Abstract
Steroid hormones are responsible for the coordinated regulation of many aspects of biological processes in multicellular organisms. Since the last century, many studies have identified and characterized steroidogenic enzymes in vertebrates, including mammals. However, much less is known about invertebrate steroidogenic enzymes. In the last 15 years, a number of steroidogenic enzymes and their functions have been characterized in ecdysozoan animals, especially in the fruit fly Drosophila melanogaster. In this review, we summarize the latest knowledge of enzymes crucial for synthesizing ecdysteroids, the principal insect steroid hormones. We also discuss the functional conservation and diversity of ecdysteroidogenic enzymes in other insects and even non-insect species, such as nematodes, vertebrates, and lower eukaryotes.
Graphical Abstract
Ecdysteroidogenic enzymes are essential for biosynthesizing the steroid hormone 20-hydroxyecdysone that triggers developmental transitions in insects.
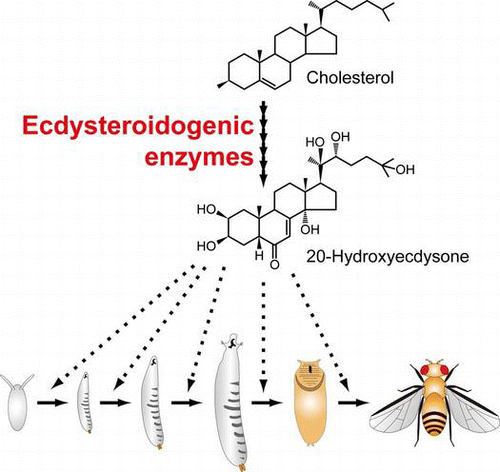
Steroid hormones are small and fat-soluble bioactive molecules that can pass through the cell membrane into the cytoplasm, where they activate signaling by binding to cytosolic or nuclear receptor proteins.Citation1) Steroid hormones play pivotal roles in the coordinated regulation of many developmental and physiological events in invertebrates as well as vertebrates. In particular, in the ecdysozoan animals, including insects and nematodes, steroid hormones are essential for controlling development, such as molting, metamorphosis, and diapause.Citation2,3) Steroid hormones are also involved in regulating biological processes in the adult stage of these animals, namely aging,Citation4,5) germline development,Citation6–9) innate immunity,Citation10) and neuronal functions controlling learning, memory, and behavior.Citation11–13) For the proper control of steroid hormone-dependent biological events, steroid hormones must be synthesized at the appropriate times throughout the life cycle. To understand the mechanisms of steroid hormone biosynthesis at the molecular level, many studies using insects and nematodes have been conducted. However, the steroidogenic enzymes in these organisms were not characterized in detail at the molecular level until recently.
In the last 15 years, a number of molecules essential for the regulation of insect steroid hormone biosynthesis, including steroidogenic enzymes, have been successfully identified and characterized. In the first half of this review, we intensively summarize the latest knowledge of the enzymes that biosynthesize the principal insect steroid hormones, called ecdysteroids, especially in a major model insect, the fruit fly Drosophila melanogaster. Notably, the identification and characterization of ecdysteroidogenic enzymes in D. melanogaster allow us to investigate how these enzymes are conserved and diversified within and beyond the class Insecta. Therefore, in the second half of this review, we discuss the conserved and diversified functions of ecdysteroidogenic enzymes in insects and beyond. We first describe ecdysteroidogenic enzymes and their counterparts in arthropods and nematodes. We particularly focus on steroidogenic enzymes identified from Caenorhabditis elegans, another valuable genetic model system to study invertebrate steroidogenesis. Finally, we discuss the functional conservation and diversity of steroidogenic enzymes beyond insects and nematodes, that is, in vertebrates and lower eukaryotes.
I. The ecdysteroid biosynthesis pathway in insects
Ecdysteroids have important functions in arthropod species, including insects.Citation14,15) Similar to the steroid hormone biosynthesis pathway in vertebrates, insects also utilize cholesterol and/or plant sterols as precursors of ecdysteroids.Citation16,17) However, it should be noted that insects, like nematodes, cannot synthesize sterol compounds de novo, as most ecdysozoan species lack genes encoding squalene synthase and other enzymes necessary for the sterol biosynthesis pathways that exist in vertebrates.Citation17,18) Thus, most ecdysozoan species must intake cholesterol and/or other sterols from their food for steroid hormone biosynthesis. Many phytophagous insects can convert plant sterols to cholesterol in their intestines.Citation19,20)
While ecdysteroids are biosynthesized from the embryonic to the adult stage,Citation12,21) ecdysteroid biosynthesis has been intensively studied using larvae and pupae. This is because the most typical ecdysteroids, ecdysone (E) and its derivative 20-hydroxyecdysone (20E), were originally identified as the “molting hormones” that can induce drastic morphological and developmental changes in the processes of molting and metamorphosis.Citation14,22) In the larval and pupal stages, E is synthesized in a special endocrine organ called the prothoracic gland (PG).Citation14) Once released into hemolymph, E is converted to 20E in the peripheral tissues, such as the fat body and midgut, but not in the PG.Citation14,23)
A current model of ecdysteroid biosynthesis is shown in Fig. . Ecdysteroid biosynthesis starts from the conversion of cholesterol to 7-dehydrocholesterol (7dC). Then, 7dC is converted to 3β,14α-dihydroxy-5β-cholest-7-en-6-one (5β-ketodiol) via multiple steps. So far, none of the precise intermediates between 7dC and 5β-ketodiol has been identified, partly because the intermediates are chemically unstable in the PG.Citation14,24) For this reason, the uncharacterized conversion steps are collectively called the “Black Box” (Fig. ).Citation25) Some studies have proposed that 3-oxo-steroids, such as cholesta-5,7-diene-3-one (3-oxo-7dC), cholesta-4,7-diene-3,6-dione-14α-ol (Δ4-diketol), and 5β[H]cholesta-7-ene-3,6-dione-14α-ol (diketol), might be the “Black Box” intermediate candidates in D. melanogaster.Citation25–27) In the terminal catalytic steps of ecdysteroid biosynthesis, 5β-ketodiol is then sequentially hydroxylated at carbon 25, carbon 22, carbon 2, and lastly carbon 20, resulting in a conversion to the active steroid hormone 20E.Citation14)
II. Identified ecdysteroidogenic enzymes
Ecdysteroidogenic enzymes identified so far are illustrated in Fig. and Table . Among them, the enzymes whose functions have been validated both in vivo and in vitro are Neverland (Nvd),Citation28,29) CYP306A1/Phantom (Phm),Citation30,31) CYP302A1/Disembodied (Dib),Citation32,33) CYP315A1/Shadow (Sad),Citation33) and CYP314A1/Shade (Shd).Citation23) Nvd is the [2Fe-2S] Rieske oxygenase and mediates the 7,8,-dehydrogenation of cholesterol to 7dC, the first step of the ecdysteroid biosynthesis pathway.Citation28,29) In fact, the cholesterol 7,8-dehydrogenation in insects had long been thought to be mediated by a cytochrome P450 monooxygenase based on experiments using pharmacological inhibitors.Citation34) Although this possibility has not been completely ruled out, the current data reliably support the idea that Nvd itself is the true identity of the cholesterol 7,8-dehydrogenase.Citation29)
Table 1. Enzymes for ecdysteroid biosynthesis.
In contrast to the Nvd-mediated cholesterol conversion step, the terminal hydroxylation steps of ecdysteroid biosynthesis, in which 5β-ketodiol is converted to 20E, are catalyzed by a series of cytochrome P450 monooxygenases, that is, Phm, Dib, Sad, and Shd.Citation23,30,31,33,35) In accordance with the involvement of P450s, ecdysteroid biosynthesis in D. melanogaster requires a gene called dare, encoding the adrenodoxin reductase.Citation36) This protein plays an essential role in reducing an electron carrier protein called adrenodoxin, which transfers the reducing equivalents to mitochondrial P450s and modifies their enzymatic activity.
In contrast to the validation of these five enzymes, ecdysteroidogenic enzymes involved in the “Black Box” have not yet been biochemically characterized because precise conversion steps in the “Black Box” still remain unclear. To date, 4 enzymes, CYP307A1/Spook (Spo),Citation37,38) CYP307A2/Spookier (Spok),Citation38) CYP6T3,Citation39) and Non-molting glossy/Shroud (Nm-g/Sro),Citation40) are hypothesized to act in the “Black Box.” Spo, Spok, and CYP6T3 are cytochrome P450s. On the other hand, Sro of D. melanogaster and Nm-g of the silkworm Bombyx mori are orthologous proteins and belong to a family of short-chain dehydrogenases/reductases (SDRs), some of which are also steroidogenic enzymes in vertebrates.Citation41) Transgenic RNAi animals of spok, sro, and Cyp6t3 cause developmental arrest at the larval stage in D. melanogaster, and the lethality of these RNAi animals is rescued by an oral administration of 5β-ketodiol but not of 7dC.Citation38–40) The same situation is observed in B. mori nm-g mutant.Citation40) These data suggest that loss-of-function mutant animals of these enzymes seem to be normal for the conversion from 5β-ketodiol to further downstream ecdysteroids but have a specific defect in the conversion step(s) between 7dC and 5β-ketodiol, which is the “Black Box.” Like Spok, the paralogous enzyme Spo is also likely to act as the “Black Box” enzyme because spo and spok are duplicated genes in Drosophilidae genomes.Citation38,42) spo and spok are involved in ecdysteroid biosynthesis during embryonic and post-embryonic development, respectively.Citation37,38)
Currently, we do not know how many enzymatic reactions are required for the synthesis of 5β-ketodiol from 7dC; thus, unidentified and uncharacterized enzymes that act in the “Black Box” may exist. Microarray analyses have reported that at least four other P450 genes, including Cyp4g1, Cyp6a17, Cyp6w1, and Cyp9c1, seem to be expressed in the D. melanogaster PG,Citation39,43) but their functions remain to be determined. In addition, our preliminary data also suggest that another non-P450-type enzyme has a crucial function in ecdysteroid biosynthesis in the PG in D. melanogaster and B. mori (Sora Enya and R.N., unpublished results).
III. Developmental phenotypes of mutants of ecdysteroidogenic enzyme genes
The first gene that was identified as a strong candidate to encode the ecdysteroidogenic enzyme was dib.Citation32) dib is predominantly expressed in the PG as well as other ecdysteroidogenic tissues, such as the ovary. A complete loss of dib function in D. melanogaster causes a significant reduction of embryonic ecdysteroid titer and signaling, resulting in embryonic lethality. dib mutants fail to progress in embryogenesis before the differentiation of the cuticle structure. Thus, dib mutants exhibit naked and polished cuticles. Importantly, the same phenotypes have been found in several mutants other than dib, including phm, sad, shd, spo, and sro.Citation32) These mutants were isolated from the classical saturation screen identifying D. melanogaster zygotic embryonic lethal mutations conducted by the Nobel laureates Christiane Nüsslein-Volhard and Eric Wieschaus.Citation44–46) They named the naked cuticle mutants, that is, characterless mutants, “disembodied,” “phantom,” “shadow,” “shade,” and “spook,” all of which refer to ghosts and specters. Based on this naming scheme, Lawrence I. Gilbert and Michael B. O’Connor, who contributed to identifying and enzymatically characterizing Dib, put forward the witty proposal that D. melanogaster embryonic lethal mutants showing the naked cuticle phenotype and the defects related to ecdysteroid biosynthesis be designated and classified as “Halloween mutants,” and their responsible genes are named “Halloween genes.”Citation47)
Another three ecdysteroidogenic enzyme genes, nvd, spok, and Cyp6t3, have not been identified as genetic mutants showing Halloween mutant-like phenotypes. Although nvd, spok, and Cyp6t3 are essential in larval development, it remains unclear whether these three genes are also crucial for embryonic ecdysteroid biosynthesis (Table ). In fact, nvd and spok appear not to be expressed in mid-embryogenesis when the embryonic ecdysteroid titer is high.Citation28,38) Therefore, it is likely that neither nvd nor spok causes the Halloween-type embryonic phenotype. To confirm this expectation, the isolation of complete loss-of-function alleles of these genes will be necessary.
Interestingly, despite the embryonic lethality of the fruit fly sro null mutant, the silkworm nm-g zygotic mutant worms exhibit no embryonic lethal phenotype but larval arrest phenotype, although the nm-g mutant is considered a complete loss-of-function allele of the clear ortholog of sro.Citation40) This outcome may occur because B. mori eggs store a large amount of ecdysteroid phosphates that can be utilized to produce embryonic ecdysteroids by dephosphorylation.Citation48) These data imply that, even if the zygotic function of an ortholog of a Halloween gene is abolished, embryonic lethality would not be caused in some insect species. In addition to nm-g, 9 other “non-molting (nm)” mutants of B. mori displaying larval arrest phenotypes have been isolated and maintained in the National BioResource Project of Japan (http://www.shigen.nig.ac.jp/silkwormbase/allele.jsp). It is possible that some of these strains are mutants of the Halloween genes, whereas the genes responsible for these nm mutants other than nm-g have not been identified.
IV. Conservation and diversity of ecdysteroidogenic enzyme genes in other insects and non-insect arthropods
As we wrote in the Introduction, the discovery of insect ecdysteroidogenic enzymes not only provides a better understanding of endocrinological regulation of insect development but also enables us to examine whether similar enzymes are encoded in the genomes of non-insect species and to compare the steroid hormone biosynthesis pathways across animal phyla.Citation49) From here, we will discuss conserved and diversified functions of ecdysteroidogenic enzymes in insects and beyond. To assist the reader, the phylogenetic relationship of the organisms described below is schematically represented in Fig. .
Fig. 2. Rough phylogenetic relationships of animals and unicellular ciliates that are described in this review. Popular names and genus names of these organisms are shown in underline and italics, respectively.
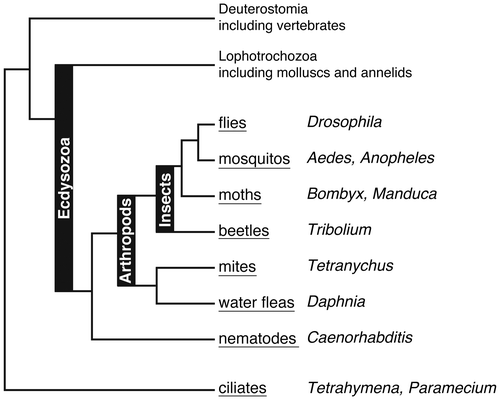
Ecdysteroids play pivotal roles not only in insects but also in other arthropods. Consistent with the fact, many ecdysteroidogenic enzyme genes are well conserved in the genomes of insects, as well as the water flea Daphnia pulexCitation50) and the spider mite Tetranychus urticae.Citation51) In contrast, curiously, some enzymes have been eliminated, duplicated, or substantially changed during arthropod evolution. For example, T. urticae lacks phm and thus lacks the ability to perform carbon-25 hydroxylation of ecdysteroids.Citation51) Consequently, the main ecdysteroid is ponasterone A instead of 20E in this species. In the red flour beetle Tribolium castaneum, we cannot find an ortholog of nvd by a regular BLAST search using available database of its genome or expression sequence tagsCitation52) (R.N., unpublished observation). This is quite paradoxical, as it has been reported that T. castaneum requires sterols for larval development.Citation53,54) More surprisingly, spok and Cyp6t3 are found only in Drosophilidae species,Citation38,39) suggesting that some ecdysteroidogenic enzymes have evolved and are maintained in very limited clades. Moreover, a transcriptional regulatory mechanism for the Drosophilidae-specific ecdysteroidogenic genes might also have co-evolved, as the molting defective gene, which encodes a Zn-finger protein found only in Drosophilidae species,Citation55) is essential for spok expression.Citation38) These data raise the interesting possibility that specific ecdysteroidogenic enzymes might also exist in specific insect species outside Drosophilidae.
The CYP307 family of cytochrome P450 proteins, including spo and spok, somehow became duplicated in the genomes of many insect species.Citation50) In addition to spo and spok, the third paralogous spo/spok-like gene, Cyp307b1/spookiest (spot), is found in some Coleopteran, Hymenoptera, and Dipteran species other than Drosophilidae. A molecular phylogenetic analysis suggests that the spo/spok (Cyp307a) family is distinctly separated from the spot genes, and the spot family appears to have originated from an early duplication following the split of Hymenoptera from Lepidoptera and Dipteran species.Citation56) spot genes are not found in Lepidopteran and Drosophilidae species, but they are present in the mosquitos Anopheles gambiae and Aedes aegypti.Citation38,56) Thus, spot has also been lost in some insect lineages. More complicatedly, even in the Drosophila radiation, there appears to have been gene duplication and loss of Cyp307a genes, and some Drosophilidae species carry another class of Cyp307a family gene Cyp307a3 in their genomes.Citation42) The in vivo functions of spot and Cyp307a3 have not yet been fully examined. It is hypothesized that spot is involved in ecdysteroid biosynthesis in the adult male tubular accessory gland of T. castaneum.Citation52) In Drosophila virilis, the temporal expression pattern of the Cyp307a genes is differentially regulated compared to that of D. melanogaster, which might reflect some sub-functionalization of Cyp307a genes during evolution.Citation42)
In addition to the evolution of ecdysteroidogenic enzyme genes at the genomic and gene-expression levels, our recent study implies that the enzymatic activity and/or substrate specificity of these enzymes has evolved as well. Most insect species appear to utilize Nvd for the 7,8-dehydrogenation of cholesterol. In contrast, Nvd of the cactophilic fruit fly Drosophila pachea has lost the 7,8-dehydrogenase activity on cholesterol but shows a 5,6-dehydrogenase enzymatic activity to produce 7dC from lathosterol, which is an unusual sterol but is enriched in the cactus.Citation57) Indeed, only three critical amino acid substitutions are needed to abrogate the 7,8-dehydrogenase activity of D. pachea Nvd.Citation57) These mutations increase animal survival fitness on lathosterol and are in a genomic region that faced recent positive selection. These data suggest that a substrate specificity of Nvd protein could be evolved and diversified among insect species depending on which sterols are utilized from food.
V. Conservation and diversity of steroidogenic enzymes in nematodes
The recent dramatic progress on the principal nematode bile acid-like steroid hormones, called dafachronic acids (DAs; Fig. ), has also made a great contribution toward a comprehensive view of steroid hormone biosynthesis, especially in invertebrates. DAs and their signaling regulate the decision to undergo reproductive development or arrest at the dauer diapause, an alternative developmental stage characterized by stress resistance.Citation58–60) DAs are also involved in the regulation of adult longevity in response to signals from the gonad.Citation4,5,59–61) The chemical structure of DAs was first reported in 2006,Citation62) after which a further unbiased comparative metabolomics approach succeeded in identifying the endogenous DAs in the soil nematode C. elegans.Citation63) Currently, Δ7-DA and Δ1,7-DA (Fig. ) are thought to be bioactive steroid hormones that act as potent ligands of DAF-12, the nuclear receptor for DAs.Citation63) Δ4-DA (Fig. ) was also previously identified as the DAF-12 ligand,Citation62) but it has recently been suggested that Δ4-DA may not play a significant role in vivo.Citation63) It should be noted that several other steroidal molecules are endogenously detected in C. elegansCitation63) and, thus, that some of them might also play significant roles in regulating nematode development and physiology.
Fig. 3. The dafachronic acid biosynthesis pathway and responsible biosynthetic enzymes in the nematode C. elegans.
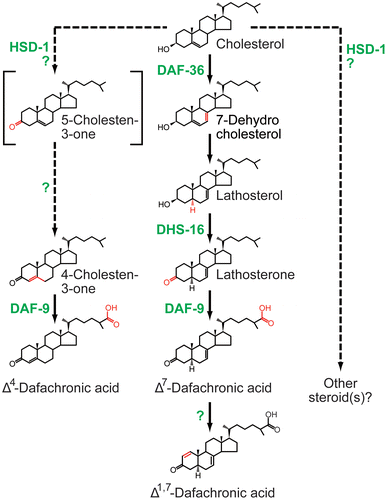
In the last decade, a number of the steroidogenic enzymes for DAs have also been identified and characterized in vivo and in vitro. Like ecdysteroids in insects, DAs are derived from dietary cholesterol.Citation64) Cholesterol is then converted to DAs via several intermediates in multiple pathways (Fig. ). As illustrated in Fig. and Table , biochemically validated enzymes for DA biosynthesis identified so far are the Rieske oxygenase DAF-36,Citation29,65–67) the cytochrome P450 monooxygenase DAF-9,Citation62,66,68) and the SDR protein DHS-16.Citation69) Genetic evidence also suggests that a putative 3β-hydroxysteroid dehydrogenase HSD-1Citation70) and a putative P450 oxidoreductase EMB-8Citation69) are involved in DA biosynthesis, whereas their functions have not been yet biochemically elucidated.
Table 2. Enzymes for dafachronic acid biosynthesis.
Considering an evolutionary commonality of steroidogenic enzymes, we can summarize that DAs are biosynthesized by proteins belonging to the Rieske oxygenase, SDR, and cytochrome P450 families, all of which are involved in ecdysteroid biosynthesis as well. More precisely, daf-36 is a truly functional ortholog of insect nvd, as C. elegans daf-36 can be substituted with nvd function in vivo in D. melanogaster.Citation29) However, molecular phylogenetic analyses suggest that neither daf-9 nor dhs-16 is orthologous to any of insect P450 or SDR genes, respectively.Citation40,49) Thus, between the biosynthesis of ecdysteroids and DAs, a molecular mechanism of cholesterol metabolism, that is, the first step of the steroidogenic pathway, is highly conserved during ecdysozoan evolution. Consistent with this feature, a family of Niemann–Pick disease type C proteins, which regulate intracellular cholesterol trafficking in the steroidogenic tissues, are also essential for the biosynthesis of both ecdysteroids and DAs.Citation71–74) In contrast, the downstream conversion steps and the responsible enzymes may have diverged and evolved independently.Citation49)
In nematodes, in addition to dauer formation and aging, the molting process also depends on sterols.Citation75) However, at least in C. elegans, DAs are not clearly involved in molting, and no steroidal “molting hormone” has been identified. In addition, no chemicals similar/analogous to ecdysteroids and no orthologous genes encoding insect ecdysteroidogenic enzymes, except daf-36/nvd, have been identified in any free-living nematodes.Citation75–77) Nevertheless, some nematodes (but not C. elegans) possess functional orthologs of genes encoding the ecdysone receptor components EcR and ultraspiracle (usp).Citation78) To further understand steroid hormone-dependent regulatory mechanisms of molting in nematodes, it would be intriguing to examine what ligands can activate such nematode EcR/USP proteins.
VI. Conservation and diversity of steroidogenic enzymes in other animal phyla
Overall, the major active steroid hormones are different between ecdysozoans and vertebrates in their structures, especially the presence and absence of the long side-chain derived from sterols, respectively. In vertebrate steroid hormone biosynthesis, cholesterol is commonly converted to pregnenolone by side-chain cleavage catalyzed by the cytochrome P450 monooxygenase P450scc/CYP11A1.Citation79) Indeed, P450scc/CYP11A1 mediates the key rate-limiting step in the biosynthesis of all vertebrate steroids.Citation79) In contrast, in the biosynthesis pathways of ecdysteroids and DAs, the active steroid hormones retain the sterol-derived side-chains. This large structural difference may reflect an independent elaboration of almost all steroidogenic enzymes between ecdysozoans and vertebrates.Citation49,80)
A notable exception is daf-36/nvd, which is evolutionarily conserved not only in ecdysozoans but also in deuterostome species, including the sea urchin, sea squirt, fishes, and amphibians.Citation28,29,65) These deuterostome DAF-36/Nvd are enzymatically functional and exhibit cholesterol 7,8-dehydrogenase activity.Citation29) Thus, in addition to the side-chain cleavage of cholesterol, the conversion from cholesterol to 7dC may occur physiologically in deuterostome animals, including vertebrates. At the same time, it should be emphasized that no orthologs of daf-36/nvd are found in mammalian genomes.Citation28,29,65) In the future, detailed functional analyses of deuterostome daf-36/nvd would be crucial to understanding the in vivo roles of orthologs of daf-36/nvd in these deuterostomes and an adaptive reason why mammals lost daf-36/nvd.
Moreover, the striking evolutionarily conservation of daf-36/nvd is indicated in more basal, primitive organisms, including the sea anemone Nematostella vectensisCitation29) and even the unicellular ciliates Tetrahymena thermophila, other Tetrahymena species, and Paramecium tetraurelia.Citation81) Current molecular phylogenetic evidence suggests that a daf-36/nvd ortholog was horizontally transferred, most likely from a unicellular opisthokont to a common ancestor of these ciliates.Citation81) The T. thermophile ortholog of daf-36/nvd, designated DES7, encodes an active sterol 7,8-dehydrogenase, as the 7,8-dehydrogenation is completely abolished in DES7 knock-out mutant cells, whereas there appear to be no differences in growth rates or cell shape between wild-type and DES7 knock-out mutant strains.Citation81) An interesting question to ask is whether this unicellular daf-36/nvd is involved in the biosynthesis and/or metabolism of steroids. Related to this point, investigating the evolutionary origin of daf-36/nvd would be intriguing for a comprehensive understanding of the roles of steroid hormones across eukaryotes.
VII. Future directions
As described above, tremendous advances in identification and characterization of enzymes responsible for ecdysteroid biosynthesis as well as DA biosynthesis have improved our understanding of the development and physiology of ecdysozoan animals. Now, many researchers are trying to unravel the higher regulatory mechanisms that control steroid hormone biosynthesis adaptively and coordinately throughout the life cycles of many organisms. With regard to hormonal and transcriptional regulatory mechanisms and their biological significance in ecdysteroid biosynthesis, we strongly recommend reading the excellent review articles that have recently been published.Citation82–93) Here, we would like to note just one of the important questions to be answered: Which enzyme serves as the rate-liming enzyme in the ecdysteroid biosynthesis pathway? Classical studies have proposed that the major trophic neuropeptide, known as the prothoracicotropic hormone (PTTH), appears to catalyze the rate-limiting step, which might be a step in the “Black Box.”Citation14) According to this hypothesis, any of the “Black Box” enzymes, including Spo, Spok, Nm-g/Sro, or CYP6T3, could act as the rate-limiting enzyme. PTTH is necessary and/or sufficient to modulate the expression of ecdysteroidogenic enzyme genes such as spo, spok, and sro in the PG of D. melanogaster and B. mori.Citation35,37,40,94,95) On the other hand, overexpression of most ecdysteroidogenic genes is not sufficient for accelerating development,Citation28,30,37–40) suggesting that the proper transcriptional regulation of an enzyme may not be critical for its action in the rate-liming step. Thus, an important future direction is to investigate whether the activity of any of these enzymes is regulated at the protein level. It should be noted that Spo is one of ecdysteroidogenic enzymes that are phosphorylated in the PG of the tobacco hornworm Manduca sexta,Citation96) implying post-translational control of Spo activity.
Identification of ecdysteroidogenic enzymes in plants is also important. A number of ecdysteroids have been isolated from not only animals but also many plants.Citation97,98) Several studies have suggested that plant ecdysteroids, so-called phytoecdysteroids, may participate in the defense of plants against phytophagous invertebrates.Citation97) Phytoecdysteroids indeed include E and 20E, and at least some catalytic steps are mediated by cytochrome P450s.Citation99) However, no plant orthologs of ecdysteroidogenic enzyme genes are found in published genomes. Therefore, it is expected that phytoecdysteroids are biosynthesized by a largely different class of enzymes.
From the aspect of applied biology, knowledge of ecdysteroidogenic enzymes and DA biosynthesis enzymes may also contribute to developing new effective pesticides for insects and nematodes. Ecdysteroid agonists that specifically bind to EcR have already been used as commercial insecticides, such as diacylhydrazine congeners.Citation100,101) Like EcR, it is conceivable that enzymes for synthesizing ecdysteroids and DAs could be feasible targets for discovering and developing chemical compounds that act as insecticides. These chemical compounds affecting steroidogenic enzyme activities are expected to be harmless to vertebrates, including humans, because most ecdysteroidogenic enzymes and DA biosynthesis enzymes are structurally dissimilar to vertebrate steroidogenic enzymes, as described above. Indeed, a high-throughput chemical screen has succeeded in identifying a chemical called dafadine, which is a specific inhibitor against C. elegans DAF-9 and suppresses C. elegans growth.Citation102) Surveying and finding other chemical compounds inhibiting any ecdysteroidogenic enzymes or DA biosynthesis enzymes might also be novel, effective pesticides in the future.
Acknowledgments
We are deeply grateful to former and current members of the Niwa laboratory, as well as the laboratories of Dr Hiroshi Kataoka (The University of Tokyo) and Dr Tetsuro Shinoda (National Institute of Agribiological Sciences), for their tremendous contributions to our projects on the ecdysteroid biosynthesis pathway described here. We also thank Drs Naoki Takaya and Fuminori Tsuruta (University of Tsukuba) for helpful comments to improve the manuscript. Y.S.N. was a recipient of postdoctoral research fellowships from the Japan Society for the Promotion of Science.
Additional information
Funding
Notes
This review was written in response to the author’s receipt of the Japan Society for Bioscience, Biotechnology, and Agrochemistry Award for the Encouragement of Young Scientists in 2011.
References
- Rousseau GG. Fifty years ago: the quest for steroid hormone receptors. Mol. Cell. Endocrinol. 2013;375:10–13.10.1016/j.mce.2013.05.005
- Thummel CS. Molecular mechanisms of developmental timing in C. elegans and Drosophila. Dev. Cell. 2001;1:453–465.10.1016/S1534-5807(01)00060-0
- Fielenbach N, Antebi A. C. elegans dauer formation and the molecular basis of plasticity. Genes Dev. 2008;22:2149–2165.10.1101/gad.1701508
- Gáliková M, Klepsatel P, Senti G, Flatt T. Steroid hormone regulation of C. elegans and Drosophila aging and life history. Exp. Gerontol. 2011;46:141–147.10.1016/j.exger.2010.08.021
- Antebi A. Regulation of longevity by the reproductive system. Exp. Gerontol. 2013;48:596–602.10.1016/j.exger.2012.09.009
- Buszczak M, Freeman MR, Carlson JR, Bender M, Cooley L, Segraves WA. Ecdysone response genes govern egg chamber development during mid-oogenesis in Drosophila. Development. 1999;126:4581–4589.
- Ables ET, Drummond-Barbosa D. The steroid hormone ecdysone functions with intrinsic chromatin remodeling factors to control female germline stem cells in Drosophila. Cell Stem Cell. 2010;7:581–592.10.1016/j.stem.2010.10.001
- König A, Yatsenko AS, Weiss M, Shcherbata HR. Ecdysteroids affect Drosophila ovarian stem cell niche formation and early germline differentiation. EMBO J. 2011;30:1549–1562.10.1038/emboj.2011.73
- Domanitskaya E, Anllo L, Schüpbach T. Phantom, a cytochrome P450 enzyme essential for ecdysone biosynthesis, plays a critical role in the control of border cell migration in Drosophila. Dev. Biol. 2014;386:408–418.10.1016/j.ydbio.2013.12.013
- Rus F, Flatt T, Tong M, Aggarwal K, Okuda K, Kleino A, Yates E, Tatar M, Silverman N. Ecdysone triggered PGRP-LC expression controls Drosophila innate immunity. EMBO J. 2013;32:1626–1638.10.1038/emboj.2013.100
- Yamazaki Y, Kiuchi M, Takeuchi H, Kubo T. Ecdysteroid biosynthesis in workers of the European honeybee Apis mellifera L. Insect Biochem. Mol. Biol. 2010;41:283–293.
- Ishimoto H, Sakai T, Kitamoto T. Ecdysone signaling regulates the formation of long-term courtship memory in adult Drosophila melanogaster. Proc. Nat. Acad. Sci. USA. 2009;106:6381–6386.10.1073/pnas.0810213106
- Ishimoto H, Wang Z, Rao Y, Wu CF, Kitamoto T. A novel role for ecdysone in Drosophila conditioned behavior: linking GPCR-mediated non-canonical steroid action to cAMP signaling in the adult brain. PLoS Genet. 2013;9:e1003843.10.1371/journal.pgen.1003843
- Gilbert LI, Rybczynski R, Warren JT. Control and biochemical nature of the ecdysteroidogenic pathway. Annu. Rev. Entomol. 2002;47:883–916.10.1146/annurev.ento.47.091201.145302
- Spindler KD, Hönl C, Tremmel Ch, Braun S, Ruff H, Spindler-Barth M. Ecdysteroid hormone action. Cell Mol. Life Sci. 2009;66:3837–3850.10.1007/s00018-009-0112-5
- Carvalho M, Schwudke D, Sampaio JL, Palm W, Riezman I, Dey G, Gupta GD, Mayor S, Riezman H, Shevchenko A, Kurzchalia TV, Eaton S. Survival strategies of a sterol auxotroph. Development. 2010;137:3675–3685.10.1242/dev.044560
- Niwa R, Niwa YS. The fruit fly Drosophila melanogaster as a model system to study cholesterol metabolism and homeostasis. Cholesterol. 2011;2011: 176802.
- Kurzchalia TV, Ward S. Why do worms need cholesterol? Nat. Cell Biol. 2003;5:684–688.10.1038/ncb0803-684
- Ciufo LF, Murray PA, Thompson A, Rigden DJ, Rees HH. Characterisation of a desmosterol reductase involved in phytosterol dealkylation in the silkworm, Bombyx mori. PLoS ONE. 2010;6:e21316.
- Ikekawa N, Fujimoto Y, Ishiguro M. Reminiscences of research on the chemistry and biology of natural sterols in insects, plants and humans. Proc. Jpn. Acad. Ser. B. 2013;89:349–369.10.2183/pjab.89.349
- Sullivan AA, Thummel CS. Temporal profiles of nuclear receptor gene expression reveal coordinate transcriptional responses during Drosophila development. Mol. Endocrinol. 2003;17:2125–2137.10.1210/me.2002-0430
- Karlson P. On the hormonal control of insect metamorphosis. A historical review. Int. J. Dev. Biol. 1996;40:93–96.
- Petryk A, Warren JT, Marques G, Jarcho MP, Gilbert LI, Kahler J, Parvy J-P, Li Y, Dauphin-Villemant C, O’Connor MB. Shade is the Drosophila P450 enzyme that mediates the hydroxylation of ecdysone to the steroid insect molting hormone 20-hydroxyecdysone. Proc. Nat. Acad. Sci. USA. 2003;100:13773–13778.10.1073/pnas.2336088100
- Iga M, Kataoka H. Recent studies on insect hormone metabolic pathways mediated by cytochrome P450 enzymes. Biol. Pharm. Bull. 2012;35:838–843.10.1248/bpb.35.838
- Warren JT, O’Connor MB, Gilbert LI. Studies on the black box: incorporation of 3-oxo-7-dehydrocholesterol into ecdysteroids by Drosophila melanogaster and Manduca sexta. Insect Biochem. Mol. Biol. 2009;39:677–687.10.1016/j.ibmb.2009.08.004
- Blais C, Dauphin-Villemant C, Kovganko N, Girault JP, Descoins C, Lafont R. Evidence for the involvement of 3-oxo-delta 4 intermediates in ecdysteroid biosynthesis. Biochem. J. 1996;320:413–419.
- Ono H, Morita S, Asakura I, Nishida R. Conversion of 3-oxo steroids into ecdysteroids triggers molting and expression of 20E-inducible genes in Drosophila melanogaster. Biochem. Biophys. Res. Commun. 2012;421:561–566.10.1016/j.bbrc.2012.04.045
- Yoshiyama T, Namiki T, Mita K, Kataoka H, Niwa R. Neverland is an evolutionally conserved Rieske-domain protein that is essential for ecdysone synthesis and insect growth. Development. 2006;133:2565–2574.10.1242/dev.02428
- Yoshiyama-Yanagawa T, Enya S, Shimada-Niwa Y, Yaguchi S, Haramoto Y, Matsuya T, Shiomi K, Sasakura Y, Takahashi S, Asashima M, Kataoka H, Niwa R. The conserved Rieske oxygenase DAF-36/Neverland is a novel cholesterol-metabolizing enzyme. J. Biol. Chem. 2011;286:25756–25762.10.1074/jbc.M111.244384
- Niwa R, Matsuda T, Yoshiyama T, Namiki T, Mita K, Fujimoto Y, Kataoka H. CYP306A1, a cytochrome P450 enzyme, is essential for ecdysteroid biosynthesis in the prothoracic glands of Bombyx and Drosophila. J. Biol. Chem. 2004;279:35942–35949.10.1074/jbc.M404514200
- Warren JT, Petryk A, Marqués G, Parvy JP, Shinoda T, Itoyama K, Kobayashi J, Jarcho M, Li Y, O’Connor MB, Dauphin-Villemant C, Gilbert LI. Phantom encodes the 25-hydroxylase of Drosophila melanogaster and Bombyx mori: a P450 enzyme critical in ecdysone biosynthesis. Insect Biochem. Mol. Biol. 2004;34:991–1010.10.1016/j.ibmb.2004.06.009
- Chávez VM, Marques G, Delbecque JP, Kobayashi K, Hollingsworth M, Burr J, Natzle JE, O’Connor MB. The Drosophila disembodied gene controls late embryonic morphogenesis and codes for a cytochrome P450 enzyme that regulates embryonic ecdysone levels. Development. 2000;127:4115–4126.
- Warren JT, Petryk A, Marques G, Jarcho M., Parvy J-P, Dauphin-Villemant C, O’Connor MB. Molecular and biochemical characterization of two P450 enzymes in the ecdysteroidogenic pathway of Drosophila melanogaster. Proc. Nat. Acad. Sci. USA. 2002;99:11043–11048.10.1073/pnas.162375799
- Grieneisen ML, Warren JT, Gilbert LI. Early steps in ecdysteroid biosynthesis: evidence for the involvement of cytochrome P-450 enzymes. Insect Biochem. Mol. Biol. 1993;23:13–23.10.1016/0965-1748(93)90077-6
- Niwa R, Sakudoh T, Namiki T, Saida K, Fujimoto Y, Kataoka H. The ecdysteroidogenic P450 Cyp302a1/disembodied from the silkworm, Bombyx mori, is transcriptionally regulated by prothoracicotropic hormone. Insect Mol. Biol. 2005;14:563–571.10.1111/imb.2005.14.issue-5
- Freeman MR, Dobritsa A, Gaines P, Segraves WA, Carlson JR. The dare gene: steroid hormone production, olfactory behavior, and neural degeneration in Drosophila. Development. 1999;126:4591–4602.
- Namiki T, Niwa R, Sakudoh T, Shirai K-I, Takeuchi H, Kataoka H. Cytochrome P450 CYP307A1/Spook: a regulator for ecdysone synthesis in insects. Biochem. Biophys. Res. Commun. 2005;337:367–374.10.1016/j.bbrc.2005.09.043
- Ono H, Rewitz KF, Shinoda T, Itoyama K, Petryk A, Rybczynski R, Jarcho M, Warren JT, Marqués G, Shimell MJ, Gilbert LI, O’Connor MB. Spook and Spookier code for stage-specific components of the ecdysone biosynthetic pathway in Diptera. Dev. Biol. 2006;298:555–570.10.1016/j.ydbio.2006.07.023
- Ou Q, Magico A, King-Jones K. Nuclear receptor DHR4 controls the timing of steroid hormone pulses during Drosophila development. PLoS Biol. 2011;9:e1001160.10.1371/journal.pbio.1001160
- Niwa R, Namiki T, Ito K, Shimada-Niwa Y, Kiuchi M, Kawaoka S, Kayukawa T, Banno Y, Fujimoto Y, Shigenobu S, Kobayashi S, Shimada T, Katsuma S, Shinoda T. Non-molting glossy/shroud encodes a short-chain dehydrogenase/reductase that functions in the “Black Box” of the ecdysteroid biosynthesis pathway. Development. 2010;137:1991–1999.10.1242/dev.045641
- Wu X, Lukacik P, Kavanagh KL, Oppermann U. SDR-type human hydroxysteroid dehydrogenases involved in steroid hormone activation. Mol. Cell. Endocrinol. 2007;265–266:71–76.10.1016/j.mce.2006.12.006
- Sztal T, Chung H, Gramzow L, Daborn PJ, Batterham P, Robin C. Two independent duplications forming the Cyp307a genes in Drosophila. Insect Biochem. Mol. Biol. 2007;37:1044–1053.10.1016/j.ibmb.2007.05.017
- Niwa R, Sakudoh T, Matsuya T, Namiki T, Kasai S, Tomita T, Kataoka H. Expressions of the cytochrome P450 monooxygenase gene Cyp4g1 and its homolog in the prothoracic glands of the fruit fly Drosophila melanogaster (Diptera: Drosophilidae) and the silkworm Bombyx mori (Lepidoptera: Bombycidae). Appl. Entomol. Zool. 2011;46:533–543.10.1007/s13355-011-0074-6
- Nüsslein-Volhard C, Wieschaus E. Mutations affecting the pattern of the larval cuticle in Drosophila melanogaster I. Zygotic loci on the second chromosome. Roux’s Arch. Dev. Biol. 1984;193:267–282.
- Jürgens G, Wieschaus E, Nüsslein-Volhard C, Kluding H. Mutations affecting the pattern of the larval cuticle in Drosophila melanogaster II. Zygotic loci on the third chromosome. Roux’s Arch. Dev. Biol. 1984;193:283–295.
- Wieschaus E, Nüsslein-Volhard C, Jürgens G. Mutations affecting the pattern of the larval cuticle in Drosophila melanogaster III. Zygotic loci on the X-chromosome and fourth chromosome. Roux’s Arch. Dev. Biol. 1984;193:296–307.
- Gilbert LI. Halloween genes encode P450 enzymes that mediate steroid hormone biosynthesis in Drosophila melanogaster. Mol. Cell. Endocrinol. 2004;215:1–10.10.1016/j.mce.2003.11.003
- Ito Y, Yasuda A, Sonobe H. Synthesis and phosphorylation of ecdysteroids during ovarian development in the silkworm, Bombyx mori. Zool. Sci. 2008;25:721–727.
- Markov GV, Tavares R, Dauphin-Villemant C, Demeneix BA, Baker ME, Laudet V. Independent elaboration of steroid hormone signaling pathways in metazoans. Proc. Nat. Acad. Sci. USA. 2009;106:11913–11918.10.1073/pnas.0812138106
- Rewitz KF, Gilbert LI. Daphnia Halloween genes that encode cytochrome P450s mediating the synthesis of the arthropod molting hormone: evolutionary implications. BMC Evol. Biol. 2008;8:60.10.1186/1471-2148-8-60
- Grbi M, Van Leeuwen T, Clark RM, Rombauts S, Rouzé P, Grbi V, Osborne EJ, Dermauw W, Thi Ngoc PC, Ortego F, Hernández-Crespo P, Diaz I, Martinez M, Navajas M, Sucena E, Magalhães S, Nagy L, Pace RM, Djuranović S, Smagghe G, Iga M, Christiaens O, Veenstra JA, Ewer J, Villalobos RM, Hutter JL, Hudson SD, Velez M, Yi SV, Zeng J, Pires-daSilva A, Roch F, Cazaux M, Navarro M, Zhurov V, Acevedo G, Bjelica A, Fawcett JA, Bonnet E, Martens C, Baele G, Wissler L, Sanchez-Rodriguez A, Tirry L, Blais C, Demeestere K, Henz SR, Gregory TR, Mathieu J, Verdon LL, Farinelli L, Schmutz J, Lindquist E, Feyereisen R, Van de Peer Y. The genome of Tetranychus urticae reveals herbivorous pest adaptations. Nature. 2011;479:487–492.10.1038/nature10640
- Hentze JL, Moeller ME, Jørgensen AF, Bengtsson MS, Bordoy AM, Warren JT, Gilbert LI, Andersen O, Rewitz KF. Accessory gland as a site for prothoracicotropic hormone controlled ecdysone synthesis in adult male insects. PLoS ONE. 2013;8:e55131.10.1371/journal.pone.0055131
- Fraenkel G, Blewett M. The sterol requirements of several insects. Biochem. J. 1943;37:692–695.
- Fraenkel G, Blewett M. The basic food requirements of several insects. J. Exp. Biol. 1943;20:28–34.
- Neubueser D, Warren JT, Gilbert LI, Cohen SM. Molting defective is required for ecdysone biosynthesis. Dev. Biol. 2005;280:362–372.10.1016/j.ydbio.2005.01.023
- Rewitz KF, O’Connor MB, Gilbert LI. Molecular evolution of the insect Halloween family of cytochrome P450s: phylogeny, gene organization and functional conservation. Insect Biochem. Mol. Biol. 2007;37:741–753.10.1016/j.ibmb.2007.02.012
- Lang M, Murat S, Clark AG, Gouppil G, Blais C, Matzkin LM, Guittard E, Yoshiyama-Yanagawa T, Kataoka H, Niwa R, Lafont R, Dauphin-Villemant C, Orgogozo V. Mutations in the neverland gene turned Drosophila pachea into an obligate specialist species. Science. 2012;337:1658–1661.10.1126/science.1224829
- Sommer RJ, Ogawa A. Hormone signaling and phenotypic plasticity in nematode development and evolution. Curr. Biol. 2011;21:R758–R766.10.1016/j.cub.2011.06.034
- Wollam J, Antebi A. Sterol regulation of metabolism, homeostasis, and development. Annu. Rev. Biochem. 2011;80:885–916.10.1146/annurev-biochem-081308-165917
- Lee SS, Schroeder FC. Steroids as central regulators of organismal development and lifespan. PLoS Biol. 2012;10:e1001307.10.1371/journal.pbio.1001307
- Shen Y, Wollam J, Magner D, Karalay O, Antebi A. A steroid receptor-microRNA switch regulates life span in response to signals from the gonad. Science. 2012;338:1472–1476.10.1126/science.1228967
- Motola DL, Cummins CL, Rottiers V, Sharma KK, Li T, Li Y, Suino-Powell K, Xu HE, Auchus RJ, Antebi A, Mangelsdorf DJ. Identification of ligands for DAF-12 that govern dauer formation and reproduction in C. elegans. Cell. 2006;124:1209–1223.10.1016/j.cell.2006.01.037
- Mahanti P, Bose N, Bethke A, Judkins JC, Wollam J, Dumas KJ, Zimmerman AM, Campbell SL, Hu PJ, Antebi A, Schroeder FC. Comparative metabolomics reveals endogenous ligands of DAF-12, a nuclear hormone receptor, regulating C. elegans development and lifespan. Cell Metab. 2014;19:73–83.10.1016/j.cmet.2013.11.024
- Matyash V, Entchev EV, Mende F, Wilsch-Bräuninger M, Thiele C, Schmidt AW, Knölker H-J, Ward S, Kurzchalia TV. Sterol-derived hormone(s) controls entry into diapause in Caenorhabditis elegans by consecutive activation of DAF-12 and DAF-16. PLoS Biol. 2004;2:e280.10.1371/journal.pbio.0020280
- Rottiers V, Motola DL, Gerisch B, Cummins CL, Nishiwaki K, Mangelsdorf DJ, Antebi A. Hormonal control of C. elegans dauer formation and life span by a Rieske-like oxygenase. Dev. Cell. 2006;10:473–482.10.1016/j.devcel.2006.02.008
- Gerisch B, Rottiers V, Li D, Motola DL, Cummins CL, Lehrach H, Mangelsdorf DJ, Antebi A. A bile acid-like steroid modulates Caenorhabditis elegans lifespan through nuclear receptor signaling. Proc. Nat. Acad. Sci. USA. 2007;104:5014–5019.10.1073/pnas.0700847104
- Wollam J, Magomedova L, Magner DB, Shen Y, Rottiers V, Motola DL, Mangelsdorf DJ, Cummins CL, Antebi A. The Rieske oxygenase DAF-36 functions as a cholesterol 7-desaturase in steroidogenic pathways governing longevity. Aging Cell. 2011;10:879–884.10.1111/j.1474-9726.2011.00733.x
- Gerisch B, Weitzel C, Kober-Eisermann C, Rottiers V, Antebi A. A hormonal signaling pathway influencing C. elegans metabolism, reproductive development, and life span. Dev. Cell. 2011;1:841–851.
- Wollam J, Magner DB, Magomedova L, Rass E, Shen Y, Rottiers V, Habermann B, Cummins CL, Antebi A. A novel 3-hydroxysteroid dehydrogenase that regulates reproductive development and longevity. PLoS Biol. 2012;10:e1001305.10.1371/journal.pbio.1001305
- Patel DS, Fang LL, Svy DK, Ruvkun G, Li W. Genetic identification of HSD-1, a conserved steroidogenic enzyme that directs larval development in Caenorhabditis elegans. Development. 2008;135:2239–2249.10.1242/dev.016972
- Li J, Brown G, Ailion M, Lee S, Thomas JH. NCR-1 and NCR-2, the C. elegans homologs of the human Niemann-Pick type C1 disease protein, function upstream of DAF-9 in the dauer formation pathways. Development. 2004;131:5741–5752.10.1242/dev.01408
- Huang X, Suyama K, Buchanan J, Zhu AJ, Scott MP. A Drosophila model of the Niemann-Pick type C lysosome storage disease: dnpc1a is required for molting and sterol homeostasis. Development. 2005;132:5115–5124.10.1242/dev.02079
- Fluegel ML, Parker TJ, Pallanck LJ. Mutations of a Drosophila NPC1 gene confer sterol and ecdysone metabolic defects. Genetics. 2006;172:185–196.
- Huang X, Warren JT, Buchanan J, Gilbert LI, Scott MP. Drosophila Niemann-Pick Type C-2 genes control sterol homeostasis and steroid biosynthesis: a model of human neurodegenerative disease. Development. 2007;134:3733–3742.10.1242/dev.004572
- Entchev EV, Kurzchalia TV. Requirement of sterols in the life cycle of the nematode Caenorhabditis elegans. Semin. Cell Dev. Biol. 2005;16:175–182.10.1016/j.semcdb.2005.01.004
- Chitwood DJ. Biochemistry and function of nematode steroids. Crit. Rev. Biochem. Mol. Biol. 1999;34:273–284.10.1080/10409239991209309
- Frand AR, Russel S, Ruvkun G. Functional genomic analysis of C. elegans molting. PLoS Biol. 2005;3:e312.10.1371/journal.pbio.0030312
- Tzertzinis G, Egaña AL, Palli SR, Robinson-Rechavi M, Gissendanner CR, Liu C, Unnasch TR, Maina CV. Molecular evidence for a functional ecdysone signaling system in Brugia malayi. PLoS Negl. Trop. Dis. 2009;4:e625.
- Miller WL, Auchus RJ. The molecular biology, biochemistry, and physiology of human steroidogenesis and its disorders. Endocr. Rev. 2011;32:81–151.10.1210/er.2010-0013
- Feyereisen R. Arthropod CYPomes illustrate the tempo and mode in P450 evolution. Biochim. Biophys. Acta. 2011;1814:19–28.10.1016/j.bbapap.2010.06.012
- Najle SR, Nusblat AD, Nudel CB, Uttaro AD. The sterol-C7 desaturase from the ciliate Tetrahymena thermophila is a Rieske oxygenase which is highly conserved in animals. Mol. Biol. Evol. 2013;30:1630–1643.10.1093/molbev/mst076
- Tennessen JM, Thummel CS. Coordinating growth and maturation—insights from Drosophila. Curr. Biol. 2011;21:R750–R757.10.1016/j.cub.2011.06.033
- Rewitz KF, Yamanaka N, O’Connor MB. Timing is everything: PTTH mediated DHR4 nucleocytoplasmic trafficking sets the tempo of Drosophila steroid production. Front. Endocrinol. 2011;2:108.
- Tanaka Y. Recent topics on the regulatory mechanism of ecdysteroidogenesis by the prothoracic glands in insects. Front. Endocrinol. 2011;2:107.
- Yamanaka N, O’Connor MB. Nitric oxide directly regulates gene expression during Drosophila development: need some gas to drive into metamorphosis? Genes Dev. 2011;25:1459–1463.10.1101/gad.2080411
- Mirth CK, Shingleton AW. Integrating body and organ size in Drosophila: recent advances and outstanding problems. Front. Endocrinol. 2012;3:1–13.
- Hariharan IK. How growth abnormalities delay “puberty” in Drosophila. Sci. Signal. 2012;5:pe27.
- Yamanaka N, Rewitz KF, O’Connor MB. Ecdysone control of developmental transitions: lessons from Drosophila research. Annu. Rev. Entomol. 2013;58:497–516.10.1146/annurev-ento-120811-153608
- Rewitz KF, Yamanaka N, O’Connor MB. Developmental checkpoints and feedback circuits time insect maturation. Curr. Topics Dev. Biol. 2013;103:1–33.10.1016/B978-0-12-385979-2.00001-0
- Danielsen ET, Moeller ME, Rewitz KF. Nutrient signaling and developmental timing of maturation. Curr. Topics Dev. Biol. 2013;105:37–67.10.1016/B978-0-12-396968-2.00002-6
- Koyama T, Mendes CC, Mirth CK. Mechanisms regulating nutrition-dependent developmental plasticity through organ-specific effects in insects. Front. Physiol. 2013;4:263.
- Andersen DS, Colombani J, Léopold P. Coordination of organ growth: principles and outstanding questions from the world of insects. Trends Cell Biol. 2013;23:336–344.10.1016/j.tcb.2013.03.005
- Niwa YS, Niwa R. Neural control of steroid hormone biosynthesis during development in the fruit fly Drosophila melanogaster. Genes Genet. Syst. 2014;89:27–34.
- Yamanaka N, Honda N, Osato N, Niwa R, Mizoguchi A, Kataoka H. Differential regulation of ecdysteroidogenic P450 gene expression in the silkworm, Bombyx mori. Biosci. Biotechnol. Biochem. 2007;71:2808–2814.10.1271/bbb.70420
- McBrayer Z, Ono H, Shimell M, Parvy J-P, Beckstead RB, Warren JT, Thummel CS, Dauphin-Villemant C, Gilbert LI, O’Connor MB. Prothoracicotropic hormone regulates developmental timing and body size in Drosophila. Dev. Cell. 2007;13:857–871.10.1016/j.devcel.2007.11.003
- Rewitz KF, Larsen MR, Lobner-Olesen A, Rybczynski R, O’Connor MB, Gilbert LI. A phosphoproteomics approach to elucidate neuropeptide signal transduction controlling insect metamorphosis. Insect Biochem. Mol. Biol. 2009;39:475–483.10.1016/j.ibmb.2009.04.005
- Adler JH, Grebenok RJ. Occurrence, biosynthesis, and putative role of ecdysteroids in plants. Crit. Rev. Biochem. Mol. Biol. 1999;34:253–264.10.1080/10409239991209282
- Dinan L. Phytoecdysteroids: biological aspects. Phytochemistry. 2001;57:325–339.10.1016/S0031-9422(01)00078-4
- Canals D, Irurre-Santilari J, Casas J. The first cytochrome P450 in ferns. Evidence for its involvement in phytoecdysteroid biosynthesis in Polypodium vulgare. FEBS J. 2005;272:4817–4825.10.1111/ejb.2005.272.issue-18
- Palli SR, Hormann RE, Schlattner U, Lezzi M. Ecdysteroid receptors and their applications in agriculture and medicine. Vitam. Horm. 2005;73:59–100.10.1016/S0083-6729(05)73003-X
- Nakagawa Y. Nonsteroidal ecdysone agonists. Vitam. Horm. 2005;73:131–173.10.1016/S0083-6729(05)73005-3
- Luciani GM, Magomedova L, Puckrin R, Urbanus ML, Wallace IM, Giaever G, Nislow C, Cummins CL, Roy PJ. Dafadine inhibits DAF-9 to promote dauer formation and longevity of Caenorhabditis elegans. Nat. Chem. Biol. 2011;7:891–893.10.1038/nchembio.698