Abstract
Two genes encoding RelA/SpoT homologs, PpRSH2a and PpRSH2b, which are involved in the synthesis of bacterial alarmone guanosine 5′-diphosphate 3′-diphosphate (ppGpp) for the stringent response, were isolated from the moss, Physcomitrella patens. A complementary analysis of PpRSH2a and PpRSH2b in Escherichia coli showed that these genes had ppGpp biosynthetic activity. The recombinant PpRSH2a and PpRSH2b were also shown to synthesize ppGpp in vitro. Both proteins were localized to the chloroplasts of P. patens. Expression of the PpRSH genes was induced upon treatment with abscisic acid or abiotic stresses, such as dehydration and UV irradiation. Overexpression of PpRSH2a and PpRSH2b caused suppression of the growth in response to 1% (w/v) of glucose. The present study suggests the existence of a mechanism to regulate the growth of P. patens, which is governed by plant RSH in chloroplasts
Graphical Abstract
Functional analysis of RelA/SpoT homologues (RSHs) suggests the existence of a mechanism to regulate the growth of P. patens, which is governed by ppGpp in chloroplasts.
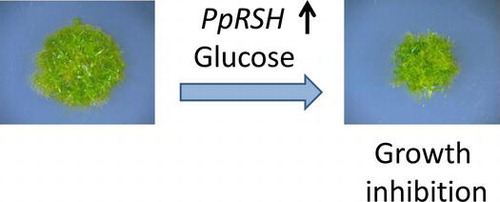
Organisms evolve a wide variety of physiological processes and molecular mechanisms for survival. In bacteria, the stringent response is one of the most significant and well-investigated gene regulatory systemsCitation1,2); it allows cells to adapt to stressful stimuli, such as the starvation of amino acids, phosphorus, carbon sources, iron, or fatty acids.Citation3–6) The signaling molecules for the stringent response are guanosine 5′-diphosphate 3′-diphosphate (ppGpp) and guanosine 5′-triphosphate 3′-diphosphate (pppGpp), which are collectively termed (p)ppGpp or bacterial alarmone. When bacteria perceive stress signals induced by unfavorable environmental changes, ribosome-dependent ppGpp synthesis is catalyzed by the relA gene product (RelA), ppGpp synthetase (also called stringent factor), in response to the binding of an uncharged tRNA to the ribosomal A site. RelA converts GTP to pppGpp by the addition of a pyrophosphate group from ATP onto the 3′ position of GTP, and, subsequently, pppGpp is converted to ppGpp by the hydrolysis of the triphosphate group. The spoT gene product (SpoT) catalyzes the conversion of ppGpp into GDP. In Escherichia coli, RelA and SpoT exist independently; nevertheless, in other bacteria, the synthetic and/or hydrolytic activities of RelA/SpoT homolog (RSH) proteins mediate the stringent response.Citation1) Additionally, the novel bacterial ppGpp synthases, small alarmone synthase has been also identified in Streptococcus mutansCitation7) and Bacillus subtilis.Citation8)
RSH genes have been identified in plants, such as Arabidopsis thaliana, Chlamydomonas reinharditii, Nicotiana tabacum, and Oryza sativa.Citation9–13) It has been shown that ppGpp is accumulated in chloroplasts by the catalytic function of chloroplast-localizing RSH enzymes,Citation14) which is in agreement with the finding that most plant RSH proteins are transferred from the cytosol to chloroplasts.Citation10–13) The accumulation of ppGpp is stimulated by plant hormones, such as abscisic acid (ABA) or jasmonic acid (JA), which are induced under stressful conditions.Citation14) A disrupted mutant of AtCRSH, which is one of the RSH genes in A. thaliana, demonstrated unusual flower development, suggesting that the AtCRSH gene affects the reproductive process in this plant.Citation13) Moreover, experiments involving the crosslinking of 6-thioppGpp with chloroplast RNA polymerase indicated that ppGpp binds to the β′ subunit of bacterial-type chloroplast RNA polymerase (plastid-encoded plastid RNA polymerase; PEP) to inhibit transcription.Citation15) Given that chloroplasts are presumably derived from cyanobacteria and that there are many similarities between the genetic systems of chloroplasts and cyanobacteria, it has been suggested that (p)ppGpp has a critical role in chloroplasts.Citation16) Additionally, Nomura et al. showed that ppGpp inhibits peptide elongation cycle of chloroplast translation system in vitro; ppGpp signaling system in translation of bacteria might be conserved in that of chloroplasts.Citation17) Recently, ppGpp was shown to inhibit guanylate kinase, an important enzyme in guanine nucleotide biosynthesis, in chloroplasts of plants.Citation18) On the other hand, nucleoside diphosphates linked to some moiety X (Nudix) hydrolases, AtNUDXs, has been shown to have ppGpp pyrophosphohydrolase activity in A. thaliana. The metabolic pathway of ppGpp without RelA/SpoT protein is shown to exist in plants.Citation19)
In terms of the evolutionary history of plants, bryophytes (mosses, liverworts, and hornworts) are considered intermediates between algae and vascular plants, forming one of the oldest groups of land plants.Citation20) The divergence of vascular plants from mosses extends back at least 400 million years; accordingly, there may be differences in the physiological processes and responses of these separate divisions. An understanding of the differences in the physiological processes could provide valuable insights into plant evolution.
The moss, Physcomitrella patens, has been widely used as a model plant because the simple developmental process facilitates the study of basic physiological processes in the plant. Furthermore, P. patens is only one of a few multicellular organisms with highly efficient homologous recombination.Citation21) The genome of P. patens has been elucidated,Citation22) and a database search revealed the presence of several putative RelA/SpoT homologs (PpRSHs) in P. patens. This study indicates that RelA/SpoT homologs play a function in the physiological processes of P. patens. Specifically, PpRSH2a and PpRSH2b are shown to get participated in the growth of P. patens.
Materials and methods
Materials
The wild-type strain of P. patens subsp. patens was used in this study.Citation23) P. patens was cultivated on BCDAT or BCDATG agar medium under continuous white fluorescent light at 25 °C. BCDAT medium contains 5 mM diammonium (+)-tartrate, 1 mM CaCl2, 1 mM MgSO4, 10 mM KNO3, 45 μM FeSO4, 1.8 mM KH2PO4 (pH 6.5 adjusted by KOH), and a trace element solution (alternative TES; 0.22 μM CuSO4, 0.19 μM ZnSO4, 10 μM H3BO3, 0.10 μM Na2MoO4, 2 μM MnCl2, 0.23 μM CoCl2, and 0.17 μM KI). BCDATG medium is BCDAT medium supplemented with 0.5% (w/v) glucose. Both media were solidified with 0.8% (w/v) agar (Nakarai tesque, Japan).Citation24)
Analysis of the expression of PpRSHs under ordinary growth conditions
The amino-acid sequence of AtRSH2 (A. thaliana, accession number NP_188021) was used as a reference sequence in searching for homologous genes in the P. patens genomic database, COSMOSS (http://www.cosmoss.org). The detailed semi-quantitative RT-PCR method is described in the supporting information.
Cloning of PpRSH1 and PpRSH2
Total RNA was extracted from P. patens grown at 25 °C for 3 days on BCDATG agar medium under continuous white fluorescent light. Reverse transcription (M-MLV reverse transcriptase; Invitrogen, USA) was carried out according to the manufacturer’s instructions to yield cDNA for use as a PCR template. The primer sets used for PCR amplification were as follows: for PpRSH2a, PpRSH2a-NcoIF with an NcoI site (5′-ttccatggtgaggctagcggaa-3′) and PpRSH2a-XhoIR with an XhoI site (5′-ttctcgagtcacatgagaccagcg-3′) and for PpRSH2b, PpRSH2b-NcoIF with an NcoI site (5′-tttagtccatgggccaaccaact-3′) and PpRSH2b-SacIR with a SacI site (5′-aaagagctctcagattccagcaa-3′). These primers were designed on the basis of the sequences of PpRSH2a (XM_001754893) and PpRSH2b (XM_001785010), respectively. The PCR reaction was optimized for 50 μL of reaction mixture per tube containing 10 μL of dNTP mixture (2 mM each), 1 μL each of both primers (10 μM), 1 μL of cDNA, 25 μL of PCR buffer (2X), 1 μL of KOD FX DNA polymerase (Toyobo, Japan), and 12 μL of Milli-Q water. The reaction parameters were set to 94 °C for 2 min (initial denaturation), 98 °C for 10 s, 56 °C for 30 s, and 68 °C for 1 min 50 s (35 cycles) followed by a final extension at 68 °C for 10 min. The PCR products were analyzed by gel electrophoresis and visualized by staining with ethidium bromide. The amplified DNA fragments were purified from the gel and digested with the corresponding restriction enzymes (Takara, Japan). The digested products were subsequently cloned into pET21d (Merck, USA) to obtain the pETRSH2a and pETRSH2b plasmids for the complementation analysis. These plasmids were sequenced to confirm that no mutation occurred during the PCR.
Complementation analysis of PpRSH1 and PpRSH2
The E. coli strains, W3110 (λDE3, wild type), CF1652 (λDE3, relA), and CF1678 (λDE3, relA spoT), were used for the complementation analysis under isopropyl-β-D-thiogalactopyranoside (IPTG)-inducible gene expression from the T7 promoter.Citation10) These strains were transformed with the pETRSH2a and pETRSH2b plasmids or the empty vector (pET21d) using a standard procedure. The transformed strains were grown at 37 °C for 60 h on 3-(N-morpholino)propanesulfonic acid (MOPS)–agar medium (40 mM MOPS and 4 mM tricine–KOH buffer [tricine = N-(Tris[hydroxymethyl]methyl)glycine] (pH 7.2), 50 mM KCl, 10 mM NH4Cl, 0.5 mM MgSO4, 0.2 mM KH2PO4, 10 μM FeCl3, and 1.5% agar) supplemented with 0.4% glucose and 0.1 mg/mL each of serine, methionine, and glycine in the absence or presence of 50 μM IPTG.Citation25)
In vitro synthesis of (p)ppGpp by the recombinant PpRSH1 and PpRSH2 proteins
For cell-free protein synthesis, we constructed pTD1 plasmids containing PpRSH2a and PpRSH2b. The primer sets used for the PCR amplification were PpRSH2a-pF (5′-atgttgaggctagcggaaga-3′) and PpRSH2a-pR (5′-tcacatgagaccagcgacgct-3′) for PpRSH2a and PpRSH2b-SmaIF with a SmaI site (5′-atattacccgggtatgggccaaccaactga-3′) and PpRSH2b-SacIR2 with a SacI site (5′-cgggagctctcagattccagcaacattaag-3′) for PpRSH2b. The PCR reaction (final volume of 50 μL) contained 5 μL of PCR buffer (10X), 5 μL of dNTP mixture (2 mM each), 3 μL of 25 mM MgSO4, 1.5 μL each of both primers (10 μM), 0.5 μg of template DNA, 1 μL of KOD-Plus (Toyobo, Japan), and 31 μL of Milli-Q water. The pETRSH2a and pETRSH2b plasmids were used as the templates, and the conditions for the PCR were those described above. Both of the PCR products were purified and the DNA fragment of PpRSH2b was digested with SmaI and SacI (Takara, Japan). The intact DNA fragments of PpRSH2a and the digested DNA fragments of PpRSH2b were cloned into pTD1, which was digested with EcoRV (Takara, Japan) or EcoRV (Takara) and SacI (Takara) to generate pTDRSH2a or pTDRSH2b, respectively. The in vitro transcription and translation reactions were performed using RiboMAX Large-Scale RNA Production Systems (Promega, USA) and the Transdirect insect cell system (Shimadzu, Japan), according to the manufacturers’ instructions. To confirm the synthesis of PpRSH2a and PpRSH2b, 1 μL of [35S]methionine (370 MBq/mL, 37 Tbq/mmol, MP Biomedicals, Santa Ana, CA, USA) was added to 50 μL of the reaction mixture of the insect cell-free protein synthesis, and then protein synthesis was carried out according to the manufacturer’s instructions. The products (15 μL of the reaction mixture) were analyzed by SDS-PAGE analysis and the protein bands of PpRSH2a and PpRSH2b were visualized by autoradiography (Fig. S3). The reaction mixtures (final volume of 20 μL) used to assay the (p)ppGpp synthetic activity contained 30 mM HEPES-KOH (HEPES = 4-[2-hydroxyethyl]piperazin-1-ethanesulfonic acid) (pH 7.6), 10 mM MgCl2, 0.1 mM dithiothreitol (DTT), 100 mM NaCl, 1 mM ATP (with 0.025 μCi/μL [γ-32P]ATP), 4 μL of the recombinant protein solution (without purification), and 1 mM guanosine diphosphate (GDP) or triphosphate (GTP). The reaction mixtures were incubated for 1 h at 30 °C and extracted with phenol/chloroform. The mixture was subsequently applied to a thin sheet of poly(ethylenimine) (PEI)–cellulose (Merck, Germany), and the TLC was developed with 1.5 M KH2PO4 solution. The resulting spots for ppGpp and pppGpp were visualized by autoradiography. The authentic [32P]ppGpp and [32P]pppGpp were synthesized according to the method of Sato et al.Citation15)
Microscopic analysis of transiently expressed PpRSH2a-GFP and PpRSH2b-GFP
To observe the localization of the PpRSH2a protein, we cloned PpRSH2a into the gateway vector, pUGWnew5, to generate a recombinant C-terminal GFP (green fluorescent protein) fusion protein. The PCR reaction was performed using KOD-plus, as described above. The primer set used for the PCR amplification was PpRSH2a-NcoIF with an NcoI site (5′-ttccatggtgaggctagcggaa-3′) and PpRSH2a-XhoIR3 with an XhoI site (5′-tatctcgagcacatgagaccagcgac-3′) for PpRSH2a. The resulting DNA fragments were cloned into the pENTR4 entry vector (Invitrogen, USA) to generate pENTRSH2a. The LR reaction was performed according to manufacturer’s protocol using the pENTRSH2a plasmid and the pUGWnew5 destination vector in conjunction with LR clonase®II (Invitrogen, USA) to generate the 35S::PpRSH2a-GFP plasmid (pUGWnew5-PpRSH2a).Citation26) The transformation of P. patens protoplasts with pUGWnew5-PpRSH2a was conducted using poly(ethylene glycol) (PEG)-mediated transformation.Citation27) The localization of the PpRSH2a-GFP protein was analyzed using a TCS-SP5 confocal laser-scanning microscope (Leica Microsystems, Germany). The same procedure was performed to obtain a 35S::PpRSH2b-GFP plasmid (pUGWnew5-PpRSH2b) for the examination of PpRSH2b protein localization, except for the PCR amplification. To clone PpRSH2b into the pUGWnew5 gateway vector, PpRSH2b-NcoIF with an NcoI site (5′-ttccatggtgaggctagcggaa-3′) and PpRSH2b-XhoIR3 with an XhoI site (5′-tatctcgagcacatgagaccagcgac-3′) were used as the primer set for the PCR amplification.
Semi-quantitative RT-PCR of the PpRSHs after treatments with plant hormones and abiotic stresses
P. patens at protonemal stage, which was grown on BCDATG agar medium for 3 days, was used for this experiment. The plants were sprayed with a hormone solution (100 μM JA or ABA) or NaCl (300 mM) or subjected to stress by spraying a solution of mannitol (600 mM) on the protonema tissues. For the UV treatment, the plants were irradiated with UV radiation (254 nm, 614 μW/cm2) for 10 min at a distance of 30 cm. The plants were transferred from the medium onto filter papers for dehydration. Next, cDNA was prepared from the total RNA extracted from the protonema with or without treatments. The detailed semi-quantitative RT-PCR method is described in the supporting information.
Construction of PpRSH2a and PpRSH2b overexpression mutants
We used the DNA fragments of PpRSH2a, obtained as described above, in the construction of the overexpression vector, pTFH9119-PpRSH2a. The vector contains a genomic fragment of the PpMADS2 gene, in the middle of which the neomycin phosphotransferase II expression cassette and the rice actin promoter, and the nos terminator are inserted. Disruptants of the PpMADS2 locus are indistinguishable from wild type.Citation28) After pTFH9119 was digested with SmaI and dephosphorylated, the DNA fragments were cloned into this plasmid to yield pTFH9119-PpRSH2a. P. patens was transformed with the resultant vector, pTHF9119-PpRSH2a, using PEG-mediated method.Citation27) Same procedure was applied to construct PpRSH2b overexpression mutant. The insertion of single copy of the each gene in the PpMADS2 gene was confirmed by PCR. The phenotype of PpRSH2a and PpRSH2b overexpression mutants was observed using MZ6 stereoscopic microscope (Leica, Germany).
Results
Cloning of PpRSH2a and PpRSH2b genes
A search of the genome database for P. patens revealed the presence of nine putative RSH genes. A phylogenetic analysis of the deduced PpRSH proteins showed that all of these homologs, except PpRSH4, were categorized in the AtRSH1, AtRSH2/3, or AtCRSH clusters (Figs. and S1). According to the result, we designated as PpRSH1a (XM_001775612), PpRSH1b (XM_001775529), PpRSH2a (XM_001754893), PpRSH2b (XM_001785010), PpRSH2c (XM_001778435), PpRSH2d (XM_001778437), PpRSH4 (XM_0017548949), PpCRSH1a (XM_001753128), and PpCRSH1b (XM_001773989), respectively. PpCRSH1a and PpCRSH1b were classified in the AtCRSH cluster, a gene that confers the ability to develop flowers; until recently, this cluster had been reported to be occupied only by flowering land plants.Citation13) To determine which PpRSHs were predominantly expressed in P. patens, we performed a semi-quantitative RT-PCR analysis using the cDNA prepared from P. patens at a protonemal stage, which was grown for three days, under normal conditions (Fig. ). We found that PpRSH2a and PpRSH2b were expressed to a much higher degree than the other PpRSH genes. Both PpRSH2a and PpRSH2b were classified in the AtRSH2/3 cluster, a gene that had previously been suggested to be involved predominantly in (p)ppGpp synthesis in flowering plants.Citation11,13) Therefore, it is possible that PpRSH2a and PpRSH2b are involved in (p)ppGpp synthesis in the moss, P. patens. PpRSH2a and PpRSH2b are predicted to consist of 573 and 627 amino acids, respectively, and a sequence alignment of PpRSH2a and PpRSH2b with known plant RSHs revealed that both proteins had a high similarity to other plant RSHs (Fig. ). The HD box of the metallophosphatase function for the degradation of (p)ppGpp and the glycine residue that is essential for (p)ppGpp synthesis were also conserved in PpRSH2a and PpRHS2b, suggesting that these proteins are capable of synthesizing (p)ppGpp.Citation29) Previously studied plant RSH proteins were shown to be translocated to the chloroplasts with the aid of an N-terminal signal peptide.Citation10–13) In contrast, the iPSORT and ChloroP (version 1.1) programs predicted the absence of an N-terminal signal peptide in PpRSH2a and PpRSH2b.
Fig. 1. Phylogenetic tree of RelA/SpoT homologs from flowering plants and P. patens.
Notes: Amino-acid sequences were aligned using Clustal W, and the phylogenetic tree was generated using Treeview software (http://txonomy.zoology.gla.ac.uk/rod/treeview.html). The analysis was performed with the following: AtRSH1, AtRSH2, AtRSH3, and AtCRSH (A. thaliana, NP_849287, NP_188021, NP_564652, and NP_188374, respectively); OsRSH1, OsRSH2, OsRSH3, OsCRSH1, OsCRSH2, and OsCRSH3 (O. sativa, NP_001050067, NP_001061969, AB095097, AB042936, AK058438, and AB298325, respectively); NtRSH1, NtRSH2, and NtRSH4 (N. tabacum, AB095098, AY346377, and AB095099, respectively); and PpRSH1a, PpRSH1b, PpRSH2a, PpRSH2b, PpRSH2c, PpRSH2d, PpRSH4, PpCRSH1a, and PpCRSH1b (P. patens, XP_001775664, XP_001775581, XP_001754945, XP_001785062, XP_001778487, XP_001778489, XP_001754946, XP_001753180, and XP_001774041, respectively).
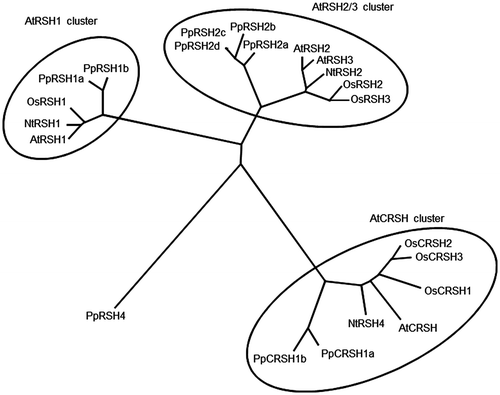
Fig. 2. Expression analysis of PpRSHs.
Notes: The cDNA was prepared from the total RNA extracted from the protonema of P. patens grown on BCDATG agar for 3 days. The PCR was conducted using specific primer sets and the corresponding cDNA as the template. The PCR products were analyzed by gel electrophoresis and visualized by staining with ethidium bromide.
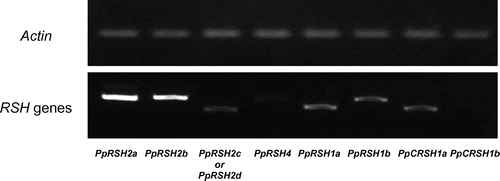
Fig. 3. Amino-acid sequence alignment of the previously reported RelA/SpoT homologs with PpRSH2a and PpRSH2b.
Notes: Amino-acid sequences were aligned using Clustal W. The arrow in the center shows the glycine residue that is essential for (p)ppGpp synthesis. The box indicates the HD box for metallophosphatases. The aligned sequences include PpRSH2a (P. patens, XP_001754945), PpRSH2b (P. patens, XP_001785062), AtRSH2 (A. thaliana, NP_188021), OsRSH2 (O. sativa, BAC81140), NtRSH2 (N. tabacum, AAQ23899), RelA (E. coli, AP_003350), and SpoT (E. coli, AP_004142).
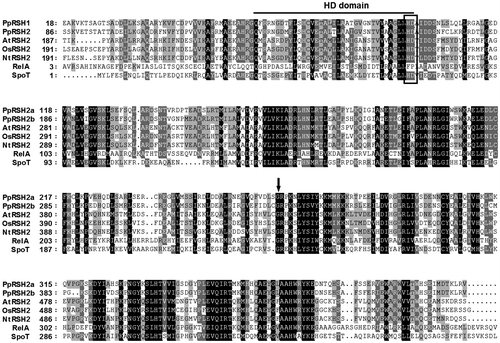
Functional complementation analysis of PpRSH2a and PpRSH2b in E. coli
The RelA/SpoT protein has been characterized as a stringent factor in E. coli. The relA mutant and the relA spoT double mutant both grow as well as the wild-type bacteria does on nutrient-rich medium, but neither of the mutants grow on minimal agar medium (SMG medium) because they lack the ability to synthesize (p)ppGppCitation29); transformation of the bacteria with a gene-encoding (p)ppGpp synthetase restores the growth of the relA-null mutants on SMG mediumCitation30). To examine whether PpRSH2a and PpRSH2b are involved in (p)ppGpp synthesis, we used these E. coli mutants in complementation experiments. We cloned the cDNA encoding PpRSH2a and PpRSH2b into the pET21d expression vector to yield pETRSH2a and pETRSH2b. The E. coli strains, CF1652 (λDE3, relA), CF1678 (λDE3, relA spoT), and W3110 (λDE3, wild-type), were prepared by the lysogenization of λDE3 for the IPTG control followed by transformation with the pETRSH2a, pETRSH2b, or pET21d plasmid. Strikingly, both PpRSH2a and PpRSH2b compensated for the deficient growth of strain CF1652 (relA) (Fig. ). Thus, PpRSH2a and PpRSH2b functioned as a ppGpp synthase. However, complementation in strain CF1678 (relA spoT) was detected only with PpRSH2b because of the unavailability of a stable PpRSH2a transformant (Fig. S2).
Fig. 4. Complementation analysis of PpRSH2a and PpRSH2b in E. coli.
Notes: E. coli strains, CF1652 (λDE3, relA), W3110 (λDE3, wild-type), were transformed with pETRSH2a (encoding PpRSH2a), pETRSH2b (encoding PpRSH2b), or the empty vector (pET21d). Each transformed mutant was incubated on SMG agar medium in the presence or absence of 50 μM IPTG at 37 °C for 60 h.
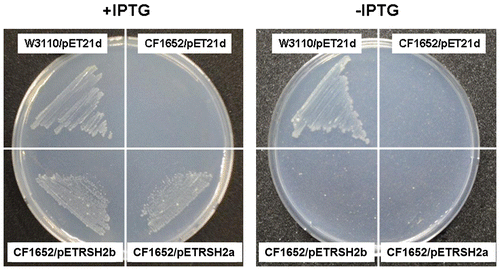
In vitro (p)ppGpp synthesis by recombinant PpRSH2a and PpRSH2b
To confirm the (p)ppGpp synthetase and hydrolase activities of PpRSH2a and PpRSH2b, we performed in vitro enzymatic reactions using cell-free-synthesized proteins incubated with [γ-32P]ATP and GTP or GDP at 30 °C and analyzed by PEI-cellulose TLC (Fig. ). The reaction mixtures of PpRSH2a and PpRSH2b synthesis were directly used for (p)ppGpp synthesis because the expression of these proteins was low in the cell-free protein system used (Fig. S3). As expected, [32P]-labeled ppGpp or pppGpp spots were detected in all of the lanes, thereby demonstrating unambiguously that both of the PpRSH proteins possess (p)ppGpp synthetic activity. The SpoT protein catalyzes the Mn2+-dependent degradation of ppGpp to GDP and PPi in E. coli.Citation31) Therefore, we examined the hydrolase activity of PpRSH2a and PpRSH2b by TLC analysis of the reaction mixtures containing [32P]-labeled ppGpp and PpRSH2a or PpRSH2b. However, no hydrolase activity of these PpRSHs was detected under the conditions of our study (data not shown).
Fig. 5. In vitro (p)ppGpp synthesis by recombinant PpRSH2a and PpRSH2b proteins.
Notes: Recombinant PpRSH2a and PpRSH2b proteins were prepared using a cell-free protein synthesis system. After reaction of PpRSH2a or PpRSH2b in the presence of 1 mM ATP (with 0.025 μCi/μL [γ-32P]ATP) and 1 mM GDP or GTP, a portion of the reaction mixture was applied to a PEI-cellulose TLC plate, which was developed with 1.5 M aqueous KH2PO4. The [32P]-labeled (p)ppGpp was visualized by autoradiography. A solution of cell-free protein synthesis system with empty pTD1 vector, which was applied to assay for (p)ppGpp synthetic activity, was used for control. The mixture of [32P]-labeled (p)ppGpp synthesized by pyrophosphate transferase of Streptomyces morookaensis was applied to same TLC as standard.
![Fig. 5. In vitro (p)ppGpp synthesis by recombinant PpRSH2a and PpRSH2b proteins.Notes: Recombinant PpRSH2a and PpRSH2b proteins were prepared using a cell-free protein synthesis system. After reaction of PpRSH2a or PpRSH2b in the presence of 1 mM ATP (with 0.025 μCi/μL [γ-32P]ATP) and 1 mM GDP or GTP, a portion of the reaction mixture was applied to a PEI-cellulose TLC plate, which was developed with 1.5 M aqueous KH2PO4. The [32P]-labeled (p)ppGpp was visualized by autoradiography. A solution of cell-free protein synthesis system with empty pTD1 vector, which was applied to assay for (p)ppGpp synthetic activity, was used for control. The mixture of [32P]-labeled (p)ppGpp synthesized by pyrophosphate transferase of Streptomyces morookaensis was applied to same TLC as standard.](/cms/asset/b12f27ce-f675-4e19-bd0d-c62f9374bc76/tbbb_a_952617_f0005_b.gif)
Cellular localization of PpRSH2a and PpRSH2b
A number of plant RSH proteins have been reported to localize to the chloroplasts.Citation10–13) However, the iPSORT and ChloroP (version 1.1) programs suggested that the N-terminal signal peptide is absent in PpRSH2a and PpRSH2b. Therefore, we examined the localization of PpRSH2a and PpRSH2b in P. patens protoplasts. The GFP plasmids, pUGWnew5-PpRSH2a and pUGWnew5-PpRSH2b, were constructed, transformed into the protoplasts, and examined under a confocal laser-scanning microscope. The presence of GFP was readily detected in the chloroplasts and could be distinguished from the red fluorescence of chlorophyll (Fig. ). These results clearly demonstrated that PpRSH2a and PpRSH2b were localized in the chloroplasts, in keeping with the localization of other plant RSH proteins.
Fig. 6. Expression of PpRSH2a-GFP fusion protein or PpRSHb2-GFP fusion protein in the chloroplasts of P. patens protoplasts.
Notes: (A) Fluorography of PpRSH2a-GFP protein in a protoplast of P. patens. (B) Fluorography of PpRSH2b-GFP protein in a plotoplast of P. patens. Each RSH protein-GFP fusion construct was introduced into P. patens protoplasts by PEG-mediated transformation. Images were taken with a confocal laser-scanning microscope with excitation at 488 nm and emission at 530 nm for the detection of the GFP signal and an emission above 655 nm for the detection of autofluorescence from chlorophyll. Scale bar: 10 μm.
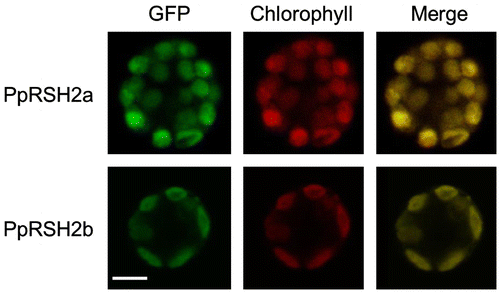
Expression analysis of PpRSH genes under treatment with plant hormones or abiotic stresses
To evaluate the effect of environmental stresses, JA, and ABA on PpRSH expression in P. patens at the transcriptional level, we performed a semi-quantitative RT-PCR analysis. We observed that the expression of the nine PpRSH genes was transiently induced by the treatment with ABA (Fig. ). Moreover, environmental stresses, such as osmotic stress, dehydration, and UV irradiation, also transiently induced the expression of some PpRSH genes. Intriguingly, dehydration treatment induced the continuous expression of PpRSH2a and PpRSH2b. In contrast, unlike flowering plants, treatment with JA or high concentrations of salt failed to induce the expression of the PpRSH genes (Fig. S4). These results suggested that (p)ppGpp signaling via ABA and environmental stresses appears to be mostly conserved in P. patens.
Fig. 7. Expression analysis of PpRSHs in P. patens.
Notes: The cDNA was prepared from the total RNA extracted from the protonema of P. patens grown on BCDATG agar with or without treatment of ABA, osmotic stress, UV irradiation, or dehydration. The PCR was conducted using each cDNA as the template. The PCR products were analyzed by gel electrophoresis and were visualized by staining with ethidium bromide.
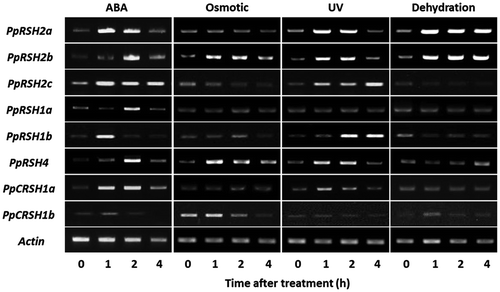
Phenotype analysis of the mutants overexpressing PpRSH2a and PpRSH2b
To examine the physiological significance of the functions of PpRSH2a and PpRSH2b, we constructed each of the transgenic P. patens mutants, each that overexpressed PpRSH2a and PpRSH2b. When these strains were grown on BCDAT or BCDATG [being supplemented with 0.5% glucose (w/v)] agar, little difference in the phenotype between the mutants and wild-type moss was observed (data not shown). However, the mutants on BCDAT agar supplemented with 1% glucose (w/v) showed reduction of the growth in comparison with the wild type grown on the same medium (Fig. ). These results indicated that overexpression of PpRSH2a and PpRSH2b caused suppression of the growth in response to adequate glucose concentration (1%) in P. patens.
Fig. 8. The phenotype of the P. patens mutants overexpressing PpRSH2a and PpRSH2b.
Notes: (a) The mutants and wild-type P. patens were grown at 25 °C on BCDAT agar supplemented with 1% glucose (w/v) for 20 days under continuous white fluorescent light. Scale bar: 5 mm. (b) Diameter of the each plant colony. Data are means ± SD (n = 6). Asterisks denote a significant difference between wild type (WT) and the mutants in a data pair (Student’s t-test, *p < 0.05, **p < 0.01).
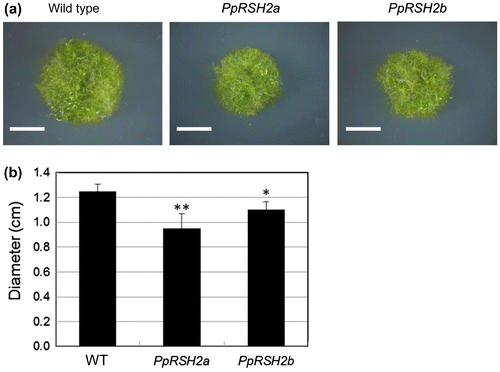
Discussion
In this study, we found nine putative RSH genes in the genome of the model moss, P. patens. Eight of the nine putative RSH proteins in P. patens were categorized in the clusters of AtRSH1, AtRSH2/3, and AtCRSH (Fig. ); notably, all of the RSH proteins in flowering plants are grouped into these clusters. Considering our database analysis of the P. patens genome, the RSH genes in these three groups were probably highly conserved in plants. PpRSH4, which is not categorized in those three clusters, is indicated to have important amino acids for ppGpp synthesis and hydrolysis. It is likely that PpRSH4 functions as ppGpp synthase and hydrolase (Fig. S1).
As in flowering plants, the expression of the PpRSHs in P. patens was transiently induced by treatments with ABA, osmotic stress, dehydration, and UV irradiation. Moreover, dehydration resulted in the constitutive expression of PpRSH2a and PpRSH2b (Fig. ). Unlike flowering plants, the treatment with JA and high concentrations of salt failed to induce the expression of the PpRSH genes; however, the presence of JA and its signal pathway has not been confirmed in P. patens. ABA is indicated to be an important plant hormone with a significant role in (p)ppGpp signaling in plants.Citation14) It is probable that the responsiveness of RSH gene expression caused by ABA is conserved in land plants.
To elucidate the function of the RSH genes in P. patens, we cloned PpRSH2a and PpRSH2b, which were predominantly expressed under conventional growth conditions and were induced by environmental cues and ABA treatment. The expression of PpRSH2a and PpRSH2b complemented the growth of the E. coli relA mutant, which lacks (p)ppGpp synthetic activity, showing that both PpRSH2a and PpRSH2b can function as ppGpp synthases (Fig. ). This result is consistent with the conserved peptide sequence of the RelA domain in PpRSH2a and PpRSH2b, although only PpRSH2b compensated for the growth of the relA spoT double mutant (Fig. S2). The inability to obtain a stable E. coli (relA spoT) mutant complemented with PpRSH2a might have resulted from increased (p)ppGpp synthesis activity and/or decreased (p)ppGpp hydrolytic activity, as the inability to turn over (p)ppGpp would give rise to harmful effects on growth and viability of E. coli. This possibility is supported by the growth recovery of the CF1652 (relA) strain with PpRSH2a. Furthermore, we have shown that the recombinant PpRSH2a and PpRSH2b prepared by an insect cell-free protein synthesis system, in fact, had ppGpp synthetic activity (Fig. ). In E. coli, the spoT gene, which is involved in ppGpp degradation, is necessary for bacterial growth when the relA gene, which contributes solely to ppGpp synthesis, functions properly. Because the expression of PpRSH2b complemented the growth in the relA spoT double mutant of E. coli, PpRSH2b appears to function both as a ppGpp hydrolase and a ppGpp synthase (Fig. S2).
The analysis of the transiently expressed PpRSH2a-GFP and PpRSH2b-GFP fusion proteins by microscopy showed that these proteins were localized in the chloroplasts of P. patens (Fig. ), in accordance with the chloroplast localization of the other proteins grouped in the AtRSH2/3 cluster. Yet, the ChloroP and iPSORT programs predicted the absence of the chloroplast transit peptide at the N-terminus of PpRSH2a and PpRSH2b. However, it has also been reported that allene oxide cyclases (PpAOCs) in P. patens, which have been predicted to lack a signal peptide, are imported into chloroplasts.Citation32,33) Those programs are trained with vascular plant sequences,Citation34) and then might not be suitable to predict the protein localization in chloroplasts of P. patens. Taken together, these data suggest the presence of a specific mechanism to recognize the signal peptides for the transport into chloroplasts in P. patens.
Our phenotypic analysis of the each overexpression mutant of PpRSH2a and PpRSH2b revealed that the growth was suppressed when the mutants were grown on the BCDAT medium supplemented with 1% glucose (Fig. ). However, the mutants grown on BCDAT and BCDATG (BCDAT with 0.5% glucose) medium did not show significant difference from the wild type (data not shown). Sufficient glucose concentration (1% w/v) was shown to inhibit the growth of mutants overexpressing PpRSH2a and PpRSH2b, respectively. Given that the PpRSH proteins and ppGpp are localized in the chloroplasts, the PpRSH-mediated synthesis of ppGpp might be responsible for the regulation of chloroplast functions. Because ppGpp has been shown to inhibit PEP, plastid-encoded plastid RNA polymerase (bacteria-type RNA polymerase),Citation15) peptide elongation cycle of chloroplast translation in vitro,Citation17) guanylate kinase in chloroplasts.Citation18) On the other hand, it has been reported that the expression of photosynthetic genes was suppressed by soluble sugars, which is a situation that would eventually depress photosynthesis in flowering plants.Citation35,36) It is highly likely that an additional factor recruited in response to sufficient dose of glucose suppresses a chloroplast function in cooperation with the ppGpp in P. patens. Therefore, suppression of a function in chloroplasts might cause the growth inhibition of the mutants overexpressing PpRSH2a and PpRSH2b, respectively.
In summary, our study indicated that the expression of PpRSHs was induced by ABA treatment and environmental stresses. The overexpression of PpRSH2a and PpRSH2b, respectively, causes the inhibition of growth in response to glucose (1% w/v) in P. patens. However, the detailed function of the RSH genes remains elusive in P. patens. Given that PpRSH2a and PpRSH2b transported to chloroplasts causes inhibition of the growth of P. patens, a regulatory mechanism for chloroplast functions governed by ppGpp, which is synthesized by PpRSHs, should exist in P. patens.
Supplemental material
The supplemental material for this paper is available at http://dx.doi.org/10.1080/09168451.2014.952617.
Supplemental Materials
Download MS Word (816.5 KB)Acknowledgments
We are grateful to Professor Hasebe of the National Institute for Basic Biology for supplying the pUGWnew5 and pTFH9119 vectors. M.S. is a recipient of JSPS (Japan Society for the Promotion of Science) fellowship. This work was supported by Akiyama Life Science Foundation to K.T.
Notes
Abbreviations: ppGpp, guanosine 5′-diphosphate 3′-diphospahte; pppGpp, guanosine 5′-triphosphate 3′-diphosphate; (p)ppGpp, ppGpp and pppGpp; RSH, RelA/SpoT homolog; CRSH, calcium-activated RelA/SpoT homolog; ABA, abscisic acid; JA, jasmonic acid; TLC, thin layer chromatography.
References
- Cashel M, Gentry DR, Hernandez VJ, Vinella D. The stringent response. In: Neidhardt FC, Curtiss R III, Ingraham JL, Lin ECC, Low KB, Low B, Reznikoff WS, Riley M, Schaechter M, Umbarger HE, editors. Escherichia coli and Salmonella: cellular and molecular biology. 2nd ed. Washington, DC: AMS Press; 1996. p. 1458–1496.
- Potrykus K, Cashel M. (p)ppGpp: still magical? Ann. Rev. Microbiol. 2008;62:35–51.10.1146/annurev.micro.62.081307.162903
- Spira B, Silberstein N, Yagil E. Guanosine 3′,5′-bispyrophosphate (ppGpp) synthesis in cells of Escherichia coli starved for Pi. J. Bacteriol. 1995;177:4053–4058.
- Magnusson LU, Farewell A, Nyström T. ppGpp: a global regulator in Escherichia coli. Trends Microbiol. 2005;13:236–242.10.1016/j.tim.2005.03.008
- Vinella D, Albrecht C, Cashel M, D’Ari R. Iron limitation induces SpoT-dependent accumulation of ppGpp in Escherichia coli. Mol. Microbiol. 2005;56:958–970.10.1111/j.1365-2958.2005.04601.x
- Battesti A, Bouveret E. Acyl carrier protein/SpoT interaction, the switch linking SpoT-dependent stress response to fatty acid metabolism. Mol. Microbiol. 2006;62:1048–1063.10.1111/mmi.2006.62.issue-4
- Lemos JA, Lin VK, Nascimento MM, Abranches J, Burne RA. Three gene products govern (p)ppGpp production by Streptococcus mutans. Mol. Microbiol. 2007;65:1568–1581.10.1111/mmi.2007.65.issue-6
- Nanamiya H, Kasai K, Nozawa A, Yun CS, Narisawa T, Murakami K, Natori Y, Kawamura F, Tozawa Y. Identification and functional analysis of novel (p)ppGpp synthetase genes in Bacillus subtilis. Mol. Microbiol. 2008;67:291–304.
- van der Biezen EA, Sun J, Coleman MJ, Bibb MJ, Jones JD. Arabidopsis RelA/SpoT homologs implicate (p)ppGpp in plant signaling. Proc. Natl. Acad. Sci. 2000;97:3747–3752.10.1073/pnas.97.7.3747
- Kasai K, Usami S, Yamada T, Endo Y, Ochi K, Tozawa Y. A RelA-SpoT homolog (Cr-RSH) identified in Chlamydomonas reinhardtii generates stringent factor in vivo and localizes to chloroplasts in vitro. Nucleic Acids Res. 2002;30:4985–4992.10.1093/nar/gkf628
- Givens RM, Lin MH, Taylor DJ, Mechold U, Berry JO, Hernandez VJ. Inducible expression, enzymatic activity, and origin of higher plant homologues of bacterial RelA/SpoT stress proteins in Nicotiana tabacum. J. Biol. Chem. 2004;279:7495–7504.10.1074/jbc.M311573200
- Tozawa Y, Nozawa A, Kanno T, Narisawa T, Masuda S, Kasai K, Nanamiya H. Calcium-activated (p)ppGpp synthetase in chloroplasts of land plants. J. Biol. Chem. 2007;282:35536–35545.10.1074/jbc.M703820200
- Masuda S, Mizusawa K, Narisawa T, Tozawa Y, Ohta H, Takamiya K. The bacterial stringent response, conserved in chloroplasts, controls plant fertilization. Plant Cell Physiol. 2008;49:135–141.
- Takahashi K, Kasai K, Ochi K. Identification of the bacterial alarmone guanosine 5′-diphosphate 3′-diphosphate (ppGpp) in plants. Proc. Natl. Acad. Sci. USA. 2004;101:4320–4324.10.1073/pnas.0308555101
- Sato M, Takahashi K, Ochiai Y, Hosaka T, Ochi K, Nabeta K. Bacterial alarmone, guanosine 5′-diphosphate 3′-diphosphate (ppGpp), predominantly binds β′ subunit of plastid-encoded plastid RNA polymerase in chloroplasts. Chembiochem. 2009;10:1227–1233.10.1002/cbic.v10:7
- Tozawa Y, Nomura Y. Signalling by the global regulatory molecule ppGpp in bacteria and chloroplasts of land plants. Plant Biol. 2011;13:699–709.10.1111/plb.2011.13.issue-5
- Nomura Y, Takabayashi T, Kuroda H, Yukawa Y, Sattasuk K, Akita M, Nozawa A, Tozawa Y. ppGpp inhibits peptide elongation cycle of chloroplast translation system in vitro. Plant Mol. Biol. 2012;78:185–196.10.1007/s11103-011-9858-x
- Nomura Y, Izumi A, Fukunaga Y, Kusumi K, Iba K, Watanabe S, Nakahira Y, Weber AP, Nozawa A, Tozawa Y. Diversity in guanosine 3′,5′-bisdiphosphate (ppGpp) sensitivity among guanylate kinases of bacteria and plants. J. Biol. Chem. 2014; 289:15631–15641.
- Ito D, Kato T, Maruta T, Tamoi M, Yoshimura K, Shigeoka S. Enzymatic and Molecular Characterization of Arabidopsis ppGpp Pyrophosphohydrolase, AtNUDX26. Biosci. Biotechnol. Biochem. 2012;76:2236–2241.10.1271/bbb.120523
- Lang D, Zimmer AD, Rensing SA, Reski R. Exploring plant biodiversity: the Physcomitrella genome and beyond. Trends Plant Sci. 2008;13:542–549.10.1016/j.tplants.2008.07.002
- Schaefer DG. A new moss genetics: targeted mutagenesis in Physcomitrella patens. Ann. Rev. Plant Biol. 2002;53:477–501.10.1146/annurev.arplant.53.100301.135202
- Lang D, Zimmer AD, Rensing SA, Reski R. Exploring plant biodiversity: the Physcomitrella genome and beyond. Trends Plant Sci. 2008;13:542–549.10.1016/j.tplants.2008.07.002
- Ashton NW, Cove DJ. The isolation and preliminary characterisation of auxotrophic and analogue resistant mutants of the moss, Physcomitreila patens. Mol. Gen. Genet. 1977;154:87–95.10.1007/BF00265581
- Nishiyama T, Hiwatashi Y, Sakakibara K, Kato M, Hasebe M. Tagged mutagenesis and gene-trap in the moss, Physcomitrella patens by shuttle mutagenesis. DNA Res. 2000;7:9–17.10.1093/dnares/7.1.9
- Neidhardt FC, Bloch PL, Smith DF. Culture medium for enterobacteria. J. Bacteriol. 1974;119:736–747.
- Nakagawa T, Ishiguro S, Kimura T. Gateway vectors for plant transformation. Plant Biotechnol. 2009;26:275–284.10.5511/plantbiotechnology.26.275
- Baur A, Reski R, Gorr G. Enhanced recovery of a secreted recombinant human growth factor using stabilizing additives and by co-expression of human serum albumin in the moss Physcomitrella patens. Plant Biotechnol. J. 2005;3:331–340.10.1111/pbi.2005.3.issue-3
- Imaizumi T, Kadota A, Hasebe M, Wada M. Cryptochrome light signals control development to suppress auxin sensitivity in the moss Physcomitrella patens. Plant Cell. 2002;14:373–386.10.1105/tpc.010388
- Hogg T, Mechold U, Malke H, Cashel M, Hilgenfeld R. Conformational antagonism between opposing active sites in a bifunctional RelA/SpoT homolog modulates (p)ppGpp metabolism during the stringent response. Cell. 2004;117:57–68.10.1016/S0092-8674(04)00260-0
- Uzan M, Danchib A. A rapid test for the relA mutation in E. coli. Biochem. Biophys. Res. Commun. 1976;69:751–758.10.1016/0006-291X(76)90939-6
- Heinemeyer EA, Geis M, Richter D. Degradation of guanosine 3′-diphosphate 5′-diphosphate in vitro by the spoT gene product of Escherichia coli. Eur. J. Biochem. 1978;89:125–131.10.1111/ejb.1978.89.issue-1
- Stumpe M, Göbel C, Faltin B, Beike AK, Hause B, Himmelsbach K, Bode J, Kramell R, Wasternack C, Frank W, Reski R, Feussner I. The moss Physcomitrella patens contains cyclopentenones but no jasmonates: mutations in allene oxide cyclase lead to reduced fertility and altered sporophyte morphology. New Phytol. 2010;188:740–749.10.1111/j.1469-8137.2010.03406.x
- Hashimoto T, Takahashi K, Sato M. Cloning and characterization of an allene oxide cyclase (PpAOC3) in Physcomitrella patens. Plant Growth Regul. 2011;65:239–243.10.1007/s10725-011-9592-z
- Emanuelsson O, Brunak S, von Heijne G, Nielsen H. Locating proteins in the cell using TargetP, SignalP, and related tools. Nat. Protoc. 2007;2:953–971.10.1038/nprot.2007.131
- Sheen J. Metabolic repression of transcription in higher plants. Plant Cell. 1990;2:1027–1038.10.1105/tpc.2.10.1027
- Pego JV, Kortstee AJ, Hujiser C, Smeekens S. Photosynthesis, sugar and the regulation of gene expression. J. Exp. Bot. 2000;51:407–416.10.1093/jexbot/51.suppl_1.407