Abstract
Tulipalin B (α-methylene-β-hydroxy-γ-butyrolactone, PaB) is an antimicrobial natural product occurring in tulip (Tulipa gesneriana). PaB is directly formed from the precursor glucose ester 6-tuliposide B (PosB) by endogenous Pos-converting enzyme (TCE). Despite the potential usefulness of antibacterial PaB in various industrial applications, lack of facile synthetic schemes hampers its practical use. Herein, we describe an environmentally benign and facile process for the preparation of PaB using tulip biomass materials based on one-step enzyme reaction catalyzed by TCE without the use of petroleum-derived solvents. By screening 115 tulip cultivars, we found three elite cultivars, which accumulated PosB almost exclusively in flower tissues. The flower extracts with aqueous ethanol were partially purified with activated charcoal and subjected to the enzyme reaction with reusable immobilized TCE prepared from bulb crude extracts. The reaction was completed in a few hours at room temperature, and PaB was purified with activated charcoal and ethanol in a batch-wise manner.
Graphical Abstract
Tulipalin B preparation from tulip biomass by one-step reaction with reusable immobilized enzyme and purification with activated charcoal and ethanol.
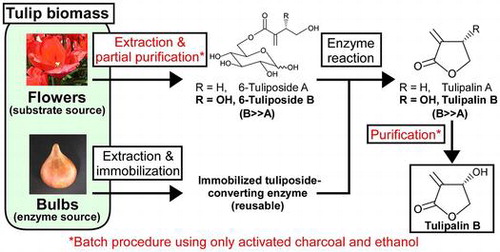
Compounds having an α-methylene-γ-butyrolactone moiety exhibit a variety of biological activities including antibacterial, antifungal, anti-inflammatory, allergic, phytotoxic, cytotoxic, and/or antitumor.Citation1,2) Natural products with this moiety are classified based on their chemical structures into three categories: sesquiterpene lactones, diterpenes, and non-terpenoid α-methylene-γ-lactones. Although they structurally have an exo- or endo-methylene group conjugated with a carbonyl group, the former, in most cases, is responsible for the biological activities of this class of compounds, in which the exo-methylene group functions as a Michael-type acceptor with biological nucleophiles such as l-cysteine, l-lysine, glutathione, and thiol-containing enzymes/proteins.
Tulipalin A (PaA) (Fig. ), the simplest α-methylene-γ-butyrolactone possible, was first isolated from Erythronium americanum as an antimicrobial substance,Citation3) and later from tulip (Tulipa gesneriana). It is known to be an antifungal substance against Fusarium oxysporum f. sp. tulipae, a causative fungus of tulip bulb rot diseaseCitation4,5) and as a causative agent of an allergic contact dermatitis called “tulip finger”.Citation6) Tulipalin B (PaB) (Fig. ), a β-hydroxy derivative of PaA, was also identified in tulip.Citation7–9) Although PaA and PaB show antimicrobial activities against a broad range of strains of bacteria and fungi, their properties are distinctly different: PaA functions as an antifungal agent, while PaB is an antibacterial.Citation4,5,7–15) PaA and PaB are formed in nature from the precursor sugar esters, 6-tuliposides A (PosA) and B (PosB), respectively (Fig. ). Pos are esters composed of d-glucose and α-methylene-γ-hydroxybutanoic acid and/or α-methylene-β,γ-dihydroxybutanoic acid, and are known as representative secondary metabolites in the tulip.Citation5,7,8,16,17) In tulip, large amounts of Pos accumulate in every tissue, although the amount and form of Pos vary depending on the tissue and developmental stage.Citation10,17) Pa, although far less abundant than Pos, are more biologically active and thus, Pos are considered to be the storage forms of active Pa. Although it has long been proposed that Pos conversion to Pa occurs non-enzymatically in planta because of the chemical lability of Pos, which are spontaneously converted to Pa under neutral to basic conditions in vitro,Citation8,10,18) we recently found that this conversion is catalyzed by the Pos-converting enzyme (TCE; EC. 4.2.99.-), a carboxylesterase-like enzyme that specifically catalyzes the quantitative lactone-forming intramolecular transesterification in plants (Fig. ).Citation19–21) PosA and PosB are converted to the corresponding Pa by PosA-converting enzyme (TCEA) and PosB-converting enzyme (TCEB), respectively. Genes for TCEA (EC 4.2.99.22) have been isolated from petals and bulbs,Citation20,21) and recently, we have identified the gene for TCEB, the details of which will be published elsewhere in due course.
Fig. 1. Enzymatic preparation of tulipalin B (PaB) based on the one-step direct conversion of 6-tuliposides (Pos) A and B to tulipalins (Pa) A and B by tuliposide-converting enzyme (TCE).
Note: The 1α- and 1β-anomers are present for PosA and PosB, and both anomers serve as substrates for TCE. Critical steps to this process are the use of tulip flowers that predominantly accumulate PosB as the substrate source, the use of tulip bulbs as a source of TCE, and the use of activated charcoal and ethanol for PaB purification.
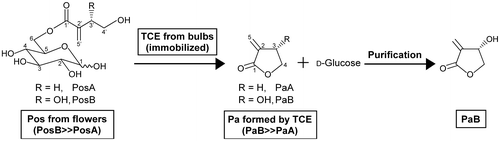
PaA and PaB are considered to be useful natural products with potential applications as synthetic intermediates in the production of several bioactive compounds,Citation22,23) antimutagenic agents,Citation24) insect repellents,Citation25) and as monomers of functional biobased polymers.Citation26) PaA is prepared by organic synthesis and is available from commercial sources. Since PaA is known as a causative agent of allergic contact dermatitis, its utilization may be limited to industrial raw materials, such as the monomer of bioplastics. In contrast, antibacterial PaB, which has been reported to be non-allergic,Citation16,27) despite some contrasting evidence from the literature on its allergic properties based on experiments with guinea pigs,Citation28,29) can potentially be used as a natural preservative agent, instead of a conventionally used synthetic parabens (a series of p-hydroxybenzoic acid esters) for pharmaceuticals, cosmetics, and toiletries. Therefore, the development of a facile preparation method of PaB will facilitate its practical use in those fields. Although many studies have demonstrated the synthesis of PaB,Citation30–34) its commercial supply has not yet been achieved because of the impracticality of the proposed methods for adaptation to industrial preparation. We previously reported a simple enzyme-mediated procedure using TCE coupled with organic solvent extraction.Citation35) In this process, PosA and PosB extracted from tulip flowers were treated with crude TCE from tulip tissues, and the resulting PaA and PaB were individually purified by successive selective extractions. Since tulip flowers are normally treated as agricultural waste in industrial bulb production and are cut immediately after flowering to prompt carbohydrate translocation from leaves to bulbs, utilizing the cut tulip flowers as a source of Pos for enzyme-mediated Pa production is a reasonable approach to maximize the use of the tulip biomass. Moreover, the use of tulip crude extracts as the TCE source, especially from bulbs, also contributes to the efficient utilization of tulip biomass, because a large number of unsalable irregular bulbs occur during bulb production. The TCE predominantly expressed in bulbs is TCEA, which catalyzes both PosA conversion to PaA and PosB conversion to PaB. Although its PosB-converting activity is approximately one-tenth of its PosA-converting activity, the PosB-converting activity in the bulb crude extracts is higher than that in the crude extracts from other tissues.Citation20,21) Thus, the bulbs remain a viable source of TCE.
This enzyme-mediated process is superior to a synthetic approach because it can take place in one step. In addition, the use of renewable biomass materials and an aqueous solution free of petroleum-derived solvents minimize the environmental impact of any process incorporating this enzyme.Citation36–39) However, several areas of improvement were still required, including the following: (1) The process was not optimized for preparation of PaB, (2) The bulb enzyme was for only a single use, and (3) PaB purification by selective extraction depended on the use of petroleum-derived solvents. To address these issues, we screened and selected tulip cultivars suitable as substrate sources with high PosB and low PosA flower content, and developed a method to immobilize the bulb TCE allowing its repeated use. In addition, a procedure was developed for PaB purification from the enzyme reaction mixture using only activated charcoal and ethanol. Taken together, we established an environmentally benign, low-cost, and facile process for the preparation of PaB.
Materials and methods
Plant materials
Cut flowers and bulbs of tulip cultivars were purchased from the Toyama Bulb Growers Association (Toyama, Japan). For quantification of the Pos/Pa content in each of the flower tissues (petals, stamens, and pistils), the tissues were separated from the flowers and stored at –20 °C until use. For some cultivars, plants were grown from the purchased bulbs in a field under natural conditions, and the flower tissues were collected at the flowering stage. In total, 115 cultivars were screened (Table S1). For measurement of TCE activities in crude extracts of bulbs, the bulbs of 38 cultivars (Table S2) purchased were stored at –20 °C until use. Preparation of immobilized bulb enzyme was performed using bulbs purchased in bulk in which several cultivars were randomly mixed.
Chemicals and other materials
Authentic PosA, PosB, and PaB were prepared as described previously.Citation20) PaA (α-methylene-γ-butyrolactone) was purchased from Tokyo Chemical Industry (Tokyo, Japan). Racemic PaB was chemically synthesized as follows: To a stirred solution of PaA (0.5 mmol) in acetonitrile (1 mL) was added SeO2 (0.5 mmol), and the mixture was heated under reflux for 1 h to carry out the allylic hydroxylation reaction. An extractive workup with diethyl ether followed by preparative thin-layer chromatography (n-hexane:diethyl ether = 1:3) yielded racemic PaB (22.5 mg, 39.7%) as a colorless syrup. 1H NMR (400 MHz, CDCl3): δ (ppm) 3.02 (brs, 1H, –OH), 4.20 (dd, 1H, J = 10.0, 3.6 Hz, H-4), 4.52 (dd, 1H, J = 10.0, 6.6 Hz, H-4), 4.98 (brm, 1H, H-3), 6.04 (d, 1H, J = 1.9 Hz, H-5), 6.45 (d, 1H, J = 1.9 Hz, H-5).
Activated charcoals (Yashi-coal M·MC [coconut shell-based], 8–30 mesh, and Brocoal CM [coal-based], 8–30 mesh) were purchased from Taihei Chemical Industrial (Osaka, Japan). Diatomaceous earth used as a filter aid (Radiolite #100) was purchased from Showa Chemical Industry (Tokyo, Japan). Resins for enzyme immobilization were kindly gifted from Mitsubishi Chemical (Tokyo, Japan) (DIAION-RCP160 M, -SK1B, -WK40, -WA10, -WA30, -PA312, -PA412, -HPA25L, -SA10A, -HP20, -HP20SS, -HP2MG, -SP70, -SP207, and -SP850), Sumika Chemtex (Osaka, Japan) (Duolite-A7, -A561, -A568, and -XAD761), and Toyo Denka Kogyo (Kochi, Japan) (Toyonite-200 M), or purchased from Tosoh (Tokyo, Japan) (DEAE-Toyopearl and SuperQ-Toyopearl) and Whatman (Kent, UK) (DE52).
Extraction and analysis of Pos/Pa content in flower tissues
Each of the flower tissues (petals, stamens, and pistils) stored at –20 °C was ground to a fine powder in liquid nitrogen by mortar and pestle, followed by extraction with 50% (v/v) ethanol. After centrifugation (21,500 ×g, 10 min, 4 °C), the supernatant was appropriately diluted with water and subjected to reversed-phase HPLC analysis (column, TSKgel ODS-100 V, 5 μm, 4.6 × 150 mm, Tosoh; solvent, 5% [v/v] methanol/ 10 mM phosphoric acid; flow rate, 0.65 mL/min; detection, 208 nm).
Partial purification of flower extracts with activated charcoal
Aliquots (5 mL) of flower extracts with 40% ethanol were mixed with 1/5 the volume of activated charcoal (Yashi-coal), which had been washed with water, in a 15-ml tube with rotary agitation (25 rpm) for 1 h at 4 °C. After centrifugation (21,500 ×g, 10 min, 4 °C), the supernatant was subjected to HPLC analysis as described above to monitor Pos/Pa recovery (solvent, 5% methanol/10 mM phosphoric acid) and to monitor the removal of hydrophobic impurities (solvent, 50% methanol/10 mM phosphoric acid).
Crude enzyme extraction from bulbs, and enzyme assay
Freeze-dried bulbs were ground to a fine powder by mortar and pestle and extracted with 10 mM potassium phosphate (KPi) buffer (pH 7.0). After centrifugation (21,500 ×g, 10 min, 4 °C), the supernatant, whose protein concentration was determined using a Protein Assay Kit (Bio-Rad, Hercules, CA, USA) with bovine serum albumin as a standard, was used for the enzyme assay for PosA- and PosB-converting activities. The enzyme reaction was performed in 50 mM KPi buffer (pH 6.5) containing 4 mM substrate and 10 μL of enzyme solution at several dilutions in a total volume of 50 μL. After incubation at room temperature for 10 min, the reaction was terminated by adding 50 μL of 0.5 M phosphoric acid, followed by dilution (1:5) with water. The reaction products (PaA and PaB) were detected by HPLC analysis as described above, except for the use of 10% (v/v) methanol/10 mM phosphoric acid as the solvent. One unit (U) of enzyme activity is defined as the amount of enzyme that catalyzes substrate conversion to product at a rate of 1 μmol/min.
Immobilization of bulb TCE activity on various resins
Frozen tulip bulbs (200 g fresh weight) were smashed with a hammer, suspended in 200 mL of 10 mM KPi buffer (pH 7.0), homogenized with a blender for several minutes, filtered through cheesecloth, and centrifuged (21,500 ×g, 10 min, 4 °C) to obtain the crude enzyme solution. Aliquots (5 mL) of the crude extracts were mixed with 0.5 g of each resin, which had been washed with 1 M NaCl and then equilibrated with water, in a 15-ml tube with rotary agitation (26 rpm, BIOSPIN MBS-1, EYELA, Tokyo, Japan) for 1 h at 4 °C. After centrifugation (7,600 ×g, 2 min, 4 °C), the enzyme activity remaining in the supernatant was measured using PosA as the substrate as described above, and the immobilization yield was calculated by subtracting the enzyme activity in the supernatant from that obtained before immobilization.
Large-scale preparation of immobilized bulb TCE
Frozen tulip bulbs (3 kg fresh weight) were smashed with a hammer, suspended in 6 L of 10 mM KPi buffer (pH 7.0), homogenized with a blender for several minutes, and stirred with a mechanical stirrer (170 rpm, NZ-1100, EYELA, Tokyo, Japan) for 2 h at 4 °C. The solution was filtered through cheesecloth, and the filtrate was mixed with 300 g of DIAION-HPA25L resin, which had been washed with 1 M NaCl and then equilibrated with water. After stirring for 6 h at 4 °C with a mechanical stirrer, the resin was collected by filtration with cheesecloth, washed with water until the contaminated starch granules were thoroughly removed, and stored at 4 °C in 500 mL of 10 mM KPi buffer (pH 7.0) containing 0.1% (v/v) Plant Preservative Mixture (Plant Cell Technology, NW Washington, DC, USA) as an antiseptic. The TCE activities of the immobilized enzyme for PosA and PosB were measured in 50 mM KPi buffer (pH 6.5) containing 1 mM substrate and 1 mg of immobilized enzyme resin in a total volume of 1 mL. After incubation at room temperature with rotary agitation (27 rpm) for 1 and 4 min for PosA and PosB, respectively, the reaction was terminated by adding 1 mL of 0.5 M phosphoric acid, followed by dilution (1:5) with water. Reaction products were quantified by HPLC analysis as described above, using 10% (v/v) methanol/10 mM phosphoric acid as the solvent.
Enzyme reaction of immobilized bulb TCE with flower extracts
Flower extracts in 40% ethanol were evaporated in vacuo and dissolved in water at 1/10 the volume of the 40% ethanol extracts (10× concentrated flower extracts). The enzyme reaction was performed in 50 mM KPi buffer (pH 6.5) containing 200 μL of 10× concentrated flower extracts and 5–50 mg of immobilized enzyme in a total volume of 1 mL. After incubation at room temperature with rotary agitation (27 rpm) for 15–120 min, the reaction was terminated and the reaction products were quantified as described above.
Purification of PaB from the enzyme reaction mixture
The reaction mixture (100 mL) containing 50 mM KPi buffer (pH 6.5), 20 mL of 10× concentrated flower extracts, and 5 g of immobilized enzyme was incubated at room temperature with rotary agitation for 1 h, and the reaction was terminated by removing the immobilized enzyme by filtration. Aliquots (5 mL) of the filtrate were mixed with 1/5 the volume (1 mL) of activated charcoal (Yashi-coal and Brocoal) previously washed with water and rotary agitated for 1 h. The charcoal was collected, washed with 50 volumes (50 mL) of water to remove d-glucose, which was formed from the enzyme reaction, and the reaction product was eluted with 5 volumes of aqueous ethanol (20–100%). PaB was monitored by HPLC analysis as described above using 5% (v/v) methanol/10 mM phosphoric acid as the solvent, and removal of d-glucose was monitored by silica-gel thin-layer chromatography using n-butanol/ethanol/water (5:3:2) with detection by heating the plate after dipping in a 1:9 mixture of sulfuric acid and methanol. On the basis of this experiment, Brocoal was found to be appropriate for PaB purification because a higher yield of PaB was obtained than that with Yashi-coal. A solution of 20% ethanol was adopted for the elution of PaB because it did not permit the co-elution of impurities. The volume of 20% ethanol required for PaB elution was equal to 20 volumes of Brocoal. To exchange the solvent from 20% ethanol to 100% ethanol before evaporation, the 20% ethanol eluate was mixed with 1/5 the volume of Brocoal, a process by which PaB in the eluate was re-absorbed to Brocoal. The Brocoal was washed with 50 volumes of water, and PaB was eluted with 10 volumes of ethanol, which was finally concentrated in vacuo.
Large-scale purification of PaB from tulip flowers
Frozen flowers of the cultivar “Tateyama-no-Haru” (1 kg fresh weight, 122 flowers including petals, stamens, and pistils) were soaked in 5 L of 40% (v/v) ethanol at 4 °C for 3 days. The plant residues were removed by filtration with cheesecloth, and the filtrate was mixed with 1 L of activated charcoal (Yashi-coal), which had been washed with water, for 1 h at 4 °C with a mechanical stirrer (200 rpm) to absorb hydrophobic impurities. To trap the microparticles of Yashi-coal, 100 g of diatomite (Radiolite #100) was added to the solution, followed by filtration with a diatomite pad (Radiolite #100, approximately 1 cm thick) on filter paper. The filtrate was evaporated, and the residue was dissolved in water (500 mL in final) to obtain 10× concentrated, partially purified flower extracts.
The enzyme reaction was performed in 50 mM KPi buffer (pH 6.5) containing 500 mL of the 10× concentrated flower extracts and 125 g of the immobilized bulb TCE (prepared as described above) in a total volume of 2.5 L. After stirring at room temperature for 2.5 h with a mechanical stirrer (110 rpm), the reaction was terminated by removing the immobilized enzyme by filtration. To the filtrate was added 500 mL of activated charcoal (Brocoal), which had been washed with water, and stirred for 1 h at room temperature with a mechanical stirrer (200 rpm) to absorb PaB. The unbound fraction was removed by filtration, and the Brocoal was washed with 25 L of water in a batch-wise manner. Washed Brocoal was collected by filtration and suspended in 10 L of 20% (v/v) ethanol to elute PaB, followed by filtration. To the filtrate was added 2 L of another portion of Brocoal, and the mixture was stirred for 3 h at room temperature with a mechanical stirrer (200 rpm). The unbound fraction was removed by filtration, and the Brocoal was washed with 100 L of water. Washed Brocoal was collected by filtration and suspended in 20 L of ethanol to elute PaB. After the charcoal was removed by filtration, the filtrate was mixed with 400 g of diatomite (Radiolite #100) and filtered through a diatomite pad (Radiolite #100, approximately 1 cm thick) on a filter paper to remove the microparticles of Brocoal. Finally, the filtrate was evaporated in vacuo to obtain PaB as a colorless oil.
The identity and purity of the purified PaB were confirmed by reversed-phase HPLC analysis as described above (solvent, 5% methanol/10 mM phosphoric acid) and NMR analysis using a Bruker AVANCE 400 spectrometer (Bruker, Karlsruhe, Germany). 1H NMR (400 MHz, CDCl3): δ (ppm) 3.00 (brs, 1H, –OH), 4.20 (dd, 1H, J = 10.0, 3.6 Hz, H-4), 4.52 (dd, 1H, J = 10.0, 6.6 Hz, H-4), 4.97 (brm, 1H, H-3), 6.04 (d, 1H, J = 1.8 Hz, H-5), 6.45 (d, 1H, J = 2.0 Hz, H-5); 13C NMR (100 MHz, CDCl3): δ (ppm) 67.6 (C-4), 73.5 (C-3), 126.7 (C-5), 137.7 (C-2), 169.3 (C-1). Optical purity was assessed by chiral HPLC analysis (column, Chiralpak-ID, 5 μm, 4.6 × 250 mm, Daicel [Osaka, Japan]; solvent, n-hexane:2-propanol (85:15); flow rate, 0.8 mL/min; detection, 240 nm) using chemically synthesized racemic PaB as a reference.
Results and discussion
Screening of the elite tulip cultivars suitable for PaB preparation
When tulip flowers are used as source of PosB, which is enzymatically converted to PaB by TCE, it is preferable to use flower tissues containing a high content of PosB and a low content of PosA in order to facilitate PaB purification from the reaction mixture. Thus, we screened 115 tulip cultivars for their Pos/Pa content in each type of flower tissues, i.e. petals, stamens, and pistils (Table S1). As described previously,Citation10,17) PosA and PosB were detected as major compounds and PaA and PaB as minor components in the flower tissues. The PosA/PaA and PosB/PaB content differed considerably with the cultivar and the tissue. As a consequence, we identified 28 cultivars that possess flower tissue(s) whose PosB/PaB content exceeded 90% of the total Pos/Pa content (Table ). Of those, 25 cultivars had only one or two tissues whose PosB/PaB content exceeded this level. If these cultivars were used as the PosB source, the tissues would need to be segregated before extraction in order to minimize the PosA/PaA incorporation, which is impractical for scale-up purposes. Thus, the cultivars “Design Impression”, “Kurobe-no-Taiyo”, and “Tateyama-no-Haru”, in which the PosB/PaB content exceeded 90% of the total Pos/Pa content in all three flower tissues (Table , Table S1), were the most appropriate PosB sources and enabled the use of all flower parts.
Table 1. Cultivars and tissues where the PosB/PaB content exceeded 90% of the total Pos/Pa content.
Preparation of flower extracts as a substrate for the enzyme reaction
In order to prepare flower extracts that can be used as a substrate for the enzyme reaction, we optimized the procedure with a focus on the extraction solvent and partial purification as follows.
(a) Extraction solvent
To establish a PaB preparation process without the use of petroleum-derived solvents, we adopted ethanol, which is one of the most environmentally preferred solvents,Citation37) for extracting the flower tissues. A high concentration of ethanol was considered to be unfavorable because it increased the chance of extracting hydrophobic impurities, and it necessitated the use of a large amount of ethanol. On the other hand, the use of aqueous ethanol lower than 40% was also unfavorable for the following partial purification of the flower extracts with activated charcoal, because, at lower percentages of ethanol, the activated charcoal absorbed both the hydrophobic impurities and the PosB instead of allowing PosB to collect in the unbound fraction (see below (b)). Thus, we decided to use 40% ethanol as the extraction solvent. When the flower tissues were extracted by soaking in 5 volumes of 40% ethanol at 4 °C, the extractable PosB content was saturated within 2–3 days and was stable at least for 2 weeks.
(b) Partial purification of the flower extracts
Because the flower extracts containing 40% ethanol could not directly be used as a substrate for the enzyme reaction with the immobilized bulb TCE due to inhibition of the activity by ethanol (see next section (d)), it first needed to be removed by evaporation. When the extracts were checked for hydrophobic impurities by reversed-phase HPLC with 50% methanol/10 mM phosphoric acid as the solvent, multiple peaks that were not detected by analysis with 5% methanol/10 mM phosphoric acid, the condition used for the detection of Pos/Pa, were found to exist (Fig. S1). Removing these hydrophobic impurities as much as possible prior to evaporation of the flower extracts not only facilitates PaB purification following the enzyme reaction, but also reduces issues with increased viscosity of the concentrated extracts after evaporation. Considering that one objective of this research was to establish an environmentally benign process, we adopted coconut shell-derived activated charcoal (Yashi-coal) as an absorbent of the hydrophobic impurities for partial purification of the flower extracts.
Following the removal of plant residues from the extracts by passing through cheesecloth, the filtrate containing 40% ethanol was mixed in a batch-wise manner with 1/5 the volume of water-washed Yashi-coal, which resulted in the removal of a large portion of the hydrophobic substances (Fig. S1), and yielded 80% PosB in the unbound fraction. Using an amount of Yashi-coal greater than 1/5 the volume dramatically lowered the PosB yield in the unbound fraction. When removing the Yashi-coal after the treatment, filtration with a diatomite pad (Radiolite #100) on a filter paper was effective to remove the microparticles of Yashi-coal. The clear filtrate was evaporated to remove the ethanol followed by dissolving in water, so as to prepare the 10× concentrated flower extracts that were used as the substrate for the enzyme reaction.
Use of tulip bulbs as a source of the TCE
TCE activities are detectable in all tulip tissues, but the specific activities and the activity ratios toward PosA and PosB differ with the tissue.Citation20) Although the TCE expressed predominantly in bulbs is TCEA, the bulb crude extracts have PosB-converting activity because the TCEA also has PosB-converting activity, although it is approximately 10 times lower than the PosA-converting activity.Citation19–21) Nevertheless, the PosB-converting activity in the bulb crude extracts is higher than that in the crude extracts from other tissues because TCE activities are very high in bulbs.Citation20) Thus, bulbs are suitable as a source of TCE for PaB preparation. In this study, we attempted to immobilize the TCE in an effort to stabilize it and allow reuse of the enzyme. We optimized various parameters in terms of the preparation, reaction, and storage of the immobilized enzyme as follows.
(a) Variation of the bulb TCE activity in the different tulip cultivars
Since we plan to use unsalable irregular bulbs of various cultivars in bulk, we first checked the TCE activities in the bulb crude extracts of 38 randomly selected cultivars. In all cultivars tested, TCE activities were detected, and the PosA-converting activity was higher than the PosB-converting activity (Table S2), which was consistent with our previous observation for a single cultivar (“Murasakizuisho”).Citation19–21,35) On average, specific activities for PosA and PosB were 26.9 ± 2.55 U/mg protein and 4.42 ± 0.618 U/mg protein (mean ± SE), respectively. Although the PosB-converting activity of the cultivar “Rosy Wings” was considerably lower than those of other cultivars, considering that the bulbs of various cultivars are used in bulk as enzyme source, the presence of such cultivar with a low frequency does not make a severe impact on the PosB-converting activity of the immobilized enzyme.
(b) Selection of the immobilization carrier
The immobilization efficiency of the TCE activity in the bulb crude extracts was compared between three cation- and 12 anion-exchange resins, and eight hydrophobic resins at pH 7.0 by adding 10% (w/v) resin to the crude extracts. As shown in Fig. , resins generally used for protein purification, such as DEAE cellulose (DE52), DEAE Toyopearl, and SuperQ Toyopearl, quantitatively bound the TCE. Despite their useful performance qualities, using such expensive carriers is unfavorable for scale up of the procedure from the viewpoint of cost saving. Among other low-cost resins tested, only DIAION-HPA25L, a highly porous strong anion-exchange resin with a quaternary ammonium group, exhibited an immobilization yield of over 50% (approximately 75%). Since the DIAION-HPA25L resin is moderate in price and is used for industrial process applications, we adopted this resin as the immobilization carrier. Increasing the amount of resin increased the immobilization yield, although it became saturated at around 80%. An immobilization yield of around 70% was reproducibly achieved when 5% (w/v) resin (based on the volume of the bulb crude extract) was used, and thus, this was employed for all further experiments.
Fig. 2. Immobilization yield of the bulb TCE with various resins.
Note: PosA-converting activity in the bulb crude extracts was set to 100%. After mixing the crude extracts with each resin, the activity in the unbound fraction was subtracted from the initial activity to calculate the activity bound to the resin.
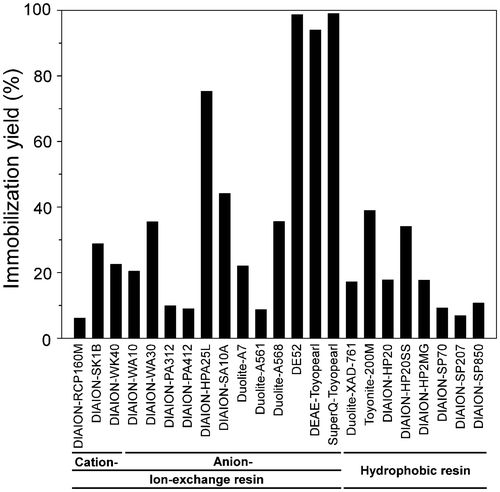
(c) Stability of the immobilized enzyme
Bulb enzyme immobilized on DIAION-HPA25L in 10 mM KPi buffer (pH 7.0) was stored in the same buffer with an antiseptic. Activities of the immobilized enzyme toward PosA and PosB were 230 and 22 U/g resin, respectively, at 1 mM substrate. Leakage of the enzyme activity from the resin only occurred to a small extent in buffer up to 100 mM, but did in the presence of over 100 mM NaCl in 100 mM KPi buffer. For example, approximately 20 and 60% of the immobilized activities were eluted at 100 and 500 mM NaCl, respectively. Changes in PosB-converting activity during storage were examined through 10 weeks. As shown in Fig. (A), the activity was reduced only slightly throughout the period, confirming the advantage which immobilization confers on the enzyme stability. In general, the most obvious benefit of enzyme immobilization is allowing the reuse of the enzyme.Citation40) We tested the reusability of the immobilized bulb enzyme by repeating the reaction five times using the same sample. Even in the fifth reaction, approximately 70% of the initial activity was retained (Fig. (B)). These results indicate that the bulb TCE was stably immobilized, providing cost savings over the use of an enzyme sample one time.
Fig. 3. Stability of the bulb enzyme immobilized on DIAION-HPA25L resin.
Note: (A) Time-course change in the PosB-converting activity during the 70-day storage period. (B) Effect of the number of uses on the PosB-converting activity. The activity at the initial use was set to 100%. The immobilized enzyme collected after each reaction was washed with water and used for the next reaction.
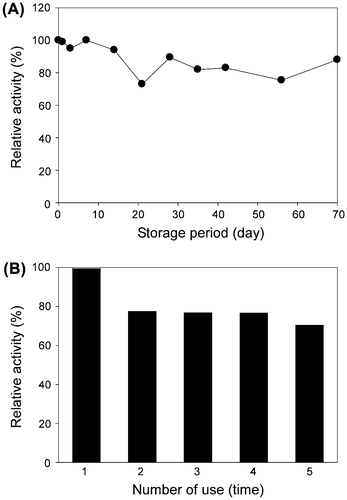
(d) Reactivity of the immobilized enzyme with flower extracts
The flower extracts used as a substrate for the enzyme reaction are prepared with 40% ethanol as described above. When the TCE activity of the immobilized bulb enzyme was measured in the presence of 40% ethanol, the activity was reduced by approximately 80%. Accordingly, the flower extracts were evaporated to remove ethanol, and a 10× concentrated aqueous solution was prepared. To examine the reactivity of the immobilized bulb enzyme with the concentrated flower extracts, enzyme reactions were performed using different amounts of immobilized enzyme, i.e. 0.5, 1, 2, and 5% (w/v) of the reaction mixture, in the presence of a 2× final substrate solution, and the time-dependent decrease in PosB in the reaction mixture was monitored. A clear dependency of the reaction rate on the amount of immobilized enzyme was observed (Fig. ), and the PosB-conversion to PaB was completed in 60 min in the presence of 5% immobilized enzyme (Figs. and ). These results indicate that the concentrated flower extracts do not contain substances that inhibit the immobilized bulb enzyme and can be directly used as a substrate. Based on these results, the amount of immobilized enzyme used for the reaction was set to 5% (w/v) of the reaction mixture.
Fig. 4. Reaction rate achieved by catalysis using different amounts of immobilized bulb enzyme.
Note: The reaction mixture containing 1/5 the volume of 10× concentrated flower extracts (2× final concentration) and the bulb enzyme immobilized on DIAION-HPA25L resin was incubated at room temperature, and the decrease in PosB was monitored. The amounts of the immobilized enzyme used were 0.5 (closed circle), 1 (open circle), 2 (closed triangle), and 5% (open triangle) (w/v) of the reaction mixture. The initial PosB content in the mixture was set to 100%.
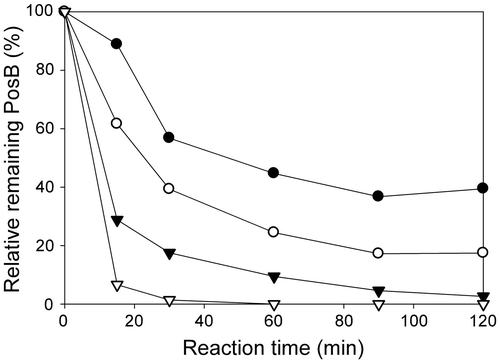
Fig. 5. HPLC chromatograms of the flower extracts before (upper panel) and after (lower panel) reaction with the bulb enzyme immobilized on DIAION-HPA25L resin.
Note: PosA and PosB in the flower extracts were thoroughly converted to PaA and PaB, respectively, by the enzyme reaction. Two peaks for each of PosA and PosB correspond to the 1α- (the later peak) and 1β- (the former peak) anomers.
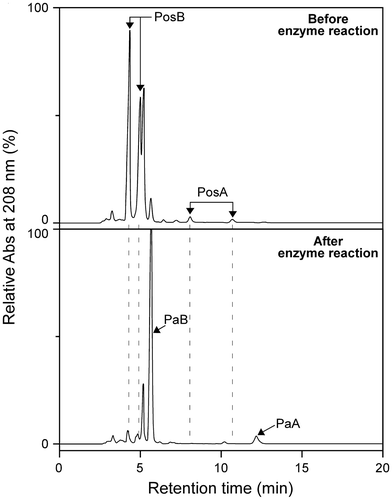
Purification of PaB from the enzyme reaction mixture
Enzymatic conversion of Pos to Pa by TCE is accompanied by the equimolar formation of d-glucose (Fig. ). In addition, the reaction mixture also contains other impurities derived from flowers. We previously reported the purification procedure of PaA and PaB by selective extraction using organic solvents. PaA is extracted with toluene, and then, PaB is extracted with a 1:1 mixture of n-butanol and acetone.Citation35) However, each extraction step cannot be conducted without cross extraction, and using organic solvents is not environmentally friendly. Thus, we attempted to develop a PaB purification procedure using activated charcoal and ethanol as the absorbent and solvent, respectively. When Yashi-coal was added to the reaction mixture, PaB was quantitatively absorbed to it. After washing out the impurities with water, the efficiency of the PaB elution with aqueous ethanol (20–100%) was tested. However, since the PaB yield was low, we tested Brocoal, a coal-based charcoal, for this purpose. While Brocoal quantitatively absorbed PaB to a similar extent as Yashi-coal, PaB could be eluted from Brocoal in a higher yield than Yashi-coal at each concentration of aqueous ethanol (data not shown). Thus, we adopted Brocoal as an absorbent for PaB purification. Since PaB elution from Brocoal using greater than 20% ethanol resulted in the co-elution of impurities, the ethanol concentration of the eluent was set to 20%, whereby approximately 60% of PaB absorbed to the Brocoal was eluted. PaB purification was indeed achieved from the 20% ethanol eluate, but it was time- and energy-consuming to concentrate PaB in 20% ethanol by evaporation. We therefore did a solvent exchange to 100% ethanol: approximately 80% of PaB contained in the 20% ethanol could be absorbed to the Brocoal a second time by adding 1/5 the volume of Brocoal, and PaB absorbed was then eluted with 100% ethanol. Evaporation of 100% ethanol, which is easily achieved, afforded purified PaB.
Large-scale purification of PaB from tulip flowers by the optimized procedure
Of the three cultivars, “Design Impression”, “Kurobe-no-Taiyo”, and “Tateyama-no-Haru”, selected from the 115 cultivars as those suitable as a substrate source, “Tateyama-no-Haru” was used for testing the large-scale preparation of PaB by the optimized procedure (Fig. ). A total of 1 kg of the flowers was soaked in 40% ethanol for 3 days. As shown in Table , the major component in the extracts was PosB, while the PosB/PaB content reached 90.5% of the total Pos/Pa content. The concentrated Yashi-coal-treated extracts were subjected to the immobilized bulb TCE which completely converted PosB in the reaction mixture to PaB. The PaB was then absorbed to Brocoal and eluted with 20% ethanol after the impurities were washed out by treating with water. Following the solvent exchange to 100% ethanol and evaporation, 2.68 mmol (306 mg) of purified PaB was obtained with 10% overall yield (Table ). Purified PaB contained only a trace amount of PaA (Fig. (A)), and its purity (PaB/[PaA + PaB]) was 98.8%. Chiral HPLC analysis with chemically synthesized racemic PaB as a reference confirmed that the purified PaB was optically pure (3S form,>99.9% e.e.) (Fig. (B)).
Table 2. Purification of PaB from 1 kg of flowers of cultivar “Tateyama-no-Haru”.
Fig. 7. HPLC analysis of PaB purified from the flowers of the cultivar “Tateyama-no-Haru” by the process reported herein.
Note: (A) Reversed-phase HPLC analysis showing the purity of the purified PaB. Only a trace of PaA was detected as the impurity. (B) Chiral HPLC analysis showing the optical purity of the purified PaB. PaB purified from flowers gave a peak corresponding to the 3S enantiomer (natural PaB), but not the 3R enantiomer. The peak was monitored at 240 nm, because monitoring at 208 nm, i.e. near PaB λmax, did not give a horizontally-linear baseline under the solvent system used (n-hexane:2-propanol = 85:15).
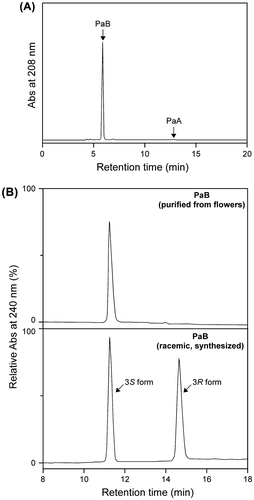
The yield of PaB decreased with each successive activated charcoal treatment. For example, the PaB yield after purification from the enzyme reaction mixture was 40% (7.06/17.3 mmol), and the yield was 44% (3.13/7.06 mmol) after the solvent was exchanged to ethanol. Future work will focus on improving the overall yield of these two steps. In this study, in order to achieve a facile process, the activated charcoal treatments were performed in a batch-wise manner. However, generally a column chromatography procedure is higher yielding and provides better separation than a batch procedure, although it is a time-consuming process. The choice of a column versus a batch procedure should be made based on the trade-off between the yield of PaB and the simplicity of the process.
Recently, we identified genes encoding TCEA from tulip petals and bulbs.Citation20,21) The establishment of a heterologous expression system in E. coli permits the use of recombinant enzyme instead of the natural bulb enzyme. The use of recombinant enzyme would not be preferable for certain uses of PaB, e.g. as a cosmetic preservative, but it is advantageous that the enzyme can be independently prepared regardless of whether the yearly supply of tulip bulbs is available or not. In this context, the use of recombinant TCEB, which exhibits superior catalytic activity toward PosB than TCEA, is preferable for PaB preparation because it reduces the required amount of enzyme and reaction time. Given the recent identification of the gene for TCEB (T. Nomura, S. Ogita, and Y. Kato, unpublished work), establishment of such an alternative process is now in progress.
Conclusion
We demonstrated the establishment of an enzyme-mediated process for the preparation of PaB, an antibacterial natural product from tulip, without the use of petroleum-derived solvents. The process described herein features the use of renewable biomass materials of tulip as substrate and enzyme sources, a quantitative one-step enzyme reaction with reusable immobilized enzyme, and the purification of PaB in a batch-wise manner using only activated charcoal and ethanol. The process is not only simpler but also more environmentally benign than previous synthetic approaches and should prove to be a viable green process for natural product preparation. Continued efforts should be devoted to scaling up the process and improving the yield, so that PaB can be employed in many industrial applications.
Supplemental material
The supplemental material for this paper is available at http://dx.doi.org/10.1080/09168451.2014.946395
Supplemental Materials
Download MS Word (414 KB)Additional information
Funding
Notes
Enzyme commission number of the tuliposide-converting enzyme, which we reported previously as EC 2.3.1.-,Citation21) has been corrected to EC 4.2.99.-. Tuliposide A-converting enzyme is numbered as EC 4.2.99.22; however, the fourth number for tuliposide B-converting enzyme has not yet been assigned.
Abbreviations: PosA, 6-tuliposide A; PosB, 6-tuliposide B; PaA, tulipalin A; PaB, tulipalin B; TCE, tuliposide-converting enzyme; TCEA, tuliposide A-converting enzyme; TCEB, tuliposide B-converting enzyme.
References
- Jenecka A, Wyrębska A, Gach K, Fichna J, Janecki T. Natural and synthetic α-methylenelactones and α-methylenelactams with anticancer potential. Drug Discov. Today. 2012;17:561–572.10.1016/j.drudis.2012.01.013
- Jun-Tao F, De-Long W, Yong-Ling W, He Y, Xing Z. New antifungal scaffold from a natural pharmacophore: Synthesis of α-methylene-γ-butyrolactone derivatives and their antifungal activity against Colletotrichum lagenarium. Bioorg. Med. Chem. Lett. 2013;23:4393–4397.10.1016/j.bmcl.2013.05.073
- Cavallito CJ, Haskell T. α-Methylene butyrolactone from Erythronium americanum. J. Am. Chem. Soc. 1946;68:2332–2334.10.1021/ja01215a057
- Bergman BHH, Beijersbergen JCM, Overeem JC, Kaars Sijpesteijn A. Isolation and identification of α-methylenebutyrolactone, a fungitoxic substance from tulips. Recl. Trav. Chim. Pays-Bas. 1967;86:709–714.
- Bergman BHH, Beijersbergen JCM. A fungitoxic substance extracted from tulips and its possible role as a protectant against disease. Neth. J. Plant Pathol. 1968;74:157–162.10.1007/BF01977525
- Verspyck Mijnssen GAW. Pathogenesis and causative agent of “tulip finger”. Br. J. Dermatol. 1969;81:737–745.10.1111/bjd.1969.81.issue-10
- Tschesche R, Kämmerer F-J, Wulff G, Schönbeck F. Über die antibiotisch wirksamen der tulpe (Tulipa gesneriana) [On the antibiotically active substances of the tulip (Tulipa gesneriana)]. Tetrahedron Lett. 1968;9:701–706.10.1016/S0040-4039(00)75615-2
- Tschesche R, Kämmerer F-J, Wulff G. Über die struktur der antibiotisch aktiven substanzen der tulpe (Tulipa gesneriana L.) [On the structure of antibiotically active substances of the tulip (Tulipa gesneriana L.)]. Chem. Ber. 1969;102:2057–2071.10.1002/(ISSN)1099-0682
- Beijersbergen JCM. A method for determination of tulipalin A and B concentrations in crude extracts of tulip tissues. Recl. Trav. Chim. Pays-Bas. 1972;91:1193–1200.
- Beijersbergen JCM, Lemmers CBG. Enzymic and non-enzymic liberation of tulipalin A (α-methylene butyrolactone) in extracts of tulip. Physiol. Plant Pathol. 1972;2:265–270.10.1016/0048-4059(72)90009-4
- Schönbeck F, Schroeder C. Role of antimicrobial substances (tuliposides) in tulips attacked by Botrytis spp. Physiol. Plant Pathol. 1972;2:91–99.10.1016/0048-4059(72)90015-X
- Mendgen T, Scholz T, Klein CD. Structure-activity relationships of tulipalines, tuliposides, and related compounds as inhibitors of MurA. Bioorg. Med. Chem. Lett. 2010;20:5757–5762.10.1016/j.bmcl.2010.07.139
- Shigetomi K, Shoji K, Mitsuhashi S, Ubukata M. The antibacterial properties of 6-tuliposide B: synthesis of 6-tuliposide B analogues and structure-activity relationship. Phytochemistry. 2010;71:312–324.10.1016/j.phytochem.2009.10.008
- Shigetomi K, Omoto S, Kato Y, Ubukata M. Asymmetric total synthesis of 6-tuliposide B and its biological activities against tulip pathogenic fungi. Biosci. Biotechnol. Biochem. 2011;75:718–722.10.1271/bbb.100845
- Shigetomi K, Olesen SH, Yang Y, Mitsuhashi S, Schönbrunn E, Ubukata M. MurA as a primary target of tulipalin B and 6-tuliposide B. Biosci. Biotechnol. Biochem. 2013;77:2517–2519.10.1271/bbb.130663
- Slob A, Varekamp HQ. Tuliposide contents of tulip (Tulipa) species and cultivars during the flowering stage. Proc. K. Ned. Acad. Wet. C Biol Med. Sci. 1977;80:201–211.
- Christensen LP, Kristiansen K. Isolation and quantification of tuliposides and tulipalins in tulips (Tulipa) by high-performance liquid chromatography. Contact Dermat. 1999;40:300–309.10.1111/cod.1999.40.issue-6
- van Rossum MWPC, Alberda M, van der Plas LHM. Tulipaline and tuliposide in cultured explants of tulip bulb scales. Phytochemistry. 1998;49:723–729.10.1016/S0031-9422(98)00199-X
- Kato Y, Shoji K, Ubukata M, Shigetomi K, Sato Y, Nakajima N, Ogita S. Purification and characterization of a tuliposide-converting enzyme from bulbs of Tulipa gesneriana. Biosci. Biotechnol. Biochem. 2009;73:1895–1897.10.1271/bbb.90226
- Nomura T, Ogita S, Kato Y. A novel lactone-forming carboxylesterase: molecular identification of a tuliposide A-converting enzyme in tulip. Plant Physiol. 2012;159:565–578.10.1104/pp.112.195388
- Nomura T, Tsuchigami A, Ogita S, Kato Y. Molecular diversity of tuliposide A-converting enzyme in the tulip. Biosci. Biotechnol. Biochem. 2013;77:1042–1048.10.1271/bbb.130021
- Hoffmann HMR, Rabe J. Synthesis and biological activity of α-methylene-γ-butyrolactones. Angew. Chem. Int. Ed. Eng. 1985;24:94–110.10.1002/(ISSN)1521-3773
- Jean M, Roch ML, Renault J, Uriac P. Synthesis of a laterally branched polyamine from α-methylene-γ-butyrolactone. Org. Lett. 2005;7:2663–2665.10.1021/ol050803x
- Kuroda M, Yoshida D, Mizusaki S. Bio-antimutagenic effect of lactones on chemical mutagenesis in Escherichia coli. Agric. Biol. Chem. 1986;50:243–245.10.1271/bbb1961.50.243
- Kim C-S, Hara T, Datta PK, Itoh E, Horiike M. Insecticidal component in thunberg spiraea, Spiraea thunbergii, against Thrips palmi. Biosci. Biotechnol. Biochem. 1998;62:1546–1549.10.1271/bbb.62.1546
- Agarwal S, Jin Q, Maji S. Biobased polymers from plant-derived tulipalin A. In: Smith PB, Gross RA, editors. Biobased monomers, polymers, and materials, ACS symposium series. Washington (DC): American Chemical Society; 2012. p. 197–212.10.1021/symposium
- Slob A. Tulip allergens in Alstroemeria and some other Liliflorae. Phytochemistry. 1973;12:811–815.10.1016/0031-9422(73)80684-3
- Barbier P, Benezra C. Allergenic α-methylene-γ-butyrolactones. Study of the capacity of β-acetoxy- and β-hydroxy-α-methylene-γ-butyrolactones to induce allergic contact dermatitis in guinea pigs. J. Med. Chem. 1986;29:868–871.10.1021/jm00155a046
- Papageorgiou C, Stampf J-L, Benezra C. Allergic contact dermatitis to tulips: an example of enantiospecificity. Arch. Dermatol. Res. 1988;280:5–7.10.1007/BF00412680
- Tanaka A, Yamashita K. Synthesis of (S)-(+)-methyl β, γ-dihydroxy-α-methylenebutyrate and (S)-(-)-tulipalin B. Agric. Biol. Chem. 1980;44:199–202.10.1271/bbb1961.44.199
- Papageorgiou C, Benezra C. Use of enzymic hydrolysis of dimethyl malates for a short synthesis of tulipalin B and of its enantiomer. J. Org. Chem. 1985;50:1144–1145.10.1021/jo00207a054
- Ohgiya T, Nishiyama S. Synthesis of (-)-tulipalin B utilizing 2-bromo-1-alkenes conveniently synthesized from the 3-O-substituted 1,2-dibromoalkane system by regioselective elimination. Heterocycles. 2004;63:2349–2354.
- Shigetomi K, Kishimoto T, Shoji K, Ubukata M. Synthesis of tulipalin B and 1-O-methyl-6-tuliposide B. Heterocycles. 2006;69:63–67.
- Shigetomi K, Kishimoto T, Shoji K, Ubukata M. First total synthesis of 6-tuliposide B. Tetrahedron: Asymmetry. 2008;19:1444–1449.10.1016/j.tetasy.2008.05.019
- Kato Y, Yoshida H, Shoji K, Sato Y, Nakajima N, Ogita S. A facile method for the preparation of α-methylene-γ-butyrolactones from tulip tissues by enzyme-mediated conversion. Tetrahedron Lett. 2009;50:4751–4753.10.1016/j.tetlet.2009.06.018
- Tang SLY, Smith RL, Poliakoff M. Principles of green chemistry: productivity. Green Chem. 2005;7:761–762.10.1039/b513020b
- Capello C, Fischer U, Hungerbühler K. What is a green solvent? A comprehensive framework for the environmental assessment of solvents. Green Chem. 2007;9:927–934.10.1039/b617536h
- Horváth IT, Anastas PT. Innovations and green chemistry. Chem. Rev. 2007;107:2169–2173.10.1021/cr078380v
- Li C-J, Trost BM. Green chemistry for chemical synthesis. Proc. Natl. Acad. Sci. USA. 2008;105:13197–13202.10.1073/pnas.0804348105
- Buchholz K, Kasche V, Bornscheuer U. Biocatalysts and enzyme technology. 2nd ed. Weinheim: Wiley-Blackwell; 2012.