that di-methylamine and tri-methylamine were also activators of this enzyme. Kinetic analysis of the activation by amines indicated that pentylamine had a more favorable effect than ethylamine as an activator to enhance the catalytic efficiency of alcohol oxidation by PpADH. This study found that type I ADHs are structurally dependent on amine activators, and individual type I ADHs have a specific amine activator. The mechanism of amine activation of type I ADHs is a question that remains to be answered.
Acknowledgments
The authors are grateful to Ms Patricia McGahan of Tokyo University of Agriculture and Technology for English proofreading during the preparation of this paper.
Funding
This research was supported by a grant from the Japan Society for the Promotion of Science [grant number 2125007].
Notes
Abbreviations: PQQ, pyrroloquinoline quinone; ADH, alcohol dehydrogenase; MDH, methanol dehydrogenase; PMS, phenazine methosulfate; DCPIP, 2,6-dichlorophenolindophenol.
References
- Goodwin PM, Anthony C. The quinoprotein dehydrogenases for methanol and glucose. Adv. Microb. Physiol. 1998;40:1–80.10.1016/S0065-2911(08)60129-0
- Toyama H, Mathews FS, Adachi O, Matsushita K. Quinohemoprotein alcohol dehydrogenases: structure, function, and physiology. Arch. Biochem. Biophys. 2004;428:10–21.10.1016/j.abb.2004.03.037
- Anthony C. The quinoprotein dehydrogenases for methanol and glucose. Arch. Biochem. Biophys. 2004;428:2–9.10.1016/j.abb.2004.03.038
- Rupp M, Gorisch H. Purification, crystallisation and characterization of quinoprotein ethanol dehydrogenase from Pseudomonas aeruginosa. Biol. Chem. Hoppe-Seyler. 1988;369:431–439.10.1515/bchm3.1988.369.1.431
- Keitel T, Diehl A, Knaute T, Stezowski JJ, Höhne W, Görisch H. X-ray structure of the quinoprotein ethanol dehydrogenase from Pseudomonas aeruginosa: basis of substrate specificity. J. Mol. Biol. 2000;297:961–974.10.1006/jmbi.2000.3603
- Toyama H, Fujii A, Matsushita K, Shinagawa E, Ameyama M, Adachi O. Three distinct quinoprotein alcohol dehydrogenases are expressed when Pseudomonas putida is grown on different alcohols. J. Bacteriol. 1995;177:2442–2450.
- Oubrie A, Rozeboom HJ, Kalk KH, Huizinga EG, Dijkstra BW. Crystal structure of quinohemoprotein alcohol dehydrogenase from Comamonas testosteroni: structural basis for substrate oxidation and electron transfer. J. Biol. Chem. 2002;277:3727–3732.10.1074/jbc.M109403200
- Toyama H, Chen ZW, Fukumoto M, Adachi O, Matsushita K, Mathews FS. Molecular cloning and structural analysis of quinohemoprotein alcohol dehydrogenase ADH-IIG from Pseudomonas putida HK5. J. Mol. Biol. 2005;352:91–104.10.1016/j.jmb.2005.06.078
- Yakushi T, Matsushita K. Alcohol dehydrogenase of acetic acid bacteria: structure, mode of action, and applications in biotechnology. Appl. Microbiol. Biotechnol. 2010;86:1257–1265.10.1007/s00253-010-2529-z
- Day D, Anthony C. Methanol dehydrogenase from Methylobacterium extorquens AM1. Methods Enzymol. 1990;188:210–216.10.1016/0076-6879(90)88035-9
- Harris TK, Davidson VL. A new kinetic model for the steady-state reactions of the quinoprotein methanol dehydrogenase from Paracoccus denitrificans. Biochemistry. 1993;32:4362–4368.10.1021/bi00067a028
- Anthony C, Zatman LJ. The microbial oxidation of methanol. 2. The methanol-oxidizing enzyme of Pseudomonas sp. M 27. Biochem. J. 1964;92:614–621.
- Anthony C. Bacterial oxidation of methane and methanol. Adv. Microb. Physiol. 1986;27:113–210.10.1016/S0065-2911(08)60305-7
- Wolf HJ, Hanson RS. Alcohol dehydrogenase from Methylobacterium organophilum. Appl. Environ. Microbiol. 1978;36:105–114.
- Goodwin MG, Anthony C. Characterization of a novel methanol dehydrogenase containing a Ba2+ ion at the active site. Biochem. J. 1996;318:673–679.
- Afolabi PR, Mohammed F, Amaratunga K, Majekodunmi O, Dales SL, Gill R, Thompson D, Cooper JB, Wood SP, Goodwin PM, Anthony C. Site-directed mutagenesis and X-ray crystallography of the PQQ-containing quinoprotein methanol dehydrogenase and its electron acceptor, cytochrome cL. Biochemistry. 2001;40:9799–9809.10.1021/bi002932l
- Hothi P, Basran J, Sutcliffe MJ, Scrutton NS. Effects of multiple ligand binding on kinetic isotope effects in PQQ-dependent methanol dehydrogenase. Biochemistry. 2003;42:3966–3978.10.1021/bi027282v
- Hothi P, Sutcliffe MJ, Scrutton NS. Kinetic isotope effects and ligand binding in PQQ-dependent methanol dehydrogenase. Biochem. J. 2005;388:123–133.
- Reddy SY, Bruice TC. Mechanisms of ammonia activation and ammonium ion inhibition of quinoprotein methanol dehydrogenase: a computational approach. Proc. Nat. Acad. Sci. USA. 2004;101:15887–15892.10.1073/pnas.0407209101
- Bamforth CW, Quayle JR. The dye-linked alcohol dehydrogenase of Rhodopseudomonas acidophila. Comparison with dye-linked methanol dehydrogenases. Biochem. J. 1978;169:677–686.
- Takeda K, Matsumura H, Ishida T, Samejima M, Igarashi K, Nakamura N, Ohno H. The two-step electrochemical oxidation of alcohols using a novel recombinant PQQ alcohol dehydrogenase as a catalyst for a bioanode. Bioelectrochemistry. 2013;94:75–78.10.1016/j.bioelechem.2013.08.001