Abstract
The aim of this study was to assess the adhesion of Bifidobacterium strains to acidic carbohydrate moieties of porcine colonic mucin. Mucins were extracted and purified via gel filtration chromatography followed by density-gradient ultracentrifugation. The presence of sulfated and sialylated carbohydrates in mucins was shown by enzyme-linked immunosorbent assays using PGM34 and HMC31 monoclonal antibodies (mAbs), respectively. Adhesion of Bifidobacterium strains to mucin preparations was markedly affected by the degree of purification. In eight of 22 strains, we observed increased adhesion to mucin preparations purified by ultracentrifugation. Moreover, in some of these eight strains, adhesion to mucin was reduced by pretreatment with sulfatase and/or sialidase, and competitively inhibited by pretreatment with PGM34 and/or HCM31 mAbs. Our results showed that some Bifidobacterium strains adhered to sulfo- and/or sialomucin and were able to recognize carbohydrate structures of the mAbs epitopes.
Graphical Abstract
The nitrogen source of the culture medium altered the alternative splicing pattern of serine-type carboxypeptidase ocpG mRNA in Aspergillus oryzae.
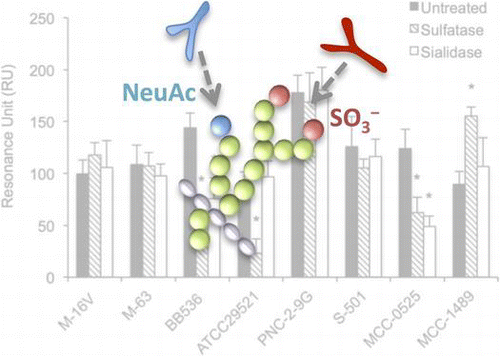
Bifidobacteria are gram-positive bacteria that represent one of the major genera found in the intestinal tract of humans and animals.Citation1) In some studies, consumption of specific bifidobacteria has been shown to be associated with immune modulation,Citation2) protection against pathogens,Citation2,3) and anti-aging effects.Citation4) Therefore, several Bifidobacterium strains are commonly used as probiotics.Citation5)
Bacterial adhesion to the mucosal surface through mucus layers is an important prerequisite for the colonization of probiotics in the intestinal lumen and provides a competitive advantage in this ecosystem.Citation6) Mucus layers consist mainly of gel-forming mucin, which are secreted by goblet cells on the intestinal mucosa.Citation7) The mucus layer is considered to be the first line of defense against bacteria in the intestinal tract and serves as the initiation surface for host–microbe interactions.Citation8,9) Mucins consist of a protein core and oligosaccharide side chains, and mucin oligosaccharides are joined to the protein core via an initial α-O-glycosidic linkage of acetylgalactosamine to the hydroxyl region of serine or threonine. Mucins can be broadly classified into neutral and acidic chemotypes, which are categorized further into sulfomucins or sialomucins based on the presence of terminal sulfate groups or sialic acid residues on the oligosaccharide chains, respectively.Citation10,11) The expression levels of sulfomucins and sialomucins have been shown to increase gradually along the human colon, where sulfation is predominant.Citation12) Thus, for probiotics, including bifidobacteria, adhesion to acidic mucins is thought to be an advantage for survival during transit and colonization in the intestinal tract.Citation13–15)
The interactions between Bifidobacterium strains and carbohydrate moieties of glycoconjugates, including glycolipids and mucins, have been described previously; for example, Bifidobacterium pseudolongum ATCC 25526 and Bifidobacterium adolescentis VTT E-001561 specifically adhere to α-1,4-linked glucose sugars,Citation16) while Bifidobacterium bifidum EB102 and Bifidobacterium longum SBT2928 bind to gangliotetraosylceramide.Citation17,18) A recent study showed that B. bifidum MCC-1092 binds to both sialic acid and sulfated carbohydrates of human colonic mucins because adhesion of the bacterium to mucins was reduced by treatment with sialidase and sulfatase and blocked by barium chloride.Citation14) However, little is known about the adhesion properties of bifidobacteria to sulfated or sialylated carbohydrate moieties of mucins.
In this study, we sought to evaluate the adhesion of 22 strains of bifidobacteria to sulfated and sialylated carbohydrate moieties of porcine colonic mucin. We first characterized mucins prepared from the porcine colon and then examined the adhesion of Bifidobacterium strains to the purified mucins. We also examined effects of enzymatic treatment of mucins and the presence of monoclonal antibodies (mAbs) targeting a specific carbohydrate structure on the adhesion of tested strains.
Materials and methods
Isolation and purification of porcine colonic mucin
Colon tissue samples of Landrace large white Duroc swine (24 weeks old) were obtained from a local slaughterhouse immediately after animals were sacrificed (Towada, Aomori, Japan). Porcine colonic mucin was isolated and purified as previously described using gel filtration chromatography and density-gradient ultracentrifugation.Citation19,20) Briefly, porcine colon tissues were lyophilized and powdered, and samples were then suspended in 50 mM Tris–HCl buffer (pH 7.2) containing 2% (v/v) TritonX-100. The mixture was centrifuged at 10,000 × g for 30 min at 4 °C and was then applied to a Sepharose CL-6B column (2.5 × 100 cm; GE Healthcare, NJ, USA). Void volume fractions were collected as mucin fractions and dialyzed against distilled water. Subsequently, the mucin fractions in 6 M guanidine hydrochloride (GuHCl) with a starting density of 1.4 g/mL of cesium chloride (CsCl; Sigma–Aldrich, MO, USA) were ultracentrifuged at 150,000 × g for 120 h at 20 °C. The hexose peaks in the fraction with a specific density of 1.35–1.45 g/mL were collected and dialyzed against distilled water. Hexose was measured by the phenol sulfuric acid method. Protein concentrations were measured using a BCA Protein Assay kit (Thermo Scientific, MA, USA). The prepared mucin (20 μg) was analyzed by reducing sodium dodecyl sulfate-polyacrylamide gel electrophoresis (SDS-PAGE; 7.5% acrylamide). Gels were stained with periodic acid/Schiff reagent.Citation21)
Sulfatase and sialidase treatment of mucin
Enzymatic treatment was performed on 10-mg lyophilized mucin batches by adding 10 mU of Aerobacter aerogenes sulfatase (Sigma–Aldrich) in 50 mM Tris–HCl (pH 7.1), 100 mM KCl, and 10 mM MgCl2, or 10 mU of Clostridium perfringens sialidase (Roche, IN, USA) in 50 mM acetate buffer (pH 5.0). Reaction mixtures were incubated for 5 h at 37 °C. After incubation, soluble mucins were separated by gel filtration using a Sepharose CL-6B column (1.0 × 50 cm) and then lyophilized.
Enzyme-linked immunosorbent assay (ELISA) using mAbs
For ELISA, PGM34 and HCM31 mAbs, which react with the Fucα1-2Galβ1-4GlcNAc(6SO3H)β- (6-sulfated blood-group H type 2 antigen) and NeuAcα2-3(GalNAcβ1-4)Galβ1-4GlcNAcβ-(Sda blood group antigen) sequences, respectively, were used to detect sulfated and sialylated oligosaccharides of mucins.Citation22,23) ELISAs were performed according to the method described by Tsubokawa et al.,Citation22) with the following modifications. A 96-well microplate was coated with 0.1–500 μg/mL of our prepared mucin samples (100 μL/well), followed by blocking with phosphate-buffered saline (PBS) containing 5% (w/v) skim milk for 2 h at room temperature. Next, 100 μL of PGM34 mAbs (1:100 dilution) and HCM31 mAbs (1:50 dilution) were added to each well, followed by incubation at room temperature for 1 h. Mouse IgM (1:100 or 1:50 dilutions, respectively) was used as a control. The wells were washed, alkaline phosphatase (AP)-conjugated anti-mouse IgG (Dako, Glostrup, Denmark; 1:2000 dilution) was added, and samples were incubated again at room temperature for 1 h. Wells were developed with a BluePhos MicroWell Substrate (KPL, MD, USA). The absorbance was then measured at 620 nm.
Dot blot assay using mAbs
Mucins were transferred to nitrocellulose membranes (Whatman, Kent, UK) and blocked in PBS containing 5% (w/v) skim milk for 2 h, followed by incubation with PGM34 (1:100 dilution) or HCM31 (1:50 dilution) mAbs in PBS-Tween (PBS-T) containing 1% (w/v) skim milk overnight at 4 °C. Mouse IgM was used as a control. After washing three times with PBS-T, membranes were incubated with AP-conjugated anti-mouse IgG (Dako; 1:1500 dilution) in PBS-T at room temperature for 1 h. After washing, the blots were developed using a BCIP/NBT liquid substrate system (Sigma–Aldrich).
Bacterial strains
Twenty-two strains of Bifidobacterium were obtained from different origins (Table ). All strains were grown in de Man–Rogosa–Sharp (MRS) broth (BD Difco, Le Pont de Claix, France; pH 6.5) supplemented with 0.05% (w/v) l-cysteine (Wako, Japan) at 37 °C, under anaerobic conditions, until the OD600 reached about 2.0.
Table 1. Bifidobacterium strains used in this study.
Surface plasmon resonance (SPR)-based adhesion assay for measurement of bifidobacterial adhesion to mucins
SPR-based adhesion assays were performed using a Biacore-X system (GE Healthcare) according to the method described by Uchida et al.,Citation24) with the following modifications. Bacterial cells were suspended in HBS-EP buffer (10 mM HEPES, 0.15 M NaCl, 3.4 mM EDTA, 0.005% surfactant P20, pH 7.2) at a concentration of 1 × 108 colony-forming units (cfu)/mL. Mucins were immobilized as a ligand onto a CM5 sensor chip (GE Healthcare) at about 3500 resonance units (RU). The analyte, 100 μL of bacterial cell suspension, was then injected. The RUs were measured at the start of dissociation without further sample addition. The flow cell surface of the sensor chip was regenerated by elution with 2 M NaCl at a flow rate of 20 μL/min, for 60 s.
Adhesion and inhibition assays using microplates
Adhesion assays were performed using microplates as described previously.Citation15) Briefly, 96-well plates were coated with mucins. The bacterial suspension was added to each well at a concentration of 1 × 108 cfu/mL. For inhibition of bacterial adhesion using mAbs, mucins were pretreated with PGM34 or HCM31 mAbs (1:100 and 1:50 dilutions, respectively) for 60 min at room temperature. The wells were washed twice with PBS containing bovine serum albumin (BSA; Sigma–Aldrich). Next, 100 μL of bacterial suspension was added to each well. The plate was incubated for 60 min at 37 °C. After washing twice, 0.01% (v/v) Triton X-100 in PBS was added to each well, and the bacterial cells were suspended by vigorous pipetting. Serial dilutions of suspended bacteria were plated on MRS agar and incubated at 37 °C anaerobically. After 24 h of incubation, CFU on the plates were counted. Adhesion to mucin was expressed as a percentage calculated from three independent experiments, as follows: 100 × (number of adhesive bacteria/number of bacteria inoculated).
Statistical analyses
PRISM6 (GraphPad Software) software was used for all statistical analyses. Significant differences were determined using Student’s t-test or one-way analysis of variance (ANOVA) with the Dunnett’s post hoc test. “n” represents the number of individual experiments. Differences with p-values of less than 0.05 were considered significant.
Results
Purification and characterization of mucins
In the present study, mucin preparations were purified via gel filtration chromatography followed by density-gradient ultracentrifugation. The void volume fraction of gel filtration chromatography (Fr. 1 indicated by the arrow in Fig. (a)) was collected and then subjected to density-gradient ultracentrifugation in CsCl/GuHCl. The fraction with the highest concentration of hexose and a specific density between 1.35 and 1.45 g/mL (Fr. 2 indicated by the arrow in Fig. (b)) was then collected.
Fig. 1. Purification and characterization of porcine colonic mucins.
Notes: Porcine colonic mucins were purified using gel filtration chromatography followed by density-gradient ultracentrifugation. Extracts from the porcine intestine were applied to a gel filtration column. Fraction 1 (Fr. 1, fraction numbers 10–18) was recovered (a) and then subjected to CsCl density-gradient ultracentrifugation. Fraction 2 (Fr. 2), showing a specific density between 1.35 and 1.45 g/mL, was also recovered (b). Hexose and protein were monitored at absorbance wavelength of 490 nm (-●-) and 562 nm (-○-), respectively. The specific density is indicated in g/mL (-△-). (c) Fr. 1 (lane 1) and Fr. 2 (lane 2) were subjected to SDS-PAGE and then stained by periodic acid/Schiff, as described in the Materials and Methods section.
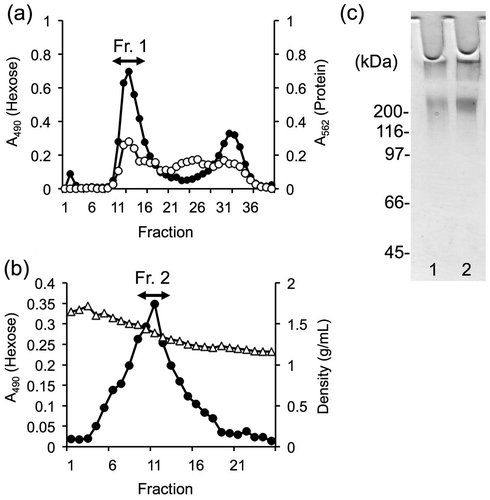
To characterize the mucin fractions, equal amounts of each fraction (as determined by dry weight) were subjected to SDS-PAGE and stained with periodic acid/Schiff reagent, which is specific for carbohydrates (Fig. (c)). High-molecular-weight mucins were observed in the stacking gel for both fractions. In contrast, the average hexose/protein (w/w) ratio was markedly increased in Fr. 2 compared to Fr.1 (0.21 for Fr. 1 to 0.77 for Fr. 2), indicating that density-gradient ultracentrifugation produced mucin with higher purity than that produced by single gel filtration chromatography. Henceforth, mucin preparations from Fr. 1 and Fr. 2 were termed as “crude mucin” and “purified mucin,” respectively.
Characterization of mucins using mAbs
We next investigated the presence of sulfated and sialylated oligosaccharides of mucins by ELISA and dot blot analyses using PGM34 and HCM31 mAbs. For ELISA, both mAbs reacted with purified mucin in a dose-dependent manner (Fig. (a)). A 1:100 dilution of PGM34 mAb and a 1:50 dilution of HCM31 mAb resulted in the best sigmoidal reaction curve, while IgM (1:100 or 1:50 dilution) bound weakly to mucins or did not bind at all. Moreover, we examined effects of enzymatic treatment of purified mucin with sulfatase or sialidase on the binding of mAbs. After sulfatase or sialidase treatment, the reactivity of both mAbs to purified mucins was markedly reduced as compared to binding to untreated mucins (Fig. (b)). These observations indicated that sulfated and sialylated oligosaccharides, especially 6-sulfated blood-group H type 2 antigen and Sda blood group antigen, were present in porcine colonic mucins.
Fig. 2. Binding of PGM34 and HCM31 mAbs to immobilized mucins.
Notes: (a) Mucins were immobilized on an ELISA plate, and the indicated concentration of PGM34 (-●-) or HCM31 (-○-) mAbs was added to each well. IgM was used as a control (-▲-, -△-). The log values of the concentration of mucin (μg/mL) are shown. (b) Effects of enzymatic treatment with sulfatase or sialidase on binding of PGM34 (■) and HCM31 (□) mAbs to mucins. Mucins were immobilized on an ELISA plate and nitrocellulose membrane. Binding tests were performed as described in the text. For all studies, error bars indicate standard deviations (n = 5).
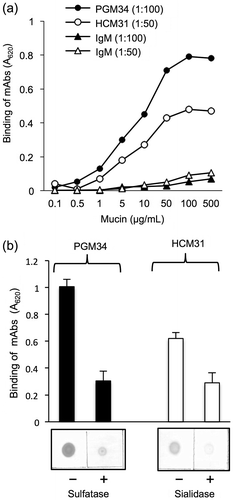
Adhesion of Bifidobacterium to mucins
Next, we examined the adhesion of 22 Bifidobacterium strains to mucins using Biacore assays. Bifidobacterium strains varied markedly in their abilities to adhere to mucins, with substantial differences in RUs, which ranged from 31.5 ± 22.9 to 190.6 ± 10.8 RU (Fig. ). The adhesion of the tested strains to purified mucin was considerably different from that to crude mucin. As shown in Fig. , of the 22 strains, B. pseudolongum subsp. pseudolongum PNC-2-9G showed the strongest adhesion to purified mucin. Eight strains, i.e. Bifidobacterium breve M-16 V, Bifidobacterium infantis M-63, B. longum BB536, B. bifidum ATCC29521, B. pseudolongum subsp. pseudolongum PNC-2-9G, Bifidobacterium thermophilum S-501, Bifidobacterium animalis subsp. animalis MCC-1489, and B. animalis subsp. lactis MCC-0525, showed significantly greater adhesion to purified mucin than to crude mucin. On the other hand, B. bifidum ATCC15696 and B. animalis subsp. lactis JCM1253 showed considerably stronger adhesion to crude mucin than to purified mucin. These results showed that the adhesion of Bifidobacterium strains to mucins was strain-specific and affected by the degree of mucin purification.
Fig. 3. Adhesion of Bifidobacterium to mucins.
Notes: The adhesion of 22 strains of Bifidobacterium to crude mucin (white bars) and purified mucin (black bars) was evaluated using a Biacore system. Strains that bound significantly more strongly to purified mucin than to crude mucin, as determined by Student’s t-test (*p < 0.05, **p < 0.01), are indicated by a single asterisk, while strains showing significantly stronger binding to crude mucin than to purified mucin are indicated with a dagger (†p < 0.05). Error bars indicate standard deviations (n = 3).
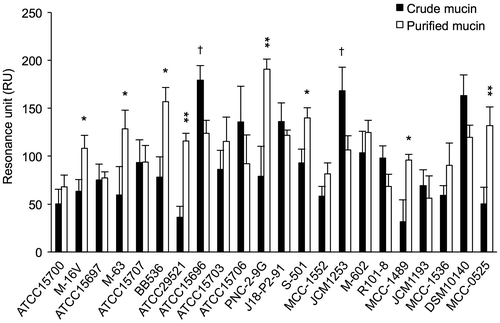
Adhesion of Bifidobacterium strains to sulfomucin and sialomucin
We examined the effects of enzymatic treatment of purified mucin with sulfatase or sialidase on the adhesion of the eight above-listed strains of Bifidobacterium that showed high adhesion to purified mucin. As shown in Fig. , treatment with sulfatase significantly reduced the adhesion of B. longum BB536, B. bifidum ATCC 29521, and B. animalis subsp. lactis MCC-0525 strains compared with adhesion to untreated mucin. Similarly, sialidase treatment significantly reduced the adhesion of B. longum BB536 and B. animalis subsp. animalis MCC-0525. In contrast, sulfatase treatment of mucin significantly increased the adhesion of B. animalis subsp. animalis MCC-1489.
Fig. 4. Effects of treatment of mucins with sulfatase or sialidase on adhesion of Bifidobacterium strains.
Notes: The purified mucins were pretreated with sulfatase (▧) or sialidase (□). Adhesion assays of the bacteria to the mucins were performed as described in the Materials and Methods. Asterisks indicate that the number of bacteria bound to the treated mucin was significantly different (*p < 0.05) from that bound to the untreated control mucin (■), as determined by one-way ANOVA with Dunnett’s post hoc test. Error bars indicate standard deviations (n = 5).
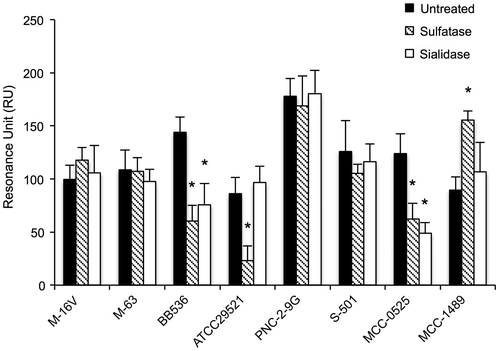
Next, we examined whether the adhesion of Bifidobacterium species could be reduced by pretreatment of mucins with PGM34 or HCM31 mAb. As shown in Fig. , purified mucin treated with PGM34 mAb exhibited significantly reduced adhesion with B. bifidum ATCC29521 as compared to that of untreated purified mucin. Additionally, pretreatment of purified mucin with PGM34 or HCM31 mAb significantly reduced the adhesion of B. longum BB536. Adhesion of B. animalis subsp. lactis MCC0525 to mucins was also significantly reduced by pretreatment with HCM31 mAb, but not PGM34 mAb. In addition, pretreatment with mouse IgM had no effect on the adhesion of Bifidobacterium.
Fig. 5. Inhibition of adhesion of Bifidobacterium strains to mucins in the presence of PGM34 or HCM31 mAbs.
Notes: The purified mucins were pretreated with PGM34 mAb (⊡), HCM31 mAb (□), and IgM (▧). Adhesion tests of the bacteria to the mucins were performed as described in the text. Asterisks indicate that the number of bacteria bound to treated mucin was significantly different (*p < 0.05) compared to that bound to antibody-free samples (■), as determined by one-way ANOVA with Dunnett’s post hoc test. Error bars indicate standard deviations (n = 3).
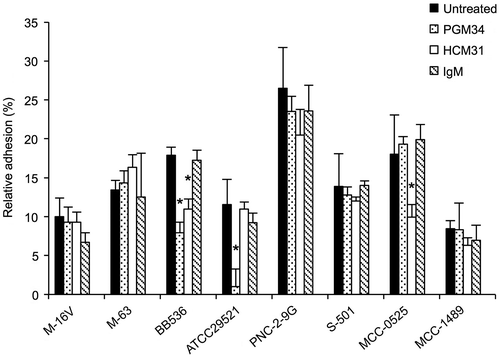
Discussion
In this study, we evaluated the adhesion of Bifidobacterium strains to sulfated or sialylated carbohydrate moieties of porcine colonic mucins. Porcine mucins have been used as a model for testing the in vitro adhesion capacity of probiotic bacteria for many years.Citation15,25–28) Mucus layers are a heterogeneous mixture of antibacterial proteins, IgA, nucleic acids, and undigested materials;Citation29) however, little is known about the effects of the degree of mucin purification on the adhesive properties of probiotic bacteria.
We were able to prepare mucins with high purity from the porcine colon using a series of purification steps including gel filtration chromatography followed by density-gradient ultracentrifugation. Interestingly, the degree of the mucin purification directly affected bacterial adhesion. Eight strains of Bifidobacterium showed higher adhesion to mucins prepared after density-gradient ultracentrifugation compared to that after gel filtration chromatography; however, two other strains adhered more strongly to the mucin preparation after gel filtration chromatography. The purification step of density-gradient ultracentrifugation in CsCl/GuHCl was expected to remove biomolecules such as nucleic acids, proteins, and lipids from the prepared mucin.Citation30) It is considered that removal of the biomolecules enhances the adhesion level of Bifidobacterium strains, which can bind to the carbohydrate moieties. Therefore, the degree of mucin purification should be considered when evaluating the adhesion properties of bacteria to the carbohydrate moieties of mucins.
Host-specific adhesion is regarded as a desirable property for probiotic bacteria.Citation31) On the other hand, the adhesion properties of probiotic bacteria to mucus are thought to be more dependent on the strain than on the host.Citation32,33) Our results showed no clear correlation between the host of origin of the bifidobacteria and the origin of the mucin. For example, B. longum BB536 and B. pseudolongum subsp. pseudolongum PNC-2-9G, originating from humans and swine, respectively, showed high adhesion to porcine colonic mucin. In contrast, Bifidobacterium suis MCC-1552, originating from swine, showed reduced adhesion.
In this study, the adhesion assay of sulfatase-pretreated mucins showed that the terminal sulfate groups of mucin sugar chains were responsible for the adhesion of B. longum BB536, B. bifidum ATCC29521, and B. animalis subsp. lactis MCC-0525 strains to mucin. Furthermore, the assay of sialidase-treated mucins indicated that B. longum BB536 and B. animalis subsp. lactis MCC-0525 were likely recognize the terminal sialic acid moieties in addition to the sulfate groups on mucin sugar chains. Thus, these Bifidobacterium strains have the ability to recognize sulfated or sialylated carbohydrates present in mucins. In case of B. longum BB536 and B. bifidum ATCC29521, the findings of assays studying the effect of enzymatic treatments agreed with those of the adhesion inhibition test performed using PGM34 and HCM31 mAbs, which react the 6-sulfated blood-group H type 2 antigen and the Sda blood group antigen, respectively.Citation22,23) The epitope of PGM34 or HCM31 may be essential for the adhesion of B. longum BB536 and B. bifidum ATCC29521. Although pretreatment with sulfatase reduced the adhesion of B. animalis subsp. lactis MCC-0525 to immobilized mucin, the adhesion remained unchanged after pretreatment with PGM34 mAbs. Therefore, we speculate that B. animalis subsp. lactis MCC-0525 may recognize sulfated carbohydrates other than the PGM34 mAb epitope. Interestingly, adhesion of B. animalis subsp. animalis MCC-1489 was significantly increased after sulfatase treatment, suggesting that the binding sites may be exposed after sulfatase treatment, as described by Huang et al.,Citation14) who showed similar interactions in the adhesion of Lactobacillus ME-522 and B. bifidum MCC-1092 to mucin. However, additional studies are needed to characterize the binding properties of B. animalis subsp. animalis MCC-1489.
Bacterial adhesion to acidic carbohydrate moieties of glycoconjugates mediated by specific adhesins has been reported. For example, non-fimbrial adhesin coli surface antigen 6 of Escherichia coliCitation34) and soluble neutrophil-activating protein and heat shock protein (Hsp) of Helicobacter pylori bind to sulfated glycolipids.Citation35,36) In addition, sialic acid-binding adhesin (Sab A) of H. pylori binds to sialyl Lewis X antigen in the gastric epithelium.Citation37) More recently, the mucin-binding domain (MucBD)-associated domain (MUBAD) of Lactobacillus reuteri was shown to bind to sulfated carbohydrates of human colonic mucin.Citation38) However, in this study, we were not able to unequivocally identify the bacterial molecular determinants involved in the mechanisms of adhesion to sulfated or sialylated carbohydrates. Our recent study showed that elongation factor Tu (EF-Tu), localized on the cell surface of L. reuteri JCM1081, bound to sulfated carbohydrates of mucins.Citation15) The binding characteristics of EF-Tu in bifidobacteria to sulfated carbohydrates of mucins are currently under further investigation.
In conclusion, we demonstrated that the degree of mucin purification influenced bifidobacterial adhesion properties and that several strains of Bifidobacterium had the ability to recognize sulfated and/or sialylated carbohydrates in mucins. In future studies, we will perform in vivo Bifidobacterium colonization studies to further elucidate the relevance and mechanisms of these processes.
Acknowledgments
We would like to thank Mr Hirotaka SEIMIYA and Ms Tomomi MASUOKA for technical help. This work was supported by a Grant-in-Aid for Scientific Research C (21580334 and 24580397) from the Japan Society for the Promotion of Science (JSPS) and Kitasato Research Project for Lactic Acid Bacteria.
Notes
Abbreviations: BSA, bovine serum albumin; CFU, colony-forming unit; CsCl, cesium chloride; ELISA, enzyme-linked immunosorbent assay; GuHCl, guanidine hydrochloride; mAb, monoclonal antibody; MRS, de Man–Rogosa–Sharpe; PBS, phosphate-buffered saline; RU, resonance unit; SPR, surface plasmon resonance.
References
- Turroni F, Van Sinderen D, Ventura M. Genomics and ecological overview of the genus Bifidobacterium. Int. J. Food Microbiol. 2011;149:37–44.10.1016/j.ijfoodmicro.2010.12.010
- Fanning S, Hall LJ, Cronin M, Zomer A, MacSharry J, Goulding D, Motherway MO, Shanahan F, Nally K, Dougan G, van Sinderen D. Bifidobacterial surface-exopolysaccharide facilitates commensal-host interaction through immune modulation and pathogen protection. Proc. Nat. Acad. Sci. USA. 2012;109:2108–2113.10.1073/pnas.1115621109
- Fukuda S, Toh H, Hase K, Oshima K, Nakanishi Y, Yoshimura K, Tobe T, Clarke JM, Topping DL, Suzuki T, Taylor TD, Itoh K, Kikuchi J, Morita H, Hattori M, Ohno H. Bifidobacteria can protect from enteropathogenic infection through production of acetate. Nature. 2011;469:543–547.10.1038/nature09646
- Matsumoto M, Kurihara S, Kibe R, Ashida H, Benno Y. Longevity in mice is promoted by probiotic-induced suppression of colonic senescence dependent on upregulation of gut bacterial polyamine production. PloS One. 2011;6:e23652.10.1371/journal.pone.0023652
- Kleerebezem M, Vaughan EE. Probiotic and gut lactobacilli and bifidobacteria: molecular approaches to study diversity and activity. Annu. Rev. Microbiol. 2009;63:269–290.10.1146/annurev.micro.091208.073341
- Lebeer S, Vanderleyden J, De Keersmaecker SCJ. Genes and molecules of lactobacilli supporting probiotic action. Microbiol. Mol. Biol. Rev. 2008;72:728–764.10.1128/MMBR.00017-08
- Johansson MEV, Larsson JMH, Hansson GC. The two mucus layers of colon are organized by the MUC2 mucin, whereas the outer layer is a legislator of host-microbial interactions. Proc. Nat. Acad. Sci. USA. 2011;108:4659–4665.10.1073/pnas.1006451107
- Moran AP, Gupta A, Joshi L. Sweet-talk: role of host glycosylation in bacterial pathogenesis of the gastrointestinal tract. Gut. 2011;60:1412–1425.10.1136/gut.2010.212704
- Bergstrom KSB, Xia L. Mucin-type O-glycans and their roles in intestinal homeostasis. Glycobiology. 2013;23:1026–1037.10.1093/glycob/cwt045
- Brockhausen I. Sulphotransferases acting on mucin-type oligosaccharides. Biochem. Soc. Trans. 2003;31:318–325.10.1042/BST0310318
- Derrien M, van Passel MW, van de Bovenkamp JH, Schipper RG, de Vos WM, Dekker J. Mucin-bacterial interactions in the human oral cavity and digestive tract. Gut Microbes. 2010;1:254–268.10.4161/gmic
- Croix JA, Carbonero F, Nava GM, Russell M, Greenberg E, Gaskins HR. On the relationship between sialomucin and sulfomucin expression and hydrogenotrophic microbes in the human colonic mucosa. PloS One. 2011;6:e24447.10.1371/journal.pone.0024447
- Mukai T, Asasaka T, Sato E, Mori K, Matsumoto M, Ohori H. Inhibition of binding of Helicobacter pylori to the glycolipid receptors by probiotic Lactobacillus reuteri. FEMS Immunol. Med. Microbiol. 2002;32:105–110.10.1111/fim.2002.32.issue-2
- Huang IN, Okawara T, Watanabe M, Kawai Y, Kitazawa H, Ohnuma S, Shibata C, Horii A, Kimura K, Taketomo N, Xiao JZ, Iwatsuki K, Saito T. New screening methods for probiotics with adhesion properties to sialic acid and sulphate residues in human colonic mucin using the Biacore assay. J. Appl. Microbiol. 2013;114:854–860.10.1111/jam.2013.114.issue-3
- Nishiyama K, Ochiai A, Tsubokawa D, Ishihara K, Yamamoto Y, Mukai T. Identification and characterization of sulfated carbohydrate-binding protein from Lactobacillus reuteri. PloS One. 2013;8:e83703.10.1371/journal.pone.0083703
- Crittenden R, Laitila A, Forssell P, Matto J, Saarela M, Mattila-Sandholm T, Myllarinen P. Adhesion of bifidobacteria to granular starch and its implications in probiotic technologies. Appl. Environ. Microbiol. 2001;67:3469–3475.10.1128/AEM.67.8.3469-3475.2001
- Fujiwara S, Hashiba H, Hirota T, Forstner JF. Proteinaceous factor(s) in culture supernatant fluids of bifidobacteria which prevents the binding of enterotoxigenic Escherichia coli to gangliotetraosylceramide. Appl. Environ. Microbiol. 1997;63:506–512.
- Mukai T, Kaneko S, Matsumoto M, Ohori H. Binding of Bifidobacterium bifidum and Lactobacillus reuteri to the carbohydrate moieties of intestinal glycolipids recognized by peanut agglutinin. Int. J. Food Microbiol. 2004;90:357–362.10.1016/S0168-1605(03)00317-9
- Komuro Y, Ishihara K, Ishii K, Ota H, Katsuyama T, Saigenji K, Hotta K. A separating method for quantifying mucus glycoprotein localized in the different layer of rat gastric mucosa. Gastroenterol. Jpn. 1992;27:466–472.
- Kodaira H, Ishihara K, Hotta K, Kagoshima M, Shimada H, Ishii K. Reaction of various lectins to mucin derived from the different layers of rat gastric mucosa: comparison of enzyme-linked lectin binding assay with lectin histochemistry. Biol. Pharm. Bull. 2000;23:1173–1179.10.1248/bpb.23.1173
- Zacharius RM, Zell TE, Morrison JH, Woodlock JJ. Glycoprotein staining following electrophoresis on acrylamide gels. Anal. Biochem. 1969;30:148–152.10.1016/0003-2697(69)90383-2
- Tsubokawa D, Goso Y, Sawaguchi A, Kurihara M, Ichikawa T, Sato N, Suganuma T, Hotta K, Ishihara K. A monoclonal antibody, PGM34, against 6-sulfated blood-group H type 2 antigen, on the carbohydrate moiety of mucin. FEBS J. 2007;274:1833–1848.10.1111/j.1742-4658.2007.05731.x
- Tsubokawa D, Goso Y, Kawashima R, Ota H, Nakamura T, Nakamura K, Sato N, Kurihara M, Dohi T, Kawamura YI, Ichikawa T, Ishihara K. The monoclonal antibody HCM31 specifically recognises the Sd(a) tetrasaccharide in goblet cell mucin. FEBS Open Bio. 2012;2:223–233.10.1016/j.fob.2012.07.006
- Uchida H, Fujitani K, Kawai Y, Kitazawa H, Horii A, Shiiba K, Saito K, Saito T. A new assay using surface plasmon resonance (SPR) to determine binding of the Lactobacillus acidophilus group to human colonic mucin. Biosci. Biotechnol. Biochem. 2004;68:1004–1010.10.1271/bbb.68.1004
- Shi T, Nishiyama K, Nakamata K, Aryantini NPD, Mikumo D, Oda Y, Yamamoto Y, Mukai T, Sujaya IN, Urashima T, Fukuda K. Isolation of potential probiotic Lactobacillus rhamnosus strains from traditional fermented mare milk produced in Sumbawa Island of Indonesia. Biosci. Biotechnol. Biochem. 2012;76:1897–1903.10.1271/bbb.120385
- Izquierdo E, Medina M, Ennahar S, Marchioni E, Sanz Y. Resistance to simulated gastrointestinal conditions and adhesion to mucus as probiotic criteria for Bifidobacterium longum strains. Curr. Microbiol. 2008;56:613–618.10.1007/s00284-008-9135-7
- Laparra JM, Sanz Y. Comparison of in vitro models to study bacterial adhesion to the intestinal epithelium. Lett. Appl. Microbiol. 2009;49:695–701.10.1111/lam.2009.49.issue-6
- Iniguez-Palomares C, Jimenez-Flores R, Vazquez-Moreno L, Ramos-Clamont-Montfort G, Acedo-Felix E. Protein-carbohydrate interactions between Lactobacillus salivarius and pig mucins. J. Anim. Sci. 2011;89:3125–3131.10.2527/jas.2010-2996
- Patsos G, Corfield A. Management of the human mucosal defensive barrier: evidence for glycan legislation. Biol. Chem. 2009;390:581–590.
- Sheehan JK, Carlstedt I. The effect of guanidinium chloride on the behaviour of human cervical-mucus glycoproteins. Evidence for unfolding regions of ordered structure in 6M-guanidinium chloride. Biochem. J. 1984;221:499–504.
- Oh PL, Benson AK, Peterson DA, Patil PB, Moriyama EN, Roos S, Walter J. Diversification of the gut symbiont Lactobacillus reuteri as a result of host-driven evolution. ISME J. 2010;4:377–387.10.1038/ismej.2009.123
- Rinkinen M, Westermarck E, Salminen S, Ouwehand AC. Absence of host specificity for in vitro adhesion of probiotic lactic acid bacteria to intestinal mucus. Vet. Microbiol. 2003;97:55–61.10.1016/S0378-1135(03)00183-4
- Mackenzie DA, Jeffers F, Parker ML, Vibert-Vallet A, Bongaerts RJ, Roos S, Walter J, Juge N. Strain-specific diversity of mucus-binding proteins in the adhesion and aggregation properties of Lactobacillus reuteri. Microbiology. 2010;156:3368–3378.10.1099/mic.0.043265-0
- Jansson L, Tobias J, Jarefjäll C, Lebens M, Svennerholm AM, Teneberg S. Sulfatide recognition by colonization factor antigen CS6 from enterotoxigenic Escherichia coli. PloS One. 2009;4:e4487.10.1371/journal.pone.0004487
- Huesca M, Borgia S, Hoffman P, Lingwood CA. Acidic pH changes receptor binding specificity of Helicobacter pylori: a binary adhesion model in which surface heat shock (stress) proteins mediate sulfatide recognition in gastric colonization. Infect. Immun. 1996;64:2643–2648.
- Teneberg S, Miller-Podraza H, Lampert HC, Evans DJ Jr., Evans DG, Danielsson D, Karlsson KA. Carbohydrate binding specificity of the neutrophil-activating protein of Helicobacter pylori. J. Biol. Chem. 1997;272:19067–19071.10.1074/jbc.272.30.19067
- Mahdavi J, Sondén B, Hurtig M, Olfat FO, Forsberg L, Roche N, Angstrom J, Larsson T, Teneberg S, Karlsson KA, Altraja S, Wadström T, Kersulyte D, Berg DE, Dubois A, Petersson C, Magnusson KE, Norberg T, Lindh F, Lundskog BB, Arnqvist A, Hammarström L, Borén T. Helicobacter pylori SabA adhesin in persistent infection and chronic inflammation. Science. 2002;297:573–578.10.1126/science.1069076
- Coic Y-M, Baleux F, Poyraz O, Thibeaux R, Labruyere E, Chretien F, Sobhani I, Lazure T, Wyplosz B, Schneider G, Mulard L, Sansonetti PJ, Marteyn BS. Design of a specific colonic mucus marker using a human commensal bacterium cell surface domain. J. Biol. Chem. 2012;287:15916–15922.10.1074/jbc.M111.310003