Abstract
Some Lactobacillus brevis strains were found to aggregate upon the addition of xylan after screening for lactic acid bacteria that interact with plant materials. The S-layer proteins of cell surface varied among the strains. The strains that displayed xylan-mediated aggregation retained its ability even after the removal of S-layer proteins. L. brevis had negative zeta potentials. A correlation between the strength of aggregation and zeta potential was not observed. However, partial removal of S-layer proteins resulted in decreases in the electric potential and aggregation ability of some strains. Therefore, xylan-mediated aggregation of L. brevis was considered to be caused by an electrostatic effect between the cells and xylan. L. brevis also aggregated in the presence of mucin, and the strengths of aggregation among the strains were similar to that induced by xylan. Thus, xylan- and mucin-mediated L. brevis aggregation was supposed to be caused by a similar mechanism.
Graphical Abstract
Some Lactobacillus brevis strains were found to aggregate upon the addition of xylan, and these strains also aggregated in the presence of mucin.
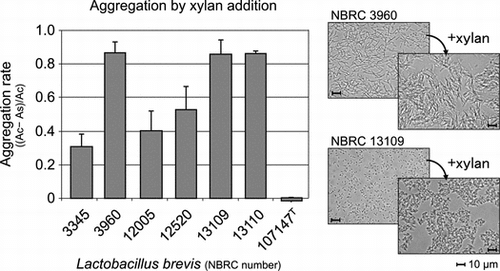
Lactic acid bacteria (LAB) are used in a variety of fermented foods such as yogurt, cheese, miso, soy sauce, kimchi, and pickles. They are also known as probiotics, which provide physiological benefits to humans and animals.Citation1) Recent studies on LAB primarily focused on their physiological functions, their interactions with the host and intestinal microbes, and their applications for providing functional food.Citation2,3) On the contrary, ecological functions of LAB, particularly the interaction between LAB and plants, have not been investigated, although LAB are widely found in nature and have been isolated from plantsCitation4,5); they are also used in fermented foods made from plant materials. Recently, Lactobacillus delbrueckii ssp. bulgaricus and Streptococcus thermophilus, which are used in yoghurt production, were isolated from the plant Cornus mas, and it was considered that these yogurt starters originated from plants.Citation6) Thus, the ecological functions of LAB, originally held in nature, have been utilized in human life for food processing and probiotic therapy. In addition, elucidating the actions of LAB with plants may help to clarify the fermentation process and physiological functions of LAB in the production of not only fermented foods made from plant materials but also dairy products.
One of the known properties of LAB as probiotics is adhesion to the host cell, particularly to those in the gastrointestinal tract.Citation7) The factors and mechanism of action between LAB and the host have been studied, and LAB surface factors such as proteins and/or carbohydrates were found to recognize intestinal mucin or other sugar chains of the host.Citation8,9) LAB strains belonging to Lactobacillus brevis and Lactobacillus gasseri that recognize human blood type-A antigen through recognition of the sugar chain of the antigen were reported.Citation10,11) Mannose-specific adhesion to the porcine jejunal epithelium by Lactobacillus plantarumCitation12) and coaggregation between and among human intestinal and oral bacteria via lectin–carbohydrate interactionsCitation13) have also been reported. Furthermore, the aggregation of LAB and yeast cells mediated by mannose and/or mannan in the yeast cell wall has been confirmed for L. plantarumCitation14,15) and Lactococcus lactis.Citation16) In this manner, it was considered that the adhesion of LAB to the host cell and other microbes occurred in a lectin-like manner through the carbohydrate. Similar mechanisms would be expected to occur between LAB and plants, which are mainly constituted by carbohydrates. However, to the best of our knowledge, research from the viewpoint of adhesion and recognition between plants and LAB has not yet been conducted.
Based on the aforementioned background, we attempted to screen LAB that interacts with carbohydrates in plants. As a result, some strains of L. brevis were observed to aggregate in the presence of xylan. In this paper, xylan-mediated aggregation of L. brevis and its relationship to cell surface properties were investigated to elucidate this aggregation effect for clarifying the actions of LAB with plants. The relationship between xylan- and mucin-mediated aggregations was also described in order to confirm the relationship of the interaction of LAB with plants and other hosts.
Materials and methods
Bacterial strains and culture conditions
LAB strains used in this study were L. brevis NBRC 3345, 3690, 12005, 12520, 13109, 13110, 107147T, and our stock strains (41 strains of 14 species). NBRC strains were obtained from the Biological Resource Center (NBRC), National Institute of Technology and Evaluation, Japan. The strains were maintained using half-strength Lactobacilli MRS broth (Becton, Dickinson and Company, Franklin Lakes, NJ) with 1.5% agar at 30 °C. They were inoculated into Lactobacilli MRS broth and incubated at 30 °C for 24 h, and 1% of the culture broth was transferred into fresh medium. After culturing at 30 °C for 24 h, the cells were harvested by centrifugation, washed with saline solution, and used in further experiments.
Aggregation assays
Xylan from oat spelts, mucin from porcine stomach (Sigma–Aldrich, St. Louis, MO), and cellulose powder (Oriental yeast Co. Ltd, Tokyo, Japan) were used in the aggregation assays with LAB strains. Harvested cells were suspended in saline solution to produce an optical density of almost 1 at 600 nm at the initiation of the assay. Xylan or cellulose powder was directly added to the cell suspension at a concentration of 10 g/L. With regard to the addition of the soluble fraction of xylan and mucin, the solutions were prepared by obtaining the supernatant via centrifugation after suspending 20 g/L xylan or 6 g/L mucin in saline solution for 1 h with shaking at room temperature. Each solution was added to the same volume of the cells suspension. As a control, the same volume of saline solution was added to the cells suspension instead of xylan or mucin solution. After addition of the solutions, the suspensions were mixed well by vortexing and incubated at 30 °C with static. A portion of the upper suspension was transferred from the center of the solution to another tube at designated intervals, and optical density was measured at 600 nm.Citation17) The aggregation rate was calculated according to a previous report Citation17) with some modifications using the formula (Ac − As)/Ac, where As and Ac represent the optical density of the sample and the control, respectively.
To evaluate the effect of the addition of various sugars on LAB aggregation, sugars were added to the suspension at a concentration of 20 g/L. The effect of pH on the aggregation was evaluated using 0.1 M Britton–Robinson buffer with pH ranging from 2.0 to 12.0. The effect of temperature was assessed by changing the temperature during incubating the suspension. The effect of NaCl concentration was evaluated by adding NaCl solution to the assay suspension at concentrations of 1, 2, and 2.5 M, respectively.
Analysis of the cell surface proteins
The cell surface proteins of LAB strains were analyzed as previously described.Citation18) Harvested cells equivalent to 0.5 mL of broth were suspended in 40 μL of Laemmli sample buffer and incubated for 4 min at 95 °C. After centrifugation, the supernatant was analyzed by SDS-PAGE using 10% Mini-PROTEAN TGX Gel (Bio-Rad Laboratories, Hercules, CA) with Tris/glycine/SDS buffer and stained with Coomassie brilliant blue.
For protein identificationCitation19,20), the stained gel was excised in a clean cabinet, destained with 50% acetonitrile in 50 mM NH4HCO3, and dehydrated with 100% acetonitrile. Subsequently, the sample was reduced with 10 mM DTT at 56 °C for 45 min and alkylated with 55 mM iodoacetamide for 30 min at room temperature. Trypsin (Sigma–Aldrich, proteomics grade) digestion (10 ng/μL) was performed overnight at 37 °C prior to peptide extraction in 50% acetonitrile in 50 mM NH4HCO3. After the concentration of the solution in vacuo, the peptides were desalted using ZipTip C18 pipette tips (Millipore, Billerica, MA). The MALDI-TOF mass spectra of the obtained peptides were recorded on a 4800 plus MALDI TOF/TOF analyzer (AB SCIEX, Framingham, MA) using α-cyano-4-hydroxycinnamic acid (Bruker Daltonics, Inc., Billerica, MA) as the matrix in the positive-ion mode with an acceleration voltage of 20 kV. The mass spectrometer was tuned and calibrated using a commercially available standard peptide mixture (peptide Calibration Standard II, Bruker Daltonics) prior to the measurement. Obtained data were applied to the Mascot search engine (Matrix Science, London, UK) over the database of NCBInr (National Center for Biotechnology Information nonredundant, updated 20120927 with 7,061,663,751 sequences). All spectra were searched using the following parameters: taxonomy, bacteria (12,697,784 sequences); enzyme, trypsin; fixed modification, carbamidoethylation of C; variable modifications, deamidation of N and Q and oxidation of M; miss cleavages, up to 1; and mass tolerances, ± 100 ppm and ± 0.25 Da for precursor and product ions, respectively.
Removal and reattachment of S-layer proteins
Harvested cells were suspended in a half volume of 0–6 M LiCl solution for 1 h at room temperature with shaking. The cells were recovered by centrifugation, washed with saline solution, and subjected to aggregation assay, surface protein analysis, and zeta potential measurements. In the case of preparing NaCl-treated cells, NaCl solution was used in place of LiCl solution.
The cells in which the S-layer protein was removed using 6 M LiCl were subjected to reattachment of S-layer proteins to the cells. The cells were mixed with a 2-fold amount of S-layer protein extracts obtained as supernatants after 6 M LiCl treatment. The mixtures were dialyzed against water overnight, and the dialysates were washed with saline and used in the aggregation assay.
Measurement of the zeta potential of the cells
The zeta potential of the cells was measured by the particle microscopic electrophoresis method using a Zeecom ZC-2000 instrument (Microtec Co. Ltd, Funabashi, Japan).Citation21) Harvested cells were washed with 10 mM phosphate buffer (pH 6.8), suspended in the same buffer, and applied to the instrument under a voltage of 20 V. The electrophoretic mobility of more than 10 cells was automatically measured, and the zeta potential was calculated according to the Smoluchowski equation.
Analytical methods
Microscopic analysis was performed using a Leica DMLB microscope (Leica Microsystems, Wetzlar, Germany).
Each measurement was repeated at least three times. Data are presented as mean values with standard deviation.
Results and discussion
Aggregation of L. brevis mediated by xylan
Preliminary screening of LAB strains for interactions with xylan or cellulose powder was conducted using our stock strains (two strains of L. brevis, one of Lactobacillus cellobiosus, one of Lactobacillus curvatus, three of Lactobacillus fermentum, one of Lactobacillus pentosus, seven of Lactobacillus plantarum, one of Lactobacillus rhamnosus, three of Lactobacillus sake, three of Leuconostoc citreum, one of Leu. lactis, twelve of Leu. mesentroides, one of Leu. sp., one of Pediococcus acidilactici, three of P. pentosaceus, and one of Enterococcus mundtii). As a result, one L. brevis strain rapidly aggregated upon the addition of xylan compared with the strain in the absence of xylan. Similar changes were not observed upon the addition of cellulose or with other strains. The aggregation abilities of all of L. brevis strains in NBRC in response to xylan solution were evaluated to examine this aggregation effect in detail. It was found that the aggregation abilities and its rates varied among the strains (Fig. (A)). L. brevis NBRC 3960, 13109, and 13110 strongly aggregated, whereas NBRC 3345, 12005, and 12520 demonstrated moderate aggregation. Formation of the cell aggregates was observed with these strains (Fig. (B)). The soluble fraction of xylan was found to induce aggregation in these strains, whereas the insoluble xylan, which was obtained as the precipitate after centrifugation of saline washes of xylan powder, did not induce aggregation. In contrast, L. brevis NBRC 107147T did not react with xylan.
Fig. 1. Aggregation rates of L. brevis strains after the addition of xylan (A) and microscopic analysis of the strains in the presence (a, c) and absence (b, d) of xylan (B). The representative results by L. brevis strains NBRC 3960 (a, b) and NBRC 13109 (c, d) are shown as the results of optical microscopic analysis. As and Ac represent the optical density at 600 nm of the sample with xylan and the control without xylan, respectively. The soluble fraction of xylan was used, and data for 2 h after xylan addition are shown.
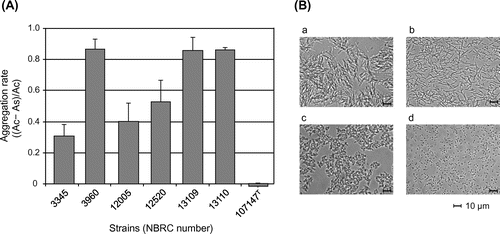
The effects of temperature, pH, and the addition of sugars on xylan-mediated aggregation were assessed using L. brevis NBRC 3960. The aggregation rate increased with increasing temperature up to 40 °C, after which the rate rapidly decreased (data not shown). Aggregation was stable between the pH range 3–12, excluding a significant decrease in aggregation at pH 11 (data not shown), which corresponds to the pI value of the S-layer protein of Lactobacillus.Citation22) Xylan-mediated aggregation was not inhibited by d-glucose, d-xylose, l-arabinose, d-mannose, d-galactose, l-rhamnose, sucrose, maltose, trehalose, lactose, raffinose, N-acetylglucosamine, xylitol, fructooligosaccharides, xylooligosaccharides, gentiooligosaccharides, or isomaltooligosaccharides (data not shown). From this observation, the aggregation of L. brevis in response to xylan was not considered to involve a specific reaction mediated by proteins, such as lectin-like proteins, which were previously identified as factors for the aggregation and gastrointestinal tract adherence of Lactobacillus.Citation8,9,12–15,23) However, another type of protein was considered to mediate xylan-induced aggregation based on the effects of pH and temperature on aggregation.
Analysis of the cell surface proteins of L. brevis
The cell surface proteins of L. brevis were analyzed by SDS-PAGE (Fig. ) and identified by mass spectrometry after in-gel digestion of the proteins (Table ). Major proteins corresponding to band No. 1, which were identified in L. brevis NBRC 3345, 3690, 107147T and suggested in NBRC 10225, 12520, 13109, and 13110 to be the S-layer proteins of L. brevis, varied among the strains, whereas the protein corresponding to band No. 3, which was identified as phosphoglycerate mutase, existed in common among the strains. Difference in relation to the aggregation ability between L. brevis NBRC 107147T and another strain appeared to be in band No. 2. The strains that aggregated in response to xylan expressed a protein corresponding to LVIS_1982 or xylanase/chitin deacetylase. Conversely, L. brevis NBRC 107147T did not express this protein; and a protein corresponding to Slp D or LVIS_1974 was identified in band No 2.
Fig. 2. Analysis of the cell surface proteins of L. brevis by SDS-PAGE. The numbers next to the bands denote the proteins subjected to identification.
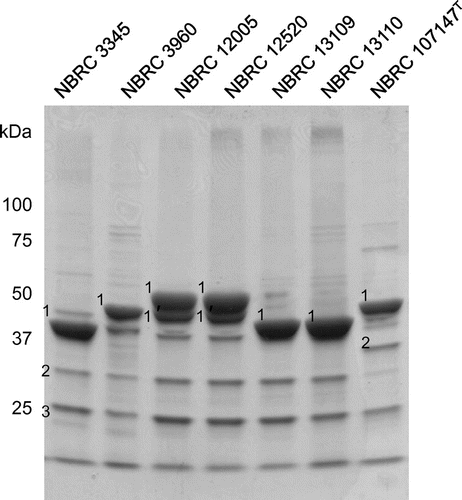
Table 1. Identification of the cell surface proteins of L. brevis by MALDI-TOF mass spectrometry.
S-layer proteins are known to be able to reassemble and reattach to the cell wall.Citation22,24–27) Thus, reattachment of S-layer proteins to the same strain and other strains was attempted to confirm their involvement in xylan-mediated aggregation. After the removal of S-layer proteins by treatment with 6 M LiCl, the aggregation rate decreased in some of the strains, as shown in Fig. . Among them, the aggregation rates of L. brevis NBRC 13109 and 13110 were recovered after reattachment of their original S-layer proteins (data not shown). Furthermore, the strain that did not aggregate in the presence of xylan, L. brevis NBRC 107147T, displayed slight aggregation activity in response to xylan after attachment of the S-layer protein of L. brevis NBRC 3345 (data not shown). Although there were variations in the data obtained from the results of reattachment of S-layer proteins to both of original (L. brevis NBRC 13109 and 13110) and heterogeneous (L. brevis NBRC 107147T) strains and these phenomena were not observed all strains, this observation was considered to indicate the participation of S-layer proteins in xylan-induced aggregation.
Fig. 3. Effect of LiCl treatment on the xylan-mediated aggregation rate and zeta potential of L. brevis. As and Ac represent the optical density at 600 nm of the sample with xylan and the control without xylan, respectively. The soluble fraction of xylan was used for the aggregation assay, and data for 2 h after xylan addition are shown. aThere was one outlier in aggregation rate of L. brevis NBRC 3960, which was treated with 4 M LiCl, and the value was −1.83 ± 0.58.
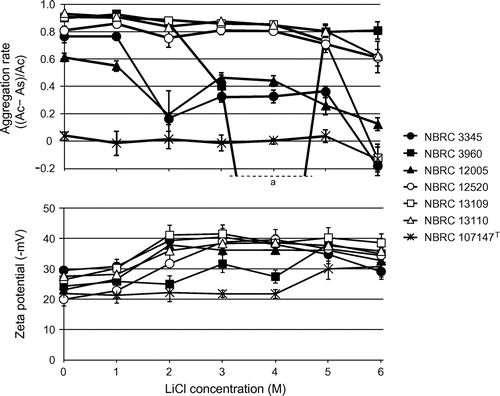
Effect of LiCl treatment on the xylan-mediated aggregation, zeta potential, and remaining S-layer proteins of L. brevis
The aggregation rate of each strain was slightly decreased by low concentrations of LiCl, and the aggregation abilities of most strains were destroyed or halved by 6 M LiCl treatment (Fig. ). However, the strains with aggregation ability retained this ability even after the removal of most of S-layer proteins (Figs. and ). All strains had negative zeta potentials and absolute electric charges were increased by LiCl treatment (Fig. ). A correlation between the strength of aggregation and zeta potential was definitely not established, although the absolute value of zeta potentials increased in all strains when S-layer proteins were removed (Figs. and ).
Fig. 4. Analysis of the remaining S-layer proteins of L. brevis after LiCl treatment. The bands corresponding to proteins labeled “1” in Figure are shown.
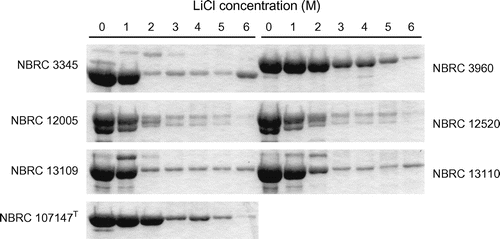
From this observation, xylan-induced aggregation was caused by an electrical property of the cells; this was because aggregation was not affected by cell surface components before and after LiCl treatment, and the essence of this aggregation was considered to be due to electrostatic effects occurring between the charged cells and xylan. The cells had a negative charge and were surrounded by positively charged ions such as a hydrogen ion, and it was considered that the cells diffused in the solution without xylan via repulsion as shown in Fig. (B). Xylan is known to possess a negative chargeCitation28), and aggregation of the cells occurred via their attachment to xylan, which was mediated by the ions in the solution. To confirm the electrostatic effect on the aggregation, the effect of NaCl concentration on the aggregation was evaluated. Both of intact cells and LiCl-treated cells, which were removed most of S-layer protein, were used for the analysis to avoid the influence of NaCl on S-layer protein because part of the protein was seemed to be removed by 5 M NaCl treatment in the strains except for L. brevis NBRC 3960 and NBRC 107147T (data not shown). The aggregation rates of both of intact and LiCl-treated cells decreased with increasing NaCl concentration (Fig. ). Thus, since the aggregation effect was reduced under the high ionic strength conditions, the electrostatic effect was involved in the xylan-mediated aggregation of L. brevis. However, it is difficult to explain the case of L. brevis NBRC 107147T, which did not display xylan-induced aggregation in spite of having an electric charge equivalent to the other strains. Therefore, it was believed that the S-layer proteins and other cell surface components such as teichoic acid, which was also considered to participate in the aggregation and adhesion of LactobacillusCitation29,30), control the stability of the electric charges and/or xylan affinity of the cells, and that xylan-induced aggregation was mediated by such complex factors.
Fig. 5. Effect of NaCl concentration on the xylan-mediated aggregation rate of both of intact and LiCl-treated L. brevis. As and Ac represent the optical density at 600 nm of the sample with xylan and the control without xylan, respectively. The soluble fraction of xylan was used for the aggregation assay, and data for 1 h after xylan addition are shown. aStandard assay condition contained 154 mM NaCl which came from saline solution. bThe concentration of LiCl treatment was 6 M for L. brevis NBRC 3960 and NBRC 107147T and 3 M for the other strains, respectively.
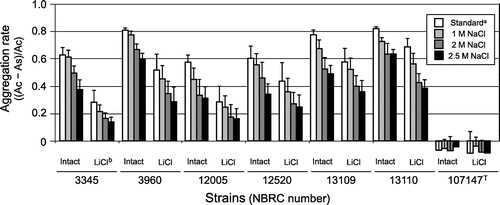
Remarkable decrements in xylan-induced aggregation were observed in L. brevis NBRC 3960 after treatment with 4 M LiCl (Fig. ). The aggregation rate of the strain under this condition was negative, indicating that self-aggregation of the cells occurred. The S-layer protein content of the cells under this condition was almost half the amount of that in the intact cells (Fig. ), and a temporal decrement of the electric charge of the cells was observed (Fig. ). In addition, similar tendencies were observed in L. brevis NBRC 3345 and 12005 after treatment with 2 M LiCl. Thus, it was considered that the unstable state of the cell surface, including the partial removal of S-layer proteins, largely affected the electric charge of the cells, and that the aggregation of the cells was influenced by the resulting charge. This hypothesis also supported the consideration that the aggregation of L. brevis in response to xylan was caused by electrostatic action.
Time course of the aggregation of L. brevis in response to xylan and mucin
It was suggested that xylan-mediated L. brevis aggregation occurred via a nonspecific electrostatic action, and thus, aggregation should also be induced by another substrate, mucin, which has a negative charge similar to xylan.Citation28) As shown in Fig. , L. brevis strains also aggregated in the presence of mucin, and the strength of aggregation among the strains was similar to that induced by xylan. Thus, it was supposed that xylan- and mucin-mediated L. brevis aggregation was caused by a similar mechanism, and that adhesion to these substrates was one of the fundamental properties of L. brevis for environmental adaptation in nature.
Fig. 6. Time course of the aggregation rate of L. brevis after xylan and mucin addition. As and Ac represent the optical density at 600 nm of the sample with xylan or mucin and the control without them, respectively. The soluble fractions of xylan and mucin were used.
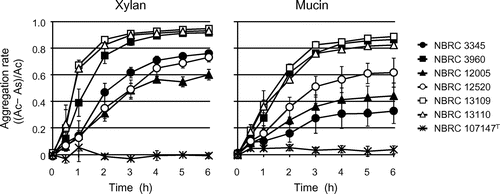
The probiotic effects of L. brevis and other Lactobacillus strains on adhesion to intestinal mucin, mucosa, and cells had been widely studied, and S-layer proteins and the aggregation ability of the strains were found to be associated with their probiotic effects.Citation31–34) However, the mechanism responsible for the probiotic effects of intestinal bacteria remains unclear, and an examination of the adhesion properties of Lactobacillus using plant materials such as xylan had not been previously conducted. Thus, this study, which described the xylan-mediated aggregation of L. brevis for the first time as well as its association with mucin-mediated aggregation, and future investigations may help to elucidate both the fundamental ecological function of Lactobacillus spp. and physiological functions such as adhesion to the gastrointestinal tract. In addition, the adhesion of LAB to food and its raw materials is important as the initial step of the fermentation. Therefore, the study showed the attachment of L. brevis to such materials as xylan, which is contained in a lot of kind of raw materials of fermented foods, and also difference in the ability among the strains will be useful for elucidation of the fermentation mechanism and development of a control method of the fermentation process. In order to apply this aggregation effect to the above utilization, further study on the aggregation of the bacteria will be conducted.
Acknowledgment
We thank I. Kubota, T. Sato, Y. Hori, and R. Nagata for their technical assistance.
References
- Mitsuoka T. Significance of dietary modulation of intestinal flora and intestinal environment. Biosci. Microflora. 2000;19:15–25.10.12938/bifidus1996.19.15
- Rajasekaran A, Kalaivani M. Designer foods and their benefits: a review. J. Food Sci. Technol. 2013;50:1–16.
- Vieira AT, Teixeira MM, Martins FS. The role of probiotics and prebiotics in inducing gut immunity. Front. Immunol. doi: 10.3389/fimmu.2013.00445.
- Mundt JO, Hammer JL. Lactobacilli on plants. Appl. Microbiol. 1968;16:1326–1330.
- Okada S. The world of plant origin lactic acid bacteria. Jpn. J. Lactic Acid Bacteria. 2002;13:23–36. ( in Japanese).
- Michaylova M, Minkova S, Kimura K, Sasaki T, Isawa K. Isolation and characterization of Lactobacillus delbrueckii ssp. bulgaricus and Streptococcus thermophilus from plants in Bulgaria. FEMS Microbiol. Lett. 2007;269:160–169.10.1111/fml.2007.269.issue-1
- Ohashi Y, Ushida K. Health-beneficial effects of probiotics: its mode of action. Anim. Sci. J. 2009;80:361–371.10.1111/asj.2009.80.issue-4
- Vélez MP, De Keersmaecker SC, Vanderleyden J. Adherence factors of Lactobacillus in the human gastrointestinal tract. FEMS Microbiol. Lett. 2007;276:140–148.10.1111/fml.2007.276.issue-2
- Sengupta R, Altermann E, Anderson RC, McNabb WC, Moughan PJ, Roy NC. The role of cell surface architecture of lactobacilli in host-microbe interactions in the gastrointestinal tract. Mediators Inflamm. 2013;2013:237921. doi: 10.1155/2013/237921.
- Uchida H, Kawai Y, Kinoshita H, Kitazawa H, Miura K, Shiiba K, Horii A, Kimura K, Taketomo N, Oda M, Yajima T, Saito T. Lactic acid bacteria (LAB) bind to human B- or H-antigens expressed on intestinal mucosa. Biosci. Biotechnol. Biochem. 2006;70:3073–3076.10.1271/bbb.60407
- Uchida H, Kinoshita H, Kawai Y, Kitazawa H, Miura K, Shiiba K, Horii A, Kimura K, Taketomo N, Oda M, Yajima T, Saito T. Lactobacilli binding human A-antigen expressed in intestinal mucosa. Res. Microbiol. 2006;157:659–665.10.1016/j.resmic.2006.03.001
- Gross G, van der Meulen J, Snel J, van der Meer R, Kleerebezem M, Niewold TA, Hulst MM, Smits MA. Mannose-specific interaction of Lactobacillus plantarum with porcine jejunal epithelium. FEMS Immunol. Med. Microbiol. 2008;54:215–223.10.1111/fim.2008.54.issue-2
- Ledder RG, Timperley AS, Friswell MK, Macfarlane S, McBain AJ. Coaggregation between and among human intestinal and oral bacteria. FEMS Microbiol. Ecol. 2008;66:630–636.10.1111/fem.2008.66.issue-3
- Pretzer G, Snel J, Molenaar D, Wiersma A, Bron PA, Lambert J, de Vos WM, van der Meer R, Smits MA, Kleerebezem M. Biodiversity-based identification and functional characterization of the mannose-specific adhesin of Lactobacillus plantarum. J. Bacteriol. 2005;187:6128–6136.10.1128/JB.187.17.6128-6136.2005
- Furukawa S, Nojima N, Yoshida K, Hirayama S, Ogihara H, Morinaga Y. The importance of inter-species cell-cell co-aggregation between Lactobacillus plantarum ML11-11 and Saccharomyces cerevisiae BY4741 in mixed-species biofilm formation. Biosci. Biotechnol. Biochem. 2011;75:1430–1434.10.1271/bbb.100817
- Katakura Y, Sano R, Hashimoto T, Ninomiya K, Shioya S. Lactic acid bacteria display on the cell surface cytosolic proteins that recognize yeast mannan. Appl. Microbiol. Biotechnol. 2010;86:319–326.10.1007/s00253-009-2295-y
- Kos B, Susković J, Vuković S, Simpraga M, Frece J, Matosić S. Adhesion and aggregation ability of probiotic strain Lactobacillus acidophilus M92. J. Appl. Microbiol. 2003;94:981–987.10.1046/j.1365-2672.2003.01915.x
- Jakava-Viljanen M, Avall-Jaaskelainen S, Messner P, Sleytr UB, Palva A. Isolation of three new surface layer protein genes (slp) from Lactobacillus brevis ATCC 14869 and characterization of the change in their expression under aerated and anaerobic conditions. J. Bacteriol. 2002;184:6786–6795.10.1128/JB.184.24.6786-6795.2002
- Oe M, Ohnishi-Kameyama M, Nakajima I, Muroya S, Chikuni K. Muscle type specific expression of tropomyosin isoforms in bovine skeletal muscles. Meat Sci. 2007;75:558–563.10.1016/j.meatsci.2006.09.003
- Yamamoto M, Ohnishi-Kameyama M, Nguyen CL, Taguchi F, Chiku K, Ishii T, Ono H, Yoshida M, Ichinose Y. Identification of genes involved in the glycosylation of modified viosamine of flagellins in Pseudomonas syringae by mass spectrometry. Genes. 2011;2:788–803.10.3390/genes2040788
- Ouhara K, Komatsuzawa H, Yamada S, Shiba H, Fujiwara T, Ohara M, Sayama K, Hashimoto K, Kurihara H, Sugai M. Susceptibilities of periodontopathogenic and cariogenic bacteria to antibacterial peptides, β-defensins and LL37, produced by human epithelial cells. J. Antimicrob. Chemother. 2005;55:888–896.10.1093/jac/dki103
- Hynönen U, Palva A. Lactobacillus surface layer proteins: structure, function and applications. Appl. Microbiol. Biotechnol. 2013;97:5225–5243.10.1007/s00253-013-4962-2
- Iniguez-Palomares C, Jimenez-Flores R, Vazquez-Moreno L, Ramos-Clamont-Montfort G, Acedo-Felix E. Protein-carbohydrate interactions between Lactobacillus salivarius and pig mucins. J. Anim. Sci. 2011;89:3125–3131.10.2527/jas.2010-2996
- Lortal S, Heijenoort J, Gruber K, Sleytr UB. S-layer of Lactobacillus helveticus ATCC 12046: isolation, chemical characterization and re-formation after extraction with lithium chloride. J. Gen. Microbiol. 1992;138:611–618.10.1099/00221287-138-3-611
- Masuda K, Kawata T. Reassembly of the regularly arranged subunits in the cell wall os Lactobacillus brevis and their reattachment to cell walls. Microbiol. Immunol. 1980;24:299–308.10.1111/mim.1980.24.issue-4
- Mobili P, Gerbino E, Tymczyszyn EE, Gómez-Zavaglia A. S-layers in lactobacilli: structural characteristics and putative role in surface and probiotic properties of whole bacteria. In: Méndez-Vilas A, editor. Current research, technology and education topics in applied microbiology and microbial biotechnology. Badajoz: Formatex Research Center; 2010. p. 1224–1234.
- Smit E, Oling F, Demel R, Martinez B, Pouwels PH. The S-layer protein of Lactobacillus acidophilus ATCC 4356: identification and characterisation of domains responsible for S-protein assembly and cell wall binding. J. Mol. Biol. 2001;305:245–257.10.1006/jmbi.2000.4258
- Claesson PM. Interactions between surfaces coated with carbohydrates, glycolipids, and glycoproteins. In: Malmsten M, editor. Biopolymers at interfaces. 2nd ed. New York (NY): Marcel Dekker; 2013. p. 165–197.
- Granato D, Perotti F, Masserey I, Rouvet M, Golliard M, Servin A, Brassart D. Cell surface-associated lipoteichoic acid acts as an adhesion factor for attachment of Lactobacillus johnsonii La1 to human enterocyte-like Caco-2 cells. Appl. Environ. Microbiol. 1999;65:1071–1077.
- Reniero R, Cocconcelli P, Bottazzi V, Morelli L High frequency of conjugation in Lactobacillus mediated by an aggregation-promoting factor. J. Gen. Microbiol. 1992;138:763–768.10.1099/00221287-138-4-763
- Beganović J, Frece J, Kos B, Leboš Pavunc A, Habjanič K, Sušković J. Functionality of the S-layer protein from the probiotic strain Lactobacillus helveticus M92. Antonie Van Leeuwenhoek. 2011;100:43–53.10.1007/s10482-011-9563-4
- Frece J, Kos B, Svetec IK, Zgaga Z, Mrsa V, Suskovic J. Importance of S-layer proteins in probiotic activity of Lactobacillus acidophilus M92. J. Appl. Microbiol. 2005;98:285–292.10.1111/jam.2005.98.issue-2
- Juntunen M, Kirjavainen PV, Ouwehand AC, Salminen SJ, Isolauri E. Adherence of probiotic bacteria to human intestinal mucus in healthy infants and during rotavirus infection. Clin. Diagn. Lab. Immunol. 2001;8:293–296.
- Xu H, Jeong HS, Lee HY, Ahn J. Assessment of cell surface properties and adhesion potential of selected probiotic strains. Lett. Appl. Microbiol. 2009;49:434–442.10.1111/lam.2009.49.issue-4