Abstract
The Gram-positive bacterium Bacillus subtilis has a thick cell wall. The cell wall contains various proteins, both for secretion and for peptidoglycan (PG) maintenance. Penicillin-binding proteins for PG synthesis, PG hydrolases (autolysins), and regulator proteins for the autolysins are the known components of the PG maintenance system. YqgA was identified as an abundant protein attached to the cell wall of B. subtilis through a proteomics analysis. The YqgA protein was localized at cell division sites during the transition period between the exponential and the stationary phases. YqgA localization was affected by mutations in the dl-endopeptidases (DLEPases), which are the autolysins involved in cell morphogenesis. Furthermore, yqgA mutations on a background of defective DLEPases led to delays in cell growth and cell morphological changes. These results demonstrate that yqgA is genetically related to the genes encoding DLEPases involved in cell morphogenesis.
Graphical Abstract
YqgA is an abundant cell wall protein in B. subtilis. Our results indicated, yqgA is genetically related to autolysins involved in cell morphogenesis.
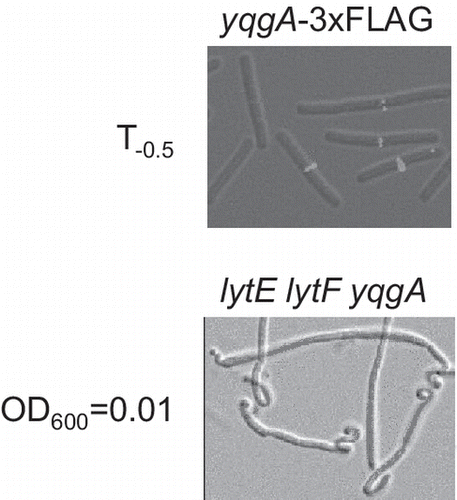
Introduction
The cell envelope of Gram-positive bacteria consists of a cytoplasmic membrane and a thick cell wall composed primarily of the polymer peptidoglycan (PG). PG forms a mesh-like structure and behaves as an exoskeleton for bacterial cells. Bacterial cells can tolerate various environmental stresses, including osmotic stress, due to the properties of PG, and the PG sacculus determines the bacterial cell shape. In addition to PG, the cell wall also consists of covalently attached anionic polymers, and proteins to be secreted and involved in cell wall maintenance.Citation1) These maintenance proteins include cell wall-degrading enzymes (autolysins) and regulatory proteins for the autolysins. The autolysins participate in cell morphogenesis and cell wall turnover.Citation2,3) Regulatory proteins for the autolysins that are both modifiers of PG and inhibitors of autolysins have been reported.Citation4–7)
More than 35 autolysins have been identified in the genome of B. subtilis.Citation2) They are classified into seven groups based on the position of their PG digestion site. In the groups, dl-endopeptidases (DLEPase), which hydrolyze the linkage of d-γ-glutamyl-meso-diaminopimelic acid in PG, are only reported to be involved in cell morphogenesis.Citation8–11) The DLEPases LytE, LytF, and CwlS are localized to cell division sites, and a triple-mutant of lytE, lytF, and cwlS has defects in cell separation.Citation8,9) Therefore, the functions of these three enzymes are to digest PG at the cell division site for cell separation. LytE and CwlO, which are DLEPases, are involved in cell elongation. LytE is localized to cell division sites, as described above, and is also found in the lateral cell walls, whereas CwlO is detected at the cellular sidewall only.Citation11,12) Double-depletion of LytE and CwlO localizing at the cellular sidewall results in synthetic lethality, and the cells are defective in cell elongation.Citation10,11) Thus, these two DLEPases are involved in cell elongation. Based on these observations, these DLEPases are proposed to be key players in cell morphogenesis.
The YqgA protein was identified through a cell wall proteome analysis of Bacillus subtilis.Citation1) YqgA is an abundant protein retained in the cell wall. It has been reported that YqgA expression is strongly induced by cold shock from 37 to 18 °C; however, the function of YqgA during cold shock is unclear.Citation13) In addition, YqgA was co-purified with SpoIIIJ or YqjG, which are Oxa1p-like proteins involved in membrane protein biogenesis.Citation14) However, the authors expected that the role of YqgA for membrane biogenesis might be specific only for a few narrow group of species, because YqgA has a limited distribution among a part of the genus Bacillus. In this paper, we performed a characterization of YqgA in B. subtilis and revealed that yqgA genetically relates to the genes encoding DLEPases involved in cell morphology.
Materials and methods
Bacterial strains, plasmids, and medium
The plasmids and the bacterial strains of B. subtilis and E. coli used in this study are listed in Table . B. subtilis 168 was used as the parental strain throughout this study. The strains were cultured in Luria-Bertani (LB) mediumCitation15) at 37 °C with ampicillin, erythromycin, chloramphenicol, or IPTG at final concentrations of 100 μg/mL, 0.3 μg/mL, 5 μg/mL, or 0.3 mM, respectively, when required. DNA manipulations and E. coli transformations were performed using standard methods.Citation15) B. subtilis transformation was performed using the conventional transformation procedure.Citation16)
Table 1. Bacterial strains and plasmids.
To measure a growth curve, fresh medium was inoculated with an overnight culture of B. subtilis to give an initial absorbance equivalent to an OD600 of 0.001. The transition point (T0) between the exponential and stationary growth phases was determined from the growth curve, and Tx was used to indicate the number of hours (x) after the transition point.
Plasmid construction
The plasmid pCA3FLYqgA was constructed to express the C-terminal 3×FLAG epitope-tagged YqgA fusion protein. Specifically, B. subtilis 168 chromosomal DNA was used as a template to PCR-amplify a fragment of yqgA without a stop codon, using the primers YQGA-HF (5′-gccgaagcttctattgatgcttacgccg-3′) and YQGA-BR (5′-gccgggatcctcttttttgtgggttatgt-3′). The amplified yqgA fragment was cloned into the HindIII and BamHI restriction enzyme sites of pCA3FL.
To construct a yqgA null mutant (∆YQ), the pT7-yqgA plasmid was prepared. The 5′-region of yqgA was amplified using primers yqgA-11 (5′-gataataagggtaactattgccaagcttccggtgcaactaccaaagttaac-3′) and yqgA-12 (5′-ggggggatcctcctttacaatgtcatccgg-3′). The 3′-region of yqgA was amplified using primers yqgA-6 (5′-aaaagcatgctttaaaagagattcccgtcc-3′) and yqgA-10 (5′-ccacatacctataatcgatggatccccattataaggggaaagctaaggg-3′). The Cm gene was amplified with the primers H-CmN (5′-ggaagcttggcaatagttacccttattatc-3′) and B-CmC (5′-atggggatccatcgattataggtatgtgg-3′), using pCA3FL as a template. Overlap extension PCR was used to connect these three amplified fragments as templates using the yqgA-12 and yqgA-6 primers. This yqgA (∆YQ) fragment was cloned into the T-vector (pT7Blue) to obtain pT7-yqgA.
Western blotting
Cell numbers equivalent to an OD600 of 0.15 were withdrawn from 168-YQ3FL and WEC-YQ3FL cultures every hour. After centrifugation, the pellet was resuspended in sodium dodecyl sulfate-polyacrylamide gel electrophoresis (SDS-PAGE) sample buffer, and the sample proteins were applied to a 15% SDS-PAGE gel.Citation17) Western blotting was performed as previously described.Citation11)
Immunofluorescent microscopy
Cell numbers corresponding to an OD600 of 0.5 were collected at each time point and were prepared for Immunofluorescent microscopy (IFM) as previously described.Citation7) Differential interference microscopy and fluorescence microscopy were performed with a Zeiss AxioImager M1, 63×Apochromat objective, and a standard rhodamine filter set for visualizing Cy3. Exposure times were 10 ms for differential interference microscopy and 50 ms for Cy3.
Results
YqgA expression levels
YqgA is an abundant cell wall-binding protein composed of 142 amino acids, including an N-terminal signal sequence for secretion. The calculated Mr of the mature protein is 12,251, and the predicted pI is 10.1. Proteins homologous to YqgA are found in only a subset of Bacillus strains: B. subtilis, B. amyloliquefaciens, B. cereus, B. pumilus, B. thuringiensis, and B. lichemiformis, and there is no information about any functional domains or motifs from the amino acid sequence at present. At first, we investigated the expression level of YqgA during culturing (Fig. ). To detect YqgA protein by Western blotting, we constructed a YqgA fusion protein with a 3×FLAG epitope tag at the C-terminus (YqgA-3×FLAG) driven by the endogenous yqgA promoter. In the 168-YQ3FL strain, which has a wild-type background, the amount of expressed YqgA-3×FLAG fusion protein increased immediately after the cells entered the exponential phase, and then decreased after the cells entered the stationary phase (Fig. (A)). In contrast, WEC is a strain that is deficient in the major extracellular proteases (WprA and Epr), and the amount of YqgA-3×FLAG expressed on a WEC background at stationary phase was apparently greater than that expressed on a wild-type background (Fig. (B)). These results suggest that YqgA is digested by the extracellular proteases in the early stationary phase. Alternatively, a protein binding to YqgA and anchoring to cell wall may be digested by the proteases.
Fig. 1. Timecourse of YqgA expression.
Notes: YqgA-3×FLAG in 168-YQ3FL with a wild-type background (A) and in WEC-YQ3FL with a WEC background (B) was detected by Western blot analysis. Cells were harvested at the times indicated. YqgA in 168-YQ3FL was mainly detected during the exponential phase. In contrast, YqgA in WEC-YQ3FL, which disrupts the major proteases WprA and Epr, exists in the early stationary phase in addition to the exponential phase.
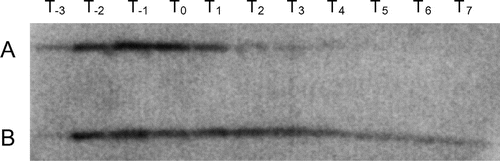
Subcellular localization of YqgA
Next, we investigated the subcellular localization of YqgA using the YqgA-3×FLAG fusion protein expressed in the 168-YQ3FL strain. We observed the localization of YqgA-3×FLAG at different time points and found that the protein was localized to the cell division site at time point T−0.5 of the culture (Fig. ). At the other time points, however, we could not observe any clear signals from the cells. To investigate whether YqgA localization is affected by the expression level of the protein or whether localization is dependent on the culture timing, we also observed subcellular localization of YqgA in WEC-background cells. As described above, a large amount of YqgA existed in early stationary phase in WEC-background cells, while it did not exist in the phase in wild-type-background cells (Fig. ). However, the localization of YqgA in WEC-background cells was also detected at T−0.5 similar to the wild-type-background cells. Although the amount of YqgA in the early stationary phase was similar to that detected in the exponential phase, we could not observe the localization in the early stationary phase. These results suggest that the localization of YqgA depends on the growth phase of the culture, and does not depend on the amount of expressed protein. YqgA may bind to a specific protein or cell wall structure existing at the cell division site in cells during the transition period between the exponential and stationary phases.
Fig. 2. YqgA localization in 168- and WEC-background cells.
Notes: Cells were cultured in LB medium and harvested at the times indicated. The images from differential interference microscopy (grey) and immunofluorescent microscopy (red) were merged. The black bar indicates 5 μm. YqgA-3×FLAG was detected at cell division sites only at T−0.5.
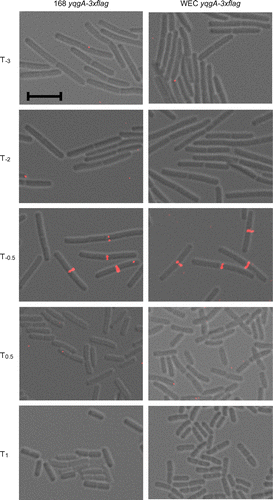
In Gram-positive bacteria, septum formation and cell separation does not carry out simultaneously. To investigate whether the YqgA localization depends upon septum formation or cell separation, we observed the localization in genetic backgrounds that are defective in septum formation and cell separation. We investigated the ability of YqgA to localize properly to the cell division site in FtsZ- or Pbp2B-depleted cells, which are defective in septum formation. However, we were unable to detect any YqgA-containing foci in cells of these genetic backgrounds (data not shown). Next, to investigate whether the YqgA localization at the cell division site is dependent on septum formation or cell separation, we used a strain defective in cell separation, while the cells form septa normally. In the EFS-YQ3FL strain used in this observation, lytE and cwlS were null, and lytF was inducible by IPTG. To separate the cells in the pre-culture, IPTG was added to the pre-culture for the induction of lytF, because depletion of these three cell separation enzymes produces a cell separation defect.Citation9) Then, aliquots of the pre-culture were used to inoculate fresh medium with or without IPTG, and the localization of YqgA-3×FLAG was observed (Fig. ). In the cells cultured without IPTG, YqgA-3×FLAG was not detected at the cell division site. This result indicates that the YqgA localization does not depend upon septum formation, because this strain forms septa without cell separation enzymes. In contrast, the cells cultured with IPTG displayed a morphology of four connected cells (Fig. ). Because these cells express only LytF out of the three cell separation enzymes, the cell separation in the cells may have been delayed compared to wild-type cells. In the cells cultured with IPTG, YqgA was detected at the center of the four connected cells. The center septum in the four connected cells is the septum formed before the first generation. In addition, no foci were detected at the new septa at the quarter positions of the four connected cells. This result demonstrates that YqgA localization depends on cell separation, and it is affected by the expression of cell separation enzymes. In Fig. , YqgA localization was observed at most of the division sites in the wild type and WEC-background cells. In contrast, the localization in the cells defective in cell separation was detected only at some of the division sites in spite of LytF induction (Fig. ). It appears that the time period for YqgA localization in cells defective in cell separation is shorter and delayed compared to the time period in wild type and WEC cells. Although cell separation proceeds slower than in wild-type cells in the separation-defective mutant, modifications of the cell wall (e.g. attaching teichoic acid) should be normal. Some type of modification to the cell wall may affect YqgA localization.
Fig. 3. Cellular localization of YqgA depends on cell separation enzymes.
Notes: EFS-YQ3FL cells were cultured in LB medium with or without 0.3 mM IPTG and harvested at T−0.5. Images from differential interference microscopy (grey) and immunofluorescent microscopy (red) were merged. Arrowheads indicate cell division sites. Black bar indicates 5 μm. YqgA-3×FLAG was detected at center of four connected cells expressing LytF.
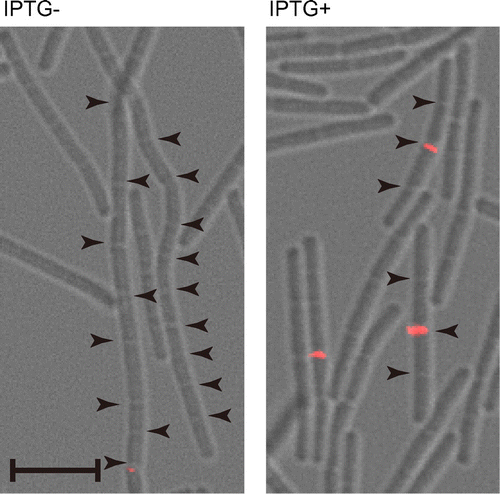
Mutations in YqgA with a cell separation-defective background affect cell growth
To investigate the function of YqgA and the relationship between YqgA and the DLEPases, we prepared two yqgA mutants and combined the mutations with the defect in cell separation enzymes. A truncated YqgA (YQGAd) was constructed by inserting pMUTIN4 into the endogenous chromosomal yqgA gene.Citation18) In the resulting protein, amino acids 103–142 at the C-terminal end of YqgA are replaced with an amino acid sequence of RIRGSPAC from the pMUTIN4 vector. Therefore, the majority of the YqgA sequence is present in the YQGAd mutation. Alternatively, ∆YQ was constructed by replacing the entire yqgA gene with the Cm-resistant gene. Only the first 22N-terminal amino acids of YqgA, which encode the signal peptide, remain in this mutant. We found that both yqgA mutants expressed in a wild-type background, 168-YQGAd and 168-∆YQ, grew similar to wild-type cells (Fig. ). However, the growth of the EF-YQGAd mutant, in which truncated yqgA is combined with ∆lytE and ∆lytF, was delayed compared to the growth of wild-type cells in early culture stage, whereas the growth rate of the EF-∆YQ mutant was not distinguishable from the growth rate of wild-type cells (Fig. ). These results suggest that the truncated mutant of YqgA (YQGAd) is a gain-of-function mutation that causes a growth delay and demonstrate that the yqgA mutation relates to the lytE and lytF mutations. Incidentally, the YQGAd mutation in combination with the single mutation of lytE or lytF did not produce the growth delay (data not shown).
Fig. 4. Growth curves of yqgA mutants.
Notes: Fresh LB medium was inoculated with overnight cultures to give an initial absorbance equivalent to OD600 = 0.001 and cultured at 37 °C with vigorous shaking. Solid lines represent the wild-type background and strains indicated with dashed lines are defective in the cell separation enzymes (∆lytE ∆lytF). Open circles are yqgA+, closed triangles are ∆YQ (a null mutant of yqgA), and × symbols are YQGAd (a truncated yqgA). Growth of EF-YQGAd with a lytE and lytF background showed a delay in cell growth.
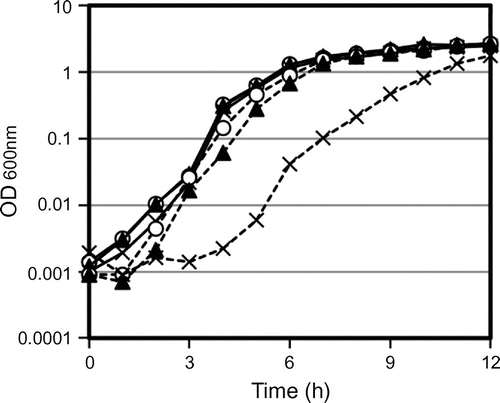
Fig. shows the cell morphology of strains EF, 168-∆YQ, EF-YQGAd, and EF-∆YQ at an OD600 = 0.01. The cell morphology of strain EF was of normal, wild type bacilli and that of 168-∆YQ was slightly twisted in places (Fig. (A) and (B)). In contrast, heavily twisted cells were often observed in strains harboring the truncated yqgA or ∆yqgA mutations on the lytE and lytF mutant background (Fig. (C) and (D)). Because these morphological changes were observed in both yqgA mutants, this phenotype was not caused by the gain-of-function mutation in the truncated yqgA mutation. Therefore, these results also demonstrate that yqgA has a genetic interaction with the genes encoding cell separation enzymes. The frequency of the twisted cells decreased after the observed time period (OD600 = 0.01), and cells from the overnight cultures of all the strains studied had usual shapes. Therefore, these morphological changes may occur in the very early culturing stage, and the twisted cells are titrated out during longer culture periods. These data imply that YqgA and the cell separation enzymes may play a role in the conversion of stationary-phase cells to exponential-phase cells in a very early culture stage.
Fig. 5. Cell morphology of yqgA mutants.
Notes: Cells were cultured same as in Fig. , and harvested at the period at OD600 = 0.01. Cell morphology was observed by differential interference microscopy. (A) EF, (B) 168-∆YQ, (C) EF-YQGAd, and (D) EF-∆YQ. The black bar indicates 5 μm. Cells with a truncated or null yqgA mutation on a lytE and lytF mutant background (EF-YQGAs and EF-∆YQ) often displayed a heavily twisted cell morphology.
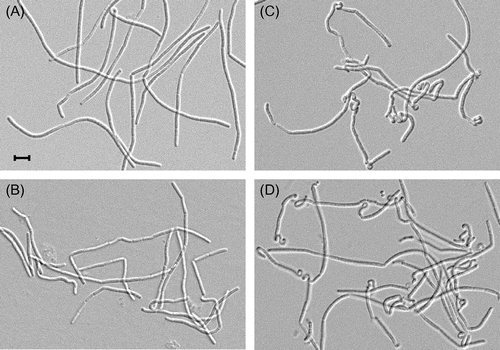
Discussion
In this study, we demonstrated that yqgA has genetic interactions with cell separation enzymes in some respects. The localization of YqgA at the cell division site was affected by defects in LytE, LytF, and CwlS, which are the cell separation enzymes (Fig. ). A gain-of-function mutation of truncated YqgA caused a delay in cell growth on the lytE and lytF mutant background (Fig. ). Furthermore, cells with either a null or truncated yqgA mutation in combination with the lytE and lytF mutations exhibited a twisted morphology (Fig. ).
YqgA was detected at cell division sites by IFM, but the foci were only observed at T−0.5. To the best of our knowledge, this is the first report to demonstrate that a bacterial protein forms foci during the transition period between the exponential and stationary growth phases. Before the transition period, the OD600 value increased rapidly, and the cell length was measurably longer than after the post-transition period (Fig. ). In other words, growth rate and cell length changed dramatically at the transition period. Therefore, the regulation of cell morphogenesis might be changed during the transition period. The cellular localization of YqgA was observed at the period, when the cell morphology started to change. Besides, morphological changes in yqgA mutant cells were observed in early culturing period (Fig. ). The twisted cells arose between OD600 = 0.001 and 0.01, because the cell morphology of all the strains used in this study looked normal in stationary phase. Since cell length in stationary phase is shorter than that in exponential phase, cell length changes in the early culture stage, and the twisted cells were observed in the period in yqgA mutants. Taken together, changes in phenotypes related to yqgA were observed in the periods when the cell morphology changed. These results imply that YqgA has a function related to changing cell morphology. Consistent with this, yqgA shows genetic interactions with genes encoding DLEPases involved in cell morphogenesis. In addition to them, large amount of YqgA was observed from transition phase to early stationary phase in wild-type-background strain (Fig. ). Cells lacking intact YqgA protein during the period show twisted cell morphology at early culturing stage in the next culture. Perhaps, YqgA is involved in some preparation for next morphological change, when the cell starts to grow again (e.g. modifying cell wall structure, changing DLEPase activity, and so on). Besides, the morphological change in the twisted cells by yqgA mutation may be suppressed by expression of LytE and LytF, because the heavily twisted cells were only observed in lytE lytF background strains. Growth delay at early culturing stage observed in EF-YQGAd strain expressing truncated YqgA is likely to be caused by defective cells in overcoming the morphological change. The truncated YqgA may interfere overcoming the twisted cell morphology. This hypothesis led us propose that YqgA affect cell-wall-degrading activity of the DLEPases. However, our in vitro experiments indicate that purified YqgA protein does not participate in the degradation of PG by the DLEPases under our experimental conditions (data not shown).
Homologues of YqgA were identified in the species B. subtilis (average homology = 100%), B. amiloliquefeciens (average homology = 32.5%), B. cereus (average homology = 28.7%), B. pumilus (average homology = 31.5%), B. thuringiensis (average homology = 35.5%), and B. lichemiformis (average homology = 30.5%). However, some species within the genus Bacillus do not possess a yqgA gene. Surprisingly, the genomic structures of the yqgA flanking regions are homologous between the Bacillus species in spite of the presence or absence of yqgA, and the sequence homology of them are at least more than 75%. This information leads us to propose that the region containing yqgA may be a type of a mobile element, but we have not identified any features similar to a mobile element near the yqgA gene. Interestingly, this narrow distribution of the yqgA gene in Bacillus is also observed for IseA, which inhibits the PG-degrading activity of the DLEPases.Citation7) They share similar evolutionary distribution but are not identical. We investigated whether purified YqgA affects the inhibitory activity of IseA in vitro; however, we found that YqgA does not affect this activity. In this study, we revealed that genetic interactions are observed between yqgA and genes encoding DLEPases, which are involved in cellular morphogenesis. In addition, the identified, various phenotypes were observed in transition periods from exponential phase to stationary phase, and from stationary phase to exponential phase, which are the time periods in which changes in cell length occur. These results lead us to propose that YqgA is involved in changing cell morphology in the transition periods. Further studies are necessary to understand the physiological functions of YqgA.
Supplemental material
The supplemental material for this paper is available at http://dx.doi.org/10.1080/09168451.2014.923294.
bbb-131011-File007_1_.pdf
Download PDF (4.1 MB)Acknowledgments
We thank Dr. H. Niki at the National Institute of Genetics for providing 168-YQGAd through the National Bio-Resource Project of the MEXT, Japan, and Dr. Ching-Hao Teng for critical reading of the text and for his comments.
Funding
This work was supported by Grants-in-Aid for Scientific Research (B) [grant number 19380047] and (A) [grant number 22248008] (JS), and the Program for Dissemination of Tenure-Track System funded by the Ministry of Education and Science, Japan (MH).
Notes
Abbreviations: PG, peptidoglycan; DLEPase, dl-endopeptidase
References
- Antelmann H, Yamamoto H, Sekiguchi J, Hecker M. Stabilization of cell wall proteins in Bacillus subtilis: a proteomic approach. Proteomics. 2002;2:591–602.
- Smith TJ, Blackman SA, Foster SJ. Autolysins of Bacillus subtilis: multiple enzymes with multiple functions. Microbiology. 2000;146:249–262.
- Reith J, Mayer C. Peptidoglycan turnover and recycling in Gram-positive bacteria. Appl. Microbiol. Biotechnol. 2011;92:1–11.
- Fukushima T, Yamamoto H, Atrih A, Foster SJ, Sekiguchi J. A polysaccharide deacetylase gene (pdaA) is required for germination and for production of muramic delta-lactam residues in the spore cortex of Bacillus subtilis. J. Bacteriol. 2002;184:6007–6015.
- Fukushima T, Tanabe T, Yamamoto H, Hosoya S, Sato T, Yoshikawa H, Sekiguchi J. Characterization of a polysaccharide deacetylase gene homologue (pdaB) on sporulation of Bacillus subtilis. J. Biochem. 2004;136:283–291.
- Kobayashi K, Sudiarta IP, Kodama T, Fukushima T, Ara K, Ozaki K, Sekiguchi J. Identification and characterization of a novel polysaccharide deacetylase C (PdaC) from Bacillus subtilis. J. Biol. Chem. 2012;287:9765–9776.
- Yamamoto H, Hashimoto M, Higashitsuji Y, Harada H, Hariyama N, Takahashi L, Iwashita T, Ooiwa S, Sekiguchi J. Post-translational control of vegetative cell separation enzymes through a direct interaction with specific inhibitor IseA in Bacillus subtilis. Mol. Microbiol. 2008;70:168–182.
- Yamamoto H, Kurosawa S, Sekiguchi J. Localization of the vegetative cell wall hydrolases LytC, LytE, and LytF on the Bacillus subtilis cell surface and stability of these enzymes to cell wall bound or extracellular proteases. J. Bacteriol. 2003;185:6666–6677.
- Fukushima T, Afkham A, Kurosawa S, Tanabe T, Yamamoto H, Sekiguchi J. A new d, l-endopeptidase gene product, YojL (renamed CwlS), plays a role in cell separation with LytE and LytF in Bacillus subtilis. J. Bacteriol. 2006;188:5541–5550.
- Bisicchia P, Noone D, Lioliou E, Howell A, Quigley S, Jensen T, Jarmer H, Devine KM. The essential YycFG two-component system controls cell wall metabolism in Bacillus subtilis. Mol. Microbiol. 2007;65:180–200.
- Hashimoto M, Ooiwa S, Sekiguchi J. Synthetic lethality of the lytE cwlO genotype in Bacillus subtilis is caused by lack of d,l-endopeptidase activity at the lateral cell wall. J. Bacteriol. 2012;194:796–803.
- Carballido-López R, Formstone A, Li Y, Ehrlich SD, Noirot P, Errington J. Actin homolog MreBH governs cell morphogenesis by localization of the cell wall hydrolase LytE. Dev. Cell. 2006;11:399–409.
- Kaan T, Homuth G, Mäder U, Bandow J, Schweder T. Genome-wide transcriptional profiling of the Bacillus subtilis cold-shock response. Microbiology. 2002;148:3441–3455.
- Saller MJ, Fusetti F, Driessen AM. Bacillus subtilis SpoIIIJ and YqjG function in membrane protein biogenesis. J. Bacteriol. 2009;191:6749–6757.
- Sambrook J, Fritch EF, Maniatis T. Molecular cloning: a laboratory manual. Cold Spring Harbor: Cold Spring Harbor Laboratory; 1989.
- Anagnostopoulos C, Spizizen J. Requirements for transformation in Bacillus subtilis. J. Bacteriol. 1961;81:741–746.
- Laemmli UK. Cleavage of structural proteins during assembly of head of bacteriophage T4. Nature. 1970;227:680–685.
- Kobayashi K, Ehrlich SD, Albertini A, Amati G, Andersen KK, Arnaud M, Asai K, Ashikaga S, Aymerich S, Bessieres P, Boland F, Brignell SC, Bron S, Bunai K, Chapuis J, Christiansen LC, Danchin A, Débarbouille M, Dervyn E, Deuerling E, Devine K, Devine SK, Dreesen O, Errington J, Fillinger S, Foster SJ, Fujita Y, Galizzi A, Gardan R, Eschevins C, Fukushima T, Haga K, Harwood CR, Hecker M, Hosoya D, Hullo MF, Kakeshita H, Karamata D, Kasahara Y, Kawamura F, Koga K, Koski P, Kuwana R, Imamura D, Ishimaru M, Ishikawa S, Ishio I, Le Coq D, Masson A, Mauël C, Meima R, Mellado RP, Moir A, Moriya S, Nagakawa E, Nanamiya H, Nakai S, Nygaard P, Ogura M, Ohanan T, O'Reilly M, O'Rourke M, Pragai Z, Pooley HM, Rapoport G, Rawlins JP, Rivas LA, Rivolta C, Sadaie A, Sadaie Y, Sarvas M, Sato T, Saxild HH, Scanlan E, Schumann W, Seegers JF, Sekiguchi J, Sekowska A, Séror SJ, Simon M, Stragier P, Studer R, Takamatsu H, Tanaka T, Takeuchi M, Thomaides HB, Vagner V, van Dijl JM, Watabe K, Wipat A, Yamamoto H, Yamamoto M, Yamamoto Y, Yamane K, Yata K, Yoshida K, Yoshikawa H, Zuber U, Ogasawara N. Essential Bacillus subtilis genes. Proc. Natl. Acad. Sci. USA. 2003;100:4678–4683.
- Yamamoto H, Miyake Y, Hisaoka M, Kurosawa SI, Sekiguchi J. The major and minor wall teichoic acids prevent the sidewall localization of vegetative dl-endopeptidase LytF in Bacillus subtilis. Mol. Microbiol. 2008;70:297–310.