Abstract
Dietary plant protein is well known to reduce serum cholesterol levels. Rice bran is a by-product of rice milling and is a good source of protein. The present study examined whether feeding rats a high-cholesterol diet containing 10% rice bran protein (RBP) for 10 d affected cholesterol metabolism. Rats fed dietary RBP had lower serum total cholesterol levels and increased excretion of fecal steroids, such as cholesterol and bile acids, than those fed dietary casein. In vitro assays showed that RBP strongly bound to taurocholate, and inhibited the micellar solubility of cholesterol, compared with casein. Moreover, the bile acid-binding proteins of the RBP were eluted by a chromatographic column conjugated with cholic acid, and one of them was identified as hypothetical protein OsJ_13801 (NCBI accession No. EAZ29742) using MALDI-TOF mass spectrometry analysis. These results suggest that the hypocholesterolemic action of the RBP may be caused by the bile acid-binding proteins.
Graphical Abstract
The bile acid-binding proteins (e.g. hypothetical protein OsJ_13801) of rice bran protein express the hypocholesterolemic action via inhibiting the micellar solubility of cholesterol in the intestine.
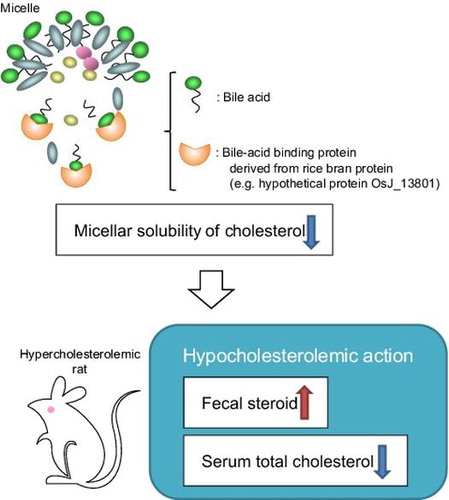
Key words:
Bile acid sequestrants (e.g. cholestyramine and colestipol) are drugs that are capable of binding to bile acids in the intestine and have been developed as a strategy for treating hypercholesterolemia, which is a major risk factor for atherosclerosis. As the drugs bind to bile acids, they form insoluble complexes which are excreted in the feces. Excretion of the insoluble complexes leads to the conversion of cholesterol to bile acids in the liver to compensate for the excreted bile acids. The decrease in hepatic cholesterol followed by the cholesterol to bile acids conversion enhances the low-density lipoprotein (LDL) receptor expression, which subsequently lowers the serum LDL-cholesterol level.Citation1)
Dietary plant protein (e.g. from soy and rice) is well known to lower serum cholesterol levels, including the LDL-cholesterol level, more than dietary animal protein.Citation2) Rice is one of the primary cereals consumed worldwide, and milled rice (also called white rice) is the principal staple food for a large number of people. Some studies have reported that dietary white rice protein, as well as dietary soy protein, reduces serum cholesterol concentrations and also promotes the excretion of fecal steroids such as cholesterol and bile acids,Citation3–6) probably indicating that these dietary proteins lead to both inhibited absorption of dietary and biliary cholesterols and reabsorption of bile acids in the intestine. Some of our previous research have shown that dietary soy protein hydrolysates and cholestyramine strongly bind to bile acids and decrease the micellar solubility of cholesterol in vitro which is a key step to dietary cholesterol absorption in the small intestine, and the hydrolysates repress cholesterol absorption in rat intestines.Citation7,8) Therefore, it is likely that foods and drugs capable of binding to bile acids reduce dietary cholesterol absorption by inhibiting the micellar solubility of cholesterol.
In contrast to the findings for white rice protein and soy protein, it is unclear whether rice bran protein (RBP) affects cholesterol metabolism. Although rice bran is produced as a by-product of rice milling, it contains 10–16% highly nutritional protein.Citation9) In addition, RBP has been recognized as nutritionally superior to other proteins, especially on its reported hypoallergenicityCitation10) and anticancer activity.Citation11) Thus, the studies on RBP indicate that it has nutritional and pharmaceutical potential. Only a little information is available on the relationship between RBP and cholesterol absorption. Zhan et al. showed that RBP hydrolysate by a pancreatic peptidase trypsin inhibited micellar solubility of cholesterol in vitro without animal experiments.Citation12) Thus, until now, the mechanism of hypocholesterolemic action of RBP in vivo is unclear. Considering that foods and drugs capable of binding to bile acids in the intestine could inhibit the micellar solubility of cholesterol, we hypothesized that RBP, including the hydrolysates, also might act as a bile acid sequestrant, resulting in a decrease in serum cholesterol levels via repression of cholesterol absorption accompanied by inhibition of micellar solubility of cholesterol in the intestine in vivo.
In this study, we examined the effects of RBP on cholesterol metabolism in rats, the bile acid-binding capacity of RBP, and the effect of RBP on micellar solubility of cholesterol in vitro. Moreover, we performed the elution of bile acid-binding proteins from RBP by bile acid-conjugated affinity chromatography and the identification of the bile acid-binding protein by MALDI-TOF mass spectrometry.
Materials and methods
Animals and diets
Five-week-old male Wistar rats weighing 90–110 g (Japan SLC) were housed individually in an air conditioned room (22 °C ± 2 °C) under a 12-h light/dark cycle (lights on: 08:00–20:00). After acclimation to a standard laboratory chow (MF; Oriental Yeast, Tokyo, Japan) for 3 d, the rats were divided into two groups: casein and RBP. The casein group received a high-cholesterol diet containing 20% casein (Meiji, Tokyo, Japan) as net amount of protein (casein diet). The RBP group received a high-cholesterol diet containing 10% casein (Meiji) and 10% RBP (Oriza Oil and Fat Chemical, Aichi, Japan) as net amount of protein (RBP diet). Casein consisted of 87.5% protein, 0.0% sugar, 1.0% lipids, 0.0% dietary fiber, 1.8% ash, and 9.7% moisture, and RBP consisted of 81.5% protein, 4.9% sugar, 0.5% lipids, 5.6% dietary fiber, 4.1% ash, and 3.4% moisture. The details of the diet compositions and the amino acid compositions of the dietary proteins were shown in Tables and . The animals were allowed free access to food and water for 10 d. Feces were collected for 3 d (day 6–8) before the animals were sacrificed. At the end of the feeding, the rats were killed under diethyl ether anesthesia by withdrawing blood from the heart after 18 h starvation. The serum obtained was frozen at −20 °C until analysis. The Gifu University Animal Care and Usage Committee approved this experiment.
Table 1. Compositions of casein and RBP diets.
Table 2. Amino acid compositions of casein and RBP.
Serum and fecal lipid assays
The serum total cholesterol concentration was enzymatically measured with a commercial assay kit (Cholesterol E-test Wako; Wako Pure Chemical, Osaka, Japan). Fecal acidic steroids were measured according to the methods reported by Bruusgaard et al.Citation13) and Malchow-Moller et al.Citation14) and fecal neutral steroids were assayed with trimethylsilyl ether by using 1.5% OV-17 with a GC-14A instrument (Shimadzu, Kyoto, Japan) and 5 α-cholestane as the internal standard.Citation15)
Taurocholate binding capacity assay
The abilities of casein and RBP to bind with taurocholate were measured with a method described previously.Citation8) Mixtures containing 1.85 kBq of tauro [carbonyl-14C] cholic acid (sodium salt) (PerkinElmer Life Sciences, MA, USA), 0.1 mol/L sodium taurocholate in 2 mL of 0.1 mol/L Tris–HCl buffer (pH 7.4), and binding substance (casein sodium (Wako Pure Chemical) or RBP (Oriza Oil and Fat Chemical), 100 g/L) were incubated at 37 °C for 2 h, and the radioactivity in the supernatant (15,000 × g for 15 min) was measured by liquid scintillation counting.
Assay for micellar solubility of cholesterol
The micellar solubility of cholesterol with casein and RBP in vitro were measured using a previously described method.Citation8) [14C]-labeled micellar solutions (1.0 mL) were prepared at the following concentrations and mixed by sonication: 0.74 kBq [4-14C]-cholesterol (2.1 Gbq/mmol, PerkinElmer Life Sciences), 0.1 mM cholesterol (Katayama Chemical, Osaka, Japan), 6.6 mM sodium taurocholate (Sigma–Aldrich, Tokyo, Japan), 1 mM oleic acid (Sigma–Aldrich), 0.6 mM phosphatidylcholine (PC) (Sigma–Aldrich), 0.5 mM monoolein (Sigma Aldrich), and 15 mM sodium phosphate (pH 7.4). After incubation at 37 °C for 24 h, casein sodium (Wako Pure Chemical) or RBP (Oriza Oil and Fat Chemical) (10 g/L) was added to the micellar solution, dispersed by sonication, incubated at 37 °C for 1 h, and centrifuged at 100,000 × g for 1 h at 37 °C. The supernatant was collected for determination of [14C]-cholesterol by a liquid scintillation counter.
Elution of bile acid binding proteins from RBP by cholic acid-conjugated EAH Sepharose 4B column chromatography
Sodium cholate hydrate (Sigma–Aldrich) was coupled to EAH Sepharose 4B (GE Healthcare, Tokyo, Japan) using 1-ethyl-3-(3-dimethylaminopropyl) carbodiimide (Dojindo Laboratories, Kumamoto, Japan)-HCl (pH 6.4) according to the procedure described by Pattinson et al.Citation16) RBP was dissolved in 0.02% NaN3 containing 10 mM Tris–HCl (pH 8.0). The RBP solution was filtered by membrane filters with a 0.20 μm pore size (Advantec, Tokyo, Japan). Twenty-five milligrams protein from the filtrate was applied to the cholic acid-conjugated EAH Sepharose 4B column, and elution was carried out according to the procedure described by Makino et al.Citation17) After elution of the filtrate, the column was washed with 0.5 M NaCl containing 10 mM Tris–HCl (pH 8.0), and the specifically bound proteins (bile acid-binding proteins) were eluted with 0.5% sodium deoxycholate containing 10 mM Tris–HCl (pH 8.0). Non-specifically bound proteins that remained in the column were eluted by 8 M urea containing 10 mM Tris–HCl (pH 8.0). During chromatography, the flow rate was 0.5 mL/min and the fraction volume was 10 mL. Protein elution profiles were monitored at 280 nm. HPLC was carried out using an AKTA prime plus system (GE Healthcare). The eluted protein was freeze dried and preserved at −20 °C until analysis.
SDS-PAGE and MALDI-TOF mass spectrometry
The RBP sample, which contained bile acid-binding proteins eluted via a cholic acid-conjugated EAH Sepharose 4B column, was separated by SDS-PAGE. The electrophoresed gel was stained with CBB-G250. A protein band, calculated to be about 55.1 kDa based on relative mobility, of the separated RBP sample was excised and digested by trypsin. The digest was desalted and concentrated with ZipTip μ-C18 (Merk Millipore, Tokyo, Japan) and analyzed by MALDI-TOF mass spectrometry. The spectral data were compared with data annotated in the NCBI database using MASCOT software.
Statistical analysis
Each value has been expressed as the mean ± SEM. Significance between two groups was evaluated by Student’s t-test. p < 0.05 was considered to indicate statistical significance.
Results
The effects of dietary RBP on metabolic parameters in rats fed a hypercholesterolemic diet
Body weight gain tended to be lower (p = 0.0641, analyzed by Student’s t-test) in the RBP-fed group than in the casein-fed group. No significant changes were found in the food intake and liver weight (p = 0.9757 and 0.1901, respectively, analyzed by Student’s t-test) for the casein- and RBP-fed groups. Serum total cholesterol concentration in the RBP-fed group was significantly lower (45% lower) than that in the casein-fed group. Fecal dry weight tended to be higher (p = 0.0646, analyzed by Student’s t-test), and neutral and acid steroid levels in the feces were significantly higher (2.0- and 1.4-fold higher, respectively) in the RBP-fed group than in the casein-fed group (Table ).
Table 3. The effects of dietary RBP on metabolic parameters in rats fed a hypercholesterolemic diet.
The taurocholate-binding capacity of RBP and the effect of RBP on micellar solubility of cholesterol in vitro
The taurocholate-binding capacity of RBP was significantly higher (3.6-fold higher) than that of casein sodium (Fig. (A)). The micellar solubility of cholesterol in the presence of RBP was significantly lower (84% lower) than that in the presence of casein sodium (Fig. (B)).
Fig. 1. The taurocholate-binding capacity of RBP and the effect of RBP on micellar solubility of cholesterol in vitro.
Notes: (A) The taurocholate-binding capacity of RBP in vitro. Values are expressed as means ± SEM (n = 3). ** p < 0.01; significantly different from casein (Student’s t-test). (B) The effect of RBP on micellar solubility of cholesterol in vitro. Values are expressed as means ± SEM (n = 4). ** p < 0.01; significantly different from casein (Student’s t-test).
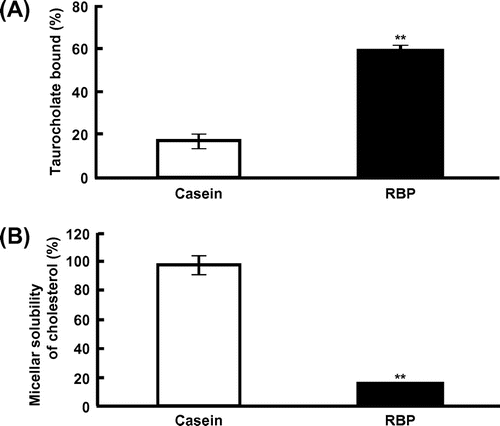
The elution of bile acid-binding proteins from RBP by a chromatographic column conjugated with cholic acid
Because it has been reported that bovine serum albumin (BSA) has bile acid-binding capacity but that ovalbumin does not,Citation17) we first verified that BSA bound to a cholic acid-conjugated EAH Sepharose 4B column and that ovalbumin did not (data not shown). Bile acid-binding proteins from RBP were eluted using a cholic acid-conjugated EAH Sepharose 4B column. The typical elution profile of RBP is shown in Fig. (A). A mixture of the three protein fractions (No. 11–13) that bound to the cholic acid-conjugated EAH Sepharose 4B column was separated by SDS-PAGE. As shown in Fig. (B), some bile acid-binding protein bands were observed. A protein band, calculated to be about 55.1 kDa, was excised, treated with trypsin, and subjected to MALDI-TOF mass spectrometry analysis. The hypothetical protein OsJ_13801 (NCBI accession No. EAZ29742; 54.5 kDa) was identified as a bile acid-binding protein. The matched peptides showed high sequence homology (sequence coverage, 45%) with the hypothetical protein OsJ_13801 (Fig. (C)).
Fig. 2. The elution of bile acid-binding proteins from RBP by a chromatographic column conjugated with cholic acid.
Notes: (A) Affinity chromatography of RBP. RBP solution containing 25 mg protein in 0.02% NaN3 containing 10 mM Tris–HCl (pH 8.0) was applied to the column and washed with (a) 0.5 M NaCl containing 10 mM Tris–HCl buffer (pH 8.0), (b) 0.5% sodium deoxycholate containing 10 mM Tris–HCl buffer (pH 8.0), and (c) 8 M urea containing 10 mM Tris–HCl buffer (pH 8.0). (B) SDS-PAGE analysis of the fraction of RBP bound to cholic acid. The fraction of RBP bound to cholic acid was subjected to SDS-PAGE and stained with CBB G250. A protein for MALDI-TOF mass spectrometry analysis is indicated by an arrow; lane 1, protein marker; lane 2, RBP. (C) Amino acid sequences of the hypothetical protein OsJ_13801 (NCBI accession No. EAZ29742) in NCBI protein databases. Matched peptide sequences are bold-faced and underlined.
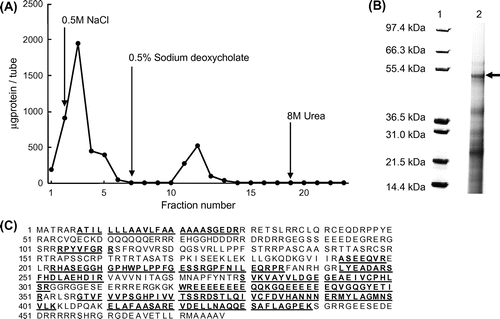
Discussion
Many studies have demonstrated that dietary plant proteins, such as soy protein and white rice protein, reduce serum cholesterol levels and promote the excretion of fecal steroids such as cholesterol and bile acid.Citation3–6) We observed a decrease in the serum total cholesterol level and an increase in fecal steroid excretion on feeding rats with dietary RBP. In addition, we found in vitro studies where RBP not only remarkably bound to bile acids but also inhibited the micellar solubility of cholesterol. Our previous study revealed that soy protein hydrolysates had the ability to bind bile acids and to inhibit the micellar solubility of cholesterol in vitro, and the hydrolysates repressed cholesterol absorption in the intestine and reduced serum cholesterol levels along with promoting the excretion of fecal steroids in vivo.Citation7,8) Moreover, in our preliminary study, hepatic cholesterol concentration was remarkably reduced in rats which were fed with high-cholesterol diet containing 5% RBP for 21 d. Considering the results of our past and present in vitro and in vivo studies, we suggest that RBP decreases serum and hepatic cholesterol levels via the enhancement of LDL receptor expression accompanied with the conversion of cholesterol to bile acids by the activation of cholesterol 7a-hydroxylase (CYP7A1) in the liver when RBP bound to bile acids represses the absorption of cholesterol and bile acids through inhibiting the micellar solubility in the intestine. Studies are needed to examine whether the intake of RBP enhances hepatic LDLR and CYP7A1 expressions and activities.
In this study, some bile acid-binding proteins were eluted from RBP and one of them was identified as hypothetical protein OsJ_13801 (NCBI accession No. EAZ29742). To our knowledge, this study is the first to identify the hypothetical protein OsJ_13801 as a novel bile acid-bound protein of RBP, eluted by a cholic acid-conjugated column. Recently, Lee et al. reported that dietary supplementation with 3% poly-γ-glutamic acid for 5 weeks reduced the serum LDL-cholesterol level of high-fat-diet-induced obese mice.Citation18) In addition, Duranti et al. demonstrated that plasma total cholesterol levels of rats fed with a hypercholesterolemic diet were lowered by daily (28 d) administration of 10 or 20 mg/kg body weight/d of α′ subunit from soybean 7S globulin (NCBI accession No. BAB64303)Citation19) which contained two tri-glutamic acid peptides EEE (residues 114–116 and 138–140) and two penta-glutamic acid peptides EEEEE (residues 64–68 and 175–179). Moreover, the identified hypothetical protein OsJ_13801 in this study had one tri-glutamic acid peptide EEE (residues 91–93), one hexa-glutamic acid peptide EEEEEE (residues 336–341), and one octa-glutamic acid peptide EEEEEEEE (residues 323–330) (Fig. (C)). We also verified using the computer program Expasy peptide cutter (http://us.expasy.org/tools/peptidecutter/) that these oligo-glutamic acid peptides in the both α′ subunit from soybean 7S globulin and hypothetical protein OsJ_13801 were not hydrolyzed by pepsin, trypsin, and chymotrypsin in silico. In addition, we have found that penta-glutamic acid EEEEE strongly inhibits the micellar solubility of cholesterol (unpublished data). Therefore, the glutamic acid-rich sequences of dietary plant protein constituents may be important in expressing their hypocholesterolemic capacities. However, we need to investigate whether purified hypothetical protein OsJ_13801and oligo-glutamic acid peptides express their bile acid-binding abilities and identify other bile acid-binding proteins from RBP by a cholic acid-conjugated column in the future.
In regard to relationship between amino acid compositions of dietary proteins and hypocholesterolemic effects, several studies have suggested that dietary proteins with the low ratios of Met/Gly and Lys/Arg, such as soy protein and white rice protein, have cholesterol-lowering effects.Citation4,20) Since PC is the primary phospholipid of serum lipoproteins, its synthesis is necessary for the assembly and secretion of very-low-density-lipoprotein in the liver.Citation21) As the dietary methionine level influences PC synthesis via the phosphatidylethanolamine N-methylation pathwayCitation22) by its action on phospholipid metabolism, methionine may affect plasma cholesterol concentrations. Sugiyama et al. reported that methionine and glycine content of dietary proteins affected the molecular species composition of plasma PC in rats.Citation23,24) Also, glycine contributes to methionine metabolism as an acceptor of the methyl group from S-adenosyl-methionine via the glycine N-methyltransferase system.Citation24) Thus, this high glycine content may depress the hypercholesterolemic action of methionine.Citation24) Meanwhile, Vahouny et al. showed that dietary soy protein supplemented with lysine to elevate the Lys/Arg ratio increased intestinal absorption of lipid emulsion in rats.Citation25) Therefore, the low Lys/Arg ratio may have the hypocholesterolemic action through the repression of cholesterol absorption. Taken all together, the ratios of Met/Gly and Lys/Arg in dietary proteins may relate to the regulation of cholesterol metabolism, although the regulation of cholesterol metabolism by the ratios of the amino acids remains controversial. In this study, the Met/Gly and Lys/Arg ratios in the RBP (Met/Gly, 0.26; Lys/Arg, 0.64) were lower than those in the casein (Met/Gly, 1.64; Lys/Arg, 3.04). Thus, our data lend support to the above mentioned preceding studies by the other researchers.
Taken together, the hypocholesterolemic action of RBP may be caused by the multiple nature of RBP, which contains some bile acid-binding proteins, such as hypothetical protein OsJ_13801, and lower ratios of Met/Gly and Lys/Arg. Future studies are needed to determine whether administration of the purified hypothetical protein OsJ_13801 improves cholesterol metabolism in vivo.
In conclusion, our results demonstrated that (1) RBP decreases serum total cholesterol levels accompanied with increasing excretion of fecal steroids in vivo, (2) RBP binds to bile acids and inhibits the micellar solubility of cholesterol in vitro, and (3) some bile acid-binding proteins of RBP were eluted by a chromatographic column conjugated with bile acids.
Supplemental material
The supplemental material for this paper is available at http://dx.doi.org/10.1080/09168451.2014.978260.
Acknowledgments
We are grateful to Oriza Oil and Fat Chemical Co., Ltd, for providing RBP. S. Nagaoka principally provided the ideas and leadership, designed and discussed the experiments, supervised the progress and direction of the study, and edited and communicated the manuscript; J. Wang did the experiments and wrote the manuscript; M. Shimada contributed the experiments and the drafting of the manuscript; Y. Kato contributed the experiments; M. Kusada contributed the experiments. The authors declare no conflicts of interest.
Additional information
Funding
References
- Executive summary of the third report of the National Cholesterol Education Program (NCEP) expert panel on detection, evaluation, and treatment of high blood cholesterol in adults (adult treatment panel III). J. Am. Med. Assoc. 2001;285:2486–2497.
- Carroll KK, Hamilton RMG. Effects of dietary protein and carbohydrate on plasma cholesterol levels in relation to atherosclerosis. J. Food Sci. 1975;40:18–23.10.1111/jfds.1975.40.issue-1
- Morita T, Oh-hashi A, Kasaoka S, Ikai M, Kiriyama S. Rice protein isolates produced by the two different methods lower serum cholesterol concentration in rats compared with casein. J. Sci. Food Agric. 1996;71:415–424.10.1002/(ISSN)1097-0010
- Morita T, Oh-hashi A, Takei K, Ikai M, Kasaoka S, Kiriyama S. Cholesterol-lowering effects of soybean, potato and rice proteins depend on their low methionine contents in rats fed a cholesterol-free purified diet. J. Nutr. 1997;127:470–477.
- Sugano M, Goto S, Yamada Y, Yoshida K, Hashimoto Y, Matsuo T, Kimoto M. Cholesterol-lowering activity of various undigested fractions of soybean protein in rats. J. Nutr. 1990;120:977–985.
- Sugano M, Yamada Y, Yoshida K, Hashimoto Y, Matsuo T, Kimoto M. The hypocholesterolemic action of the undigested fraction of soybean protein in rats. Atherosclerosis. 1988;72:115–122.10.1016/0021-9150(88)90071-8
- Nagaoka S, Miwa K, Eto M, Kuzuya Y, Hori G, Yamamoto K. Soy protein peptic hydrolysate with bound phospholipids decreases micellar solubility and cholesterol absorption in rats and caco-2 cells. J. Nutr. 1999;129:1725–1730.
- Nagaoka S, Nakamura A, Shibata H, Kanamaru Y. Soystatin (VAWWMY), a novel bile acid-binding peptide, decreased micellar solubility and inhibited cholesterol absorption in rats. Biosci. Biotechnol. Biochem. 2010;74:1738–1741.10.1271/bbb.100338
- Saunders RM. The properties of rice bran as a food stuff. Cereal Food World. 1990;35:632–636.
- Helm RM, Burks AW. Hypoallergenicity of rice bran protein. Cereal Food World. 1996;41:839–843.
- Shoji Y, Mita T, Isemura M, Mega T, Hase S, Isemura S, Aoyagi Y. A fibronectin-binding protein from rice bran with cell adhesion activity for animal tumor cells. Biosci. Biotechnol. Biochem. 2001;65:1181–1186.10.1271/bbb.65.1181
- Zhang H, Yokoyama WH. Concentration-dependent displacement of cholesterol in micelles by hydrophobic rice bran protein hydrolysates. J. Sci. Food Agric. 2012;92:1395–1401.10.1002/jsfa.v92.7
- Bruusgaard A, Sørensen H, Gilhuus-Moe CC, Skålhegg BA. Bile acid determination with different preparations of 3α-hydroxysteroid dehydrogenase. Clin. Chim. Acta. 1977;77:387–395.10.1016/0009-8981(77)90245-5
- Malchow-Møller A, Arffmann S, Larusso NF, Krag E. Enzymatic determination of total 3α-hydroxy bile acids in faeces validation in healthy subjects of a rapid method suitable for clinical routine purpose. Scand. J. Gastroenterol. 1982;17:331–333.10.3109/00365528209182063
- Miettinen TA, Ahrens EH Jr, Grundy SM. Quantitative isolation and gas–liquid chromatographic analysis of total dietary and fecal neutral steroids. J. Lipid Res. 1965;6:411–424.
- Pattinson N, Collins D, Campbell B. Covalent coupling of cholic acid to aminohexyl amino-Sepharose 4B and its use in affinity chromatography of serum albumin. J. Chromatogr. A. 1980;187:409–412.10.1016/S0021-9673(00)80473-2
- Makino S, Nakashima H, Minami K, Moriyama R, Takao S. Bile acid-binding protein from soybean seed: isolation, partial characterization and insulin-stimulating activity. Agric. Biol. Chem. 1988;52:803–809.10.1271/bbb1961.52.803
- Lee EH, Son WC, Lee SE, Kim BH. Anti-obesity effects of poly-γ-glutamic acid with or without isoflavones on high-fat diet induced obese mice. Biosci. Biotechnol. Biochem. 2013;77:1694–1702.10.1271/bbb.130253
- Duranti M, Lovati MR, Dani V, Barbiroli A, Scarafoni A, Castiglioni S, Ponzone C, Morazzoni P. The alpha′ subunit from soybean 7S globulin lowers plasma lipids and upregulates liver β-VLDL receptors in rats fed a hypercholesterolemic diet. J. Nutr. 2004;134:1334–1339.
- Kritchevsky D, Tepper SA, Czarnecki SK, Klurfeld DM. Atherogenicity of animal and vegetable protein: influence of the lysine to arginine ratio. Atherosclerosis. 1982;41:429–431.10.1016/0021-9150(82)90208-8
- Yao ZM, Vance DE. The active synthesis of phosphatidylcholine is required for very low density lipoprotein secretion from rat hepatocytes. J. Biol. Chem. 1988;263:2998–3004.
- Zeisel SH. Dietary choline: biochemistry, physiology, and pharmacology. Annu. Rev. Nutr. 1981;1:95–121.10.1146/annurev.nu.01.070181.000523
- Sugiyama K, Kanamori H, Tanaka S. Correlation of the plasma cholesterol-lowering effect of dietary glycine with the alteration of hepatic phospholipid composition in rats. Biosci. Biotechnol. Biochem. 1993;57:1461–1465.10.1271/bbb.57.1461
- Sugiyama K, Yamakawa A, Kumazawa A, Saeki S. Methionine content of dietary proteins affects the molecular species composition of plasma phosphatidylcholine in rats fed a cholesterol-free diet. J. Nutr. 1997;127:600–607.
- Vahouny GV, Chalcarz W, Satchithanandam S, Adamson I, Klurfeld DM, Kritchevsky D. Effect of soy protein and casein intake on intestinal absorption and lymphatic transport of cholesterol and oleic acid. Am. J. Clin. Nutr. 1984;40:1156–1164.