Abstract
The chemotaxis of wild-type NaCl-conditioned nematodes exposed to 100 mM NaCl, maintained on a growth medium containing 0.3 mM nicotine from first larva to young adult (YA) hermaphrodite, was significantly weaker than the chemotaxis of those maintained on a medium without nicotine. The result indicates that chronic nicotine exposure augments gustatory plasticity. The gustatory plasticity was also augmented when tph-1 mutants, with a defect in serotonin biosynthesis, were maintained on a medium containing nicotine until the YA stage. Chronic nicotine exposure did not augment gustatory plasticity in bas-1 mutants, which had defects in both serotonin and dopamine biosynthesis, and in cat-2 mutants, which had a defect in dopamine biosynthesis. However, augmentation of gustatory plasticity was observed when bas-1 and cat-2 mutants were maintained on a growth medium containing nicotine along with dopamine, suggesting that dopamine signaling is involved in the augmentation of gustatory plasticity due to chronic nicotine exposure.
Graphical Abstract
Chronic nicotine exposure-induced augmentation of gustatory plasticity in C. elegance is mediated through dopamine signaling.
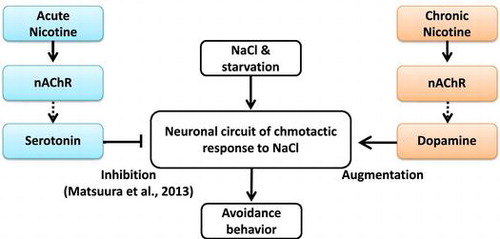
Introduction
In humans, nicotine, which is the main psychoactive ingredient in cigarettes, is responsible for psychopharmacological effects such as mild euphoria, increased energy, heightened arousal, and reduced stress and anxiety.Citation1) In experiments using rats and mice, acute administration of nicotine evoked changes in locomotor activity, grooming, and feeding,Citation2,3) and could improve attention, mental flexibility, and working memory function.Citation3,4) Chronic nicotine administration enhances the affective, anxiogenic, and neurochemical effects,Citation5) although there is no consistent result of learning behavior in rodents.Citation3) For example, chronic nicotine administration improves the result of radial-arm maze test in young, but not in aged rats.Citation6) Some experiments also revealed no effect of chronic nicotine exposure on performance efficiency of learning behavior,Citation7,8) but disrupts cognitive performance.Citation9) These inconsistent results of learning behavior using rodents may be caused by experimental procedure involving differences in the developmental stage of animals used, and the type of tests employed. Although the roles of nicotine in directly modulating the function of the nicotinic acetylcholine receptor (nAChR) are well known, the mechanisms leading to neuronal plasticity due to chronic nicotine exposure remain poorly understood. The aim of this study is to investigate the chronic effects of nicotine on behavioral plasticity, using established tests for learning behavior in model animal.
The nematode Caenorhabditis elegans is an excellent model to study behavioral plasticity because of its simple neuronal network of 302 neurons.Citation10) When nematodes are exposed to odorants, such as benzaldehyde and diacetyl, in the absence of food, they exhibit a decreased chemotactic response to the odorant.Citation11,12) This decrease is an adaptation to the chemical, and this behavioral adaptation is a result of associative learning in which the nematode forms an association between odorants and the environmental condition, such as starvation.Citation13) Nematodes are also capable of associating temperature and NaCl with food availability as demonstrated by the thermotaxis learning and salt chemotaxis learning paradigms.Citation14,15) The chemicals including food, and temperature are mainly sensed by the sensory neurons in the head sensory organ called the amphid.Citation16) Information about chemicals and temperature is transmitted to several interneurons, and causes activity in motoneurons to initiate a behavioral response.
C. elegans is also a model organism in studies to evaluate the effects of some drugs such as ethanol,Citation17,18) cocaine,Citation19) amphetamines,Citation20) and nicotine.Citation21–23) Ethanol administration interferes with gustatory plasticity in nematodes, i.e. salt chemotaxis learning, and serotonin signaling plays an essential role in this ethanol effect.Citation18) Cocaine and amphetamines are inhibitors of monoamine transporters, and acute treatment with these drugs changes locomotor activity in nematodes.Citation19,20) Dual effects of nicotine on locomotion speed, which are dependent on differences in its dosage and treatment duration, have also been revealed.Citation22)
Recently, we revealed that nicotine, which is an attractant, impaired gustatory plasticity and that serotonin has an essential role in modulating the acute effects of nicotine.Citation23) In the present study, we investigated the chronic effects of nicotine on gustatory plasticity in C. elegans in order to understand the mechanisms behind it.
Materials and methods
Animals
The wild-type strain of C. elegans (Bristol N2) and lev-1(e211), lev-1 (ok3201), unc-29(e1072), bas-1(tm351), bas-1(ad446), cat-2(e1112), and tph-1(mg280) mutants were obtained from the Caenorhabditis Genetics Center of the University of Minnesota. The nematodes were grown and maintained on nematode growth medium (NGM) agar plates (3 g/L NaCl, 2.5 g/L polypeptone, 5 mg/L cholesterol, 1 mM CaCl2, 1 mM MgSO4, 25 mM KH2PO4 (pH 6.0), and 17 g/L agar) in an incubator (Fukushima FMU-133I, Osaka, Japan) at 20 °C with Escherichia coli strain OP50 as food.
Synchronously staged young adult (YA) hermaphrodites were used in all experiments.Citation24,25) Last larval (L4) stage nematodes have a crescent structure around the vulva; this structure disappears in the YA stage and thus was absent in all experimental animals. To obtain synchronous hermaphrodites, 50 gravid nematodes were transferred to fresh NGM plates with or without 0.3 mM nicotine and incubated for 3 h at 25 °C to lay eggs. Subsequently, these gravid nematodes were removed and the plates with the eggs were maintained in an incubator at 20 °C. Some groups of nematodes were maintained on NGM plate containing 0–1.0 mM nicotine from first larva to YA hermaphrodite (until YA stage), to investigate the effects of chronic nicotine concentration on gustatory plasticity and locomotor activity.
Gustatory plasticity assays
In the gustatory plasticity assays (chemotaxis assays for NaCl), tissue culture dishes (9 cm in diameter) containing 1 mM CaCl2, 1 mM MgSO4, 5 mM KH2PO4 (pH 6.0), and 17 g/L agar (NaCl was omitted) were used. To obtain a concentration gradient of NaCl, 100 mM NaCl (7 μL) was spotted on the surface of an assay plate (NaCl location: Fig. ) at 18 and 3 h before the start of the assay.Citation23,24,26,27) The assay plate was stored in an incubator at 20 °C. A 1 μL of 0.5 M sodium azide was spotted on the same location as that of NaCl shortly before the chemotaxis assay to anesthetize the nematodes. As a control, 1 μL of 0.5 M sodium azide was also spotted on a control location.
Fig. 1. Experimental procedure for gustatory plasticity assay.
Notes: Wild-type nematodes and mutants, which were maintained on NGM plate with or without nicotine from first larva to YA hermaphrodite, were transferred to a pre-exposure wash buffer without NaCl (mock-conditioned nematodes) or wash buffer containing 100 mM NaCl (NaCl-conditioned nematodes). The nematodes were maintained at 20 °C for 15 min in their respective pre-exposure solutions. Approximately 30 nematodes were then placed at their original locations on the assay plate and allowed to move freely for 90 min.
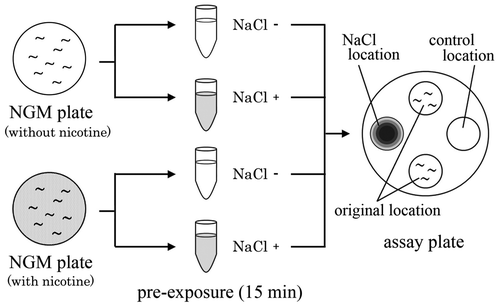
To investigate the effects of chronic nicotine exposure on gustatory plasticity, nematodes in the NGM plates without and with nicotine were collected and washed thrice with a pre-exposure solution: wash buffer without NaCl (mock-conditioned nematodes) or wash buffer containing 100 mM NaCl (NaCl-conditioned nematodes). The nematodes were maintained at 20 °C for 15 min in their respective pre-exposure solutions (Fig. ). After the pre-exposure period, they were washed once with the wash buffer, which did not include NaCl, by centrifugation for 60 s at approximately 450 × g, to eliminate NaCl from the body. In the present paper, mock-conditioned nematodes, which were maintained on NGM plate without and with nicotine, were referred to as nicotine−/NaCl− and nicotine+/NaCl− nematodes, respectively. NaCl-conditioned nematodes, which were maintained on NGM plate without and with nicotine, were referred to as nicotine−/NaCl+ and nicotine+/NaCl+ nematodes, respectively.
Subsequently, approximately 30 nematodes were placed at the original location, equidistant (2.8 cm) from the NaCl and control spots. The nematodes were dried, separated by gently touching them with a Kimwipe, and allowed to move freely on the assay plate for 90 min. Every 10 min, the number of nematodes at the NaCl and control locations (circle with a 1-cm radius) was determined using a microscope (Olympus SZ40). The chemotaxis index was calculated based on the number of nematodes that gathered at the NaCl location Citation28) using the following equation: chemotaxis index = (number of nematodes at the NaCl location−number of nematodes at the control location)/(total number of nematodes on the plate). The assays were performed during the daytime in an experimental room maintained closely at 20 °C. Each measurement was repeated more than three times.
Dopamine treatment
To determine the role of dopamine in augmenting gustatory plasticity due to chronic nicotine exposure, N2 nematodes, and bas-1 and cat-1 mutants, were treated with dopamine on an NGM plate for approximately 75 h (from hatch to the YA stage). Dopamine hydrochloride (LKT Laboratories, Inc., Minnesota, USA) was dissolved in NGM plates at a final concentration of 20 mM. The efficacy of the determined concentration was confirmed using methods described previously.Citation29)
Measurement of locomotor activity
The spontaneous locomotor rate, which is an index of locomotion speed, was measured to determine the locomotor activity of the nematodes. A nematode, which was maintained on NGM plate containing 0–1.0 mM nicotine and conditioned using pre-exposure solutions, was placed on a no-attractant assay plate after washing with wash buffer. Then, the number of body bends, including omega turns,Citation30) was measured for each animal during five separate 30-s time periods at 1-min intervals. Values of five trials were averaged over the number of animals used (n ≥ 10).
Statistical analysis
A one-way analysis of variance was used for multiple comparisons of the chemotaxis indices for the various nicotine concentrations and spontaneous locomotion rate. Differences in the chemotaxis indices and spontaneous locomotion rate between mock-conditioned and NaCl-conditioned nematodes, and between nematodes maintained with and without nicotine were analyzed using student’s t-test. Values are presented as mean ± standard errors. A P value of <0.05 was considered significant.
Results
Augment of gustatory plasticity due to chronic nicotine exposure
The chemotaxis index of nicotine−/NaCl+ nematodes was significantly lower than that of nicotine−/NaCl− nematodes (0.18 ± 0.03 vs. 0.52 ± 0.02, respectively) at 90 min for 100 mM NaCl, indicating that exposure to 100 mM NaCl induced gustatory plasticity (Fig. ). When nematodes were maintained at 0.01 mM nicotine, the chemotaxis index for NaCl of nicotine+/NaCl+ nematodes was almost the same as that of nicotine−/NaCl+ nematodes. When nematodes were maintained at 0.03, 0.1, and 0.3 mM nicotine, the chemotaxis indices for NaCl of nicotine+/NaCl+ nematodes were significantly lower than those of nicotine−/NaCl+ nematodes (p < 0.05) in spite of no change in the indices among nicotine+/NaCl− (mock-conditioned) nematodes (F4,132 = 0.08, NS; Fig. (A)). For example, when nematodes were maintained at 0.3 mM nicotine, the time course of chemotaxis index of nicotine+/NaCl− nematodes was almost the same as that of nicotine−/NaCl− nematodes, whereas the time course of the index of nicotine+/NaCl+ nematodes significantly declined in comparison with that of nicotine−/NaCl+ nematodes (0.00 ± 0.05 and 0.18 ± 0.03 at 90 min, respectively, p < 0.01) (Fig. (B)). These results suggest that nicotine+/NaCl+ nematodes showed augmentation of gustatory plasticity due to chronic nicotine exposure.
Fig. 2. Augmentation of gustatory plasticity due to chronic nicotine exposure.
Notes: (A) Chemotaxis index for 100 mM NaCl in N2 nematodes. When the nematodes were maintained on NGM plate with 0.03−0.3 mM nicotine, the chemotaxis indices of nicotine+/NaCl+ nematodes were lower than those of nicotine−/NaCl+ nematodes (F5,138 = 7.02, p < 0.001), indicating augmentation of gustatory plasticity due to chronic nicotine exposure. (B) Time course changes in the average chemotaxis index for NaCl in each of the conditioned nematodes, which were maintained on the medium containing 0.3 mM nicotine until YA stage. Error bars indicate standard errors. *p < 0.05, **p < 0.01, and ***p < 0.001 compared with nicotine−/NaCl− or nicotine−/NaCl+ nematode (n ≥ 17 assays).
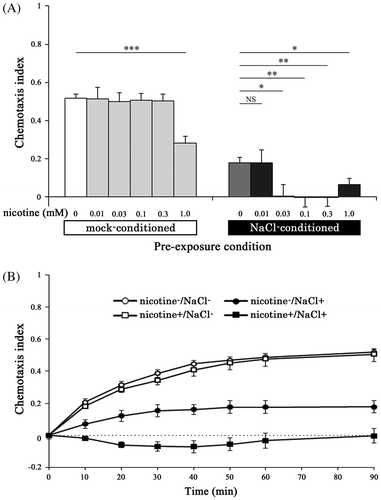
Although the chemotaxis index of nicotine+/NaCl+ nematodes, which were maintained at 1.0 mM nicotine, were significantly lower than that of nicotine−/NaCl+ nematodes (0.06 ± 0.03 and 0.18 ± 0.03 at 90 min, respectively, p < 0.05), the index of nicotine+/NaCl− nematodes was also lower than that of nicotine−/NaCl− (0.28 ± 0.04 and 0.52 ± 0.02 at 90 min, respectively, p < 0.001) (Fig. (A)). No significant difference in the spontaneous locomotor activity was observed among the nicotine−/NaCl−, nicotine+/NaCl−, nicotine−/NaCl+ and nicotine + /NaCl+ nematodes (F11,159 = 0.92, NS) (Fig. ). In subsequent experiments, therefore, we used 0.3 mM nicotine for chronic exposure.
Effect of nAChR on augmentation of gustatory plasticity
The chemotactic response of lev-1 and unc-29 mutants, which have a deficit in non-alpha subunits of nAChR,Citation31) to NaCl was examined to confirm whether nicotine affects the augmentation of gustatory plasticity via nAChR (Fig. ). The chemotaxis index was lower in nicotine−/NaCl+ lev-1 mutants compared with nicotine−/NaCl− mutants (p < 0.001), and in nicotine+/NaCl+ lev-1 mutants compared with nicotine+/NaCl− mutants (p < 0.001). The chemotaxis index of nicotine−/NaCl+ unc-29 mutants was lower than that of nicotine−/NaCl− mutants (p < 0.001), and the index of nicotine+/NaCl+ unc-29 mutants was also lower than that of nicotine+/NaCl− mutants (p < 0.01). These results indicate that both lev-1 and unc-29 mutants exhibit gustatory plasticity regardless of nicotine exposure.
Fig. 4. Effects of nicotinic acetylcholine receptor (nAChR) mutation on augmentation of gustatory plasticity due to chronic nicotine exposure.
Notes: There was no significant difference in chemotaxis index between nicotine−/NaCl+ and nicotine+/NaCl+ lev-1 and unc-29 mutants, which were defective in nAChR function, suggesting that augment of gustatory plasticity was caused by signals via nAChR. Error bars indicate standard errors. **p < 0.01 (n ≥ 16 assays).
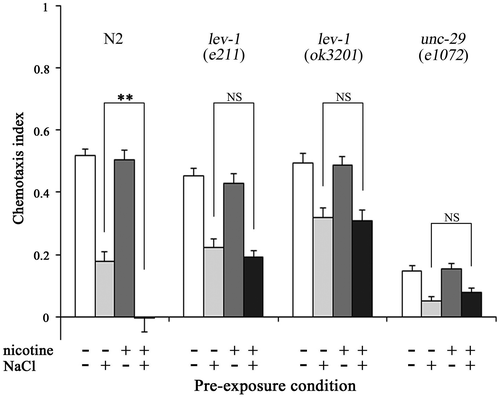
On the other hand, there was no significant change in the chemotaxis index between nicotine−/NaCl+ and nicotine+/NaCl+ in both lev-1 and unc-29 mutants (Fig. ), indicating that mutations of lev-1 and unc-29 gene do not cause augmentation of gustatory plasticity upon exposure to nicotine. The result suggests that the signaling via nAChRs causes augmentation of gustatory plasticity due to chronic nicotine exposure.
Necessity of dopamine signaling in augmentation of gustatory plasticity
To determine the effects of the neurotransmitters on augmentation of gustatory plasticity due to chronic nicotine exposure, we used mutants defective in serotonin and/or dopamine secretion, because serotonin and dopamine are neurotransmitters involved in behavioral plasticity in nematodes.Citation13,17,23,25,32) bas-1(tm351) and bas-1(ad446) mutants are defective in an aromatic amino acid decarboxylase required for serotonin and dopamine production.Citation33) The chemotaxis index for NaCl of nicotine−/NaCl+ bas-1 mutants was significantly lower than that of nicotine−/NaCl− mutants (p < 0.01). When bas-1 mutants were maintained on a medium containing 0.3 mM nicotine until YA stage, the chemotaxis index of nicotine+/NaCl+ mutants was also lower than that of nicotine+/NaCl− mutants (p < 0.01). There was no significant difference in chemotaxis index between nicotine−/NaCl+ and nicotine+/NaCl+ bas-1 mutants (Fig. ). The results indicate that augmentation of gustatory plasticity due to chronic nicotine exposure did not occur in bas-1 mutants, and suggest that serotonin and/or dopamine are involved in regulating the chronic effect of nicotine on gustatory plasticity.
Fig. 5. Dopamine signaling is involved in augmentation of gustatory plasticity due to chronic nicotine exposure.
Notes: Effects of chronic nicotine exposure on gustatory plasticity in wild-type nematodes and mutants. bas-1 mutants are defective in serotonin and dopamine biosynthesis, cat-2 mutants are defective in dopamine biosynthesis, and tph-1 mutants are defective in serotonin biosynthesis. The observations suggest a possibility that dopamine signaling mediates the regulation of the chronic effect of nicotine on gustatory plasticity. Error bars indicate standard errors. **p < 0.01, ***p < 0.001 (n ≥ 16 assays).
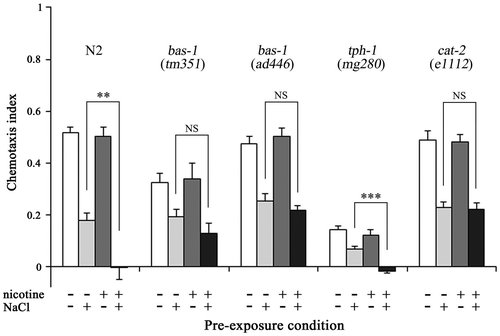
The chemotactic response of tph-1 and cat-2 mutants with defects in serotonin synthesis and dopamine synthesis,Citation33,34) respectively, was investigated to determine the neurotransmitters involved in the regulation of the chronic effect of nicotine on gustatory plasticity. The chemotaxis index of nicotine−/NaCl+ tph-1 mutants was lower than that of nicotine−/NaCl− mutants (p < 0.001). The chemotaxis index of nicotine+/NaCl+ tph-1 mutants was lower than that of nicotine−/NaCl+ mutants (−0.02 ± 0.01 and 0.07 ± 0.01 at 90 min, respectively, p < 0.001) (Fig. ), indicating augmentation of gustatory plasticity in tph-1 mutants. In cat-2 mutants, the chemotaxis index was significantly lower in nicotine−/NaCl+ mutants compared with nicotine−/NaCl− mutants (p < 0.001) and in nicotine+/NaCl+ mutants compared with nicotine+/NaCl− mutants (p < 0.001). In contrast to result of tph-1 mutants, there was no significant difference in chemotaxis index between nicotine−/NaCl+ and nicotine+/NaCl+ in cat-2 mutants (Fig. ), indicating no augmentation of gustatory plasticity. These results suggest that dopamine mediates the regulation of the chronic effect of nicotine on gustatory plasticity.
In the presence of 20 mM dopamine, augmentation of gustatory plasticity due to chronic nicotine exposure was observed in both bas-1 and cat-2 mutants, consistent with the results obtained from N2 nematodes (Fig. ). The chemotaxis indices of nicotine−/NaCl+ bas-1 and cat-2 mutants were lower than those of nicotine−/NaCl− mutants (p < 0.001), and significant differences were also observed in the chemotaxis indices between nicotine+/NaCl+ and nicotine+/NaCl− of bas-1 and cat-2 mutants (p < 0.001). Moreover, the chemotaxis indices of nicotine+/NaCl+, for both bas-1 and cat-2 mutants, were lower than that of nicotine−/NaCl+ (p < 0.05), indicating that dopamine signals are associated with augmentation of gustatory plasticity due to chronic nicotine exposure.
Fig. 6. Dopamine supplementation augments gustatory plasticity in bas-1 and cat-2 mutants.
Notes: When bas-1 and cat-2 mutants were bred on NGM plates containing nicotine with dopamine until YA stage, augment of gustatory plasticity due to chronic nicotine exposure was observed in both mutants. The results strongly support the role of dopamine in this response. Error bars indicate standard errors. *p < 0.05, ***p < 0.001 (n ≥ 16 assays).
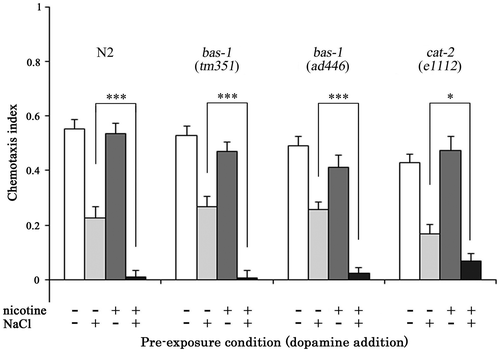
Discussion
Effects of chronic nicotine exposure on locomotor activity, sensitivity, and gustatory plasticity
It is well known that nicotine acts as a nAChR agonist, and is a potent parasympathomimetic alkaloid and a habitual chemical, which is toxic at high concentrations. In nematodes, nicotine exposure affects the locomotor activity,Citation21–23) egg-laying behavior, and body wall muscle activity.Citation35,36) Decline in spontaneous locomotor activity was observed when the nematodes were maintained on medium with nicotine concentrations above 3.0 mM, although there was no effect on the locomotor activity during acute nicotine exposure in the range of 0.1–3.0 mM.Citation22,23) In the present study, we observed that the spontaneous locomotor activity did not change even when the nematodes were maintained on NGM plate containing 0.01–1.0 mM nicotine until YA stage (Fig. ).
The nematodes showed a preference response to low concentration of nicotine (lower than 1.0 mM), and the preference behavior declined over 3.0 mM nicotine.Citation23) High concentrations of acute nicotine exposure induce muscle hypercontraction paralysis Citation22) and cause rapid paralysis of the body wall muscles within 10–15 min.Citation37) These observations suggest that the nematodes are affected by nicotine toxicity when they were maintained on a medium with more than 3.0 mM nicotine.Citation23) In the present study, the chemotactic response of nematodes, exposed chronically to 1.0 mM nicotine (nicotine+/NaCl− nematodes), to NaCl was significantly decreased in comparison with that of nicotine−/NaCl− nematodes (Fig. (A)) in spite of no change in locomotor activity (Fig. ), indicating the effect of nicotine toxicity on sensory integration by the chronic exposure. On the other hand, when the nematodes were maintained on 0.3 mM nicotine chronically, nicotine+/NaCl− nematodes showed almost the same time course of the chemotaxis index as nicotine−/NaCl− nematodes (Fig. (B)). These results indicate that chronic exposure to 0.3 mM nicotine does not alter their chemical sensitivity, ability to respond, or locomotor activity. We concurred that the gustatory plasticity experimental procedure employed in this study was suitable for evaluating the effect of chronic nicotine exposure on associative learning.
The chemotactic response of nicotine−/NaCl+ nematodes to NaCl was significantly lower than that of nicotine−/NaCl− nematodes (Fig. (A) and (B)). It is well known that nematodes show an avoidance response to NaCl after pre-exposure to this chemical without food,Citation18,38,39) whereas NaCl is an attractant for the nematodes. This gustatory plasticity represents a simple form of associative learning. The gustatory plasticity was augmented by chronic nicotine exposure (Fig. (A)), but is interfered by acute nicotine exposure.Citation23) The augment of gustatory plasticity due to chronic nicotine exposure may be caused by a mechanism other than the mechanism employed to inhibit gustatory plasticity during acute nicotine exposure, although both acute and chronic nicotine exposure affect the modulation of neural or molecular functions underlying gustatory plasticity.
The effects of chronic nicotine administration on learning behavior have been reported in studies involving rodents, with no consistent result.Citation3) Some reports reveal that chronic administration improved the working memory function,Citation3,6) and that nicotine exposure stimulated the release of a variety of neurotransmitters including dopamine and serotonin.Citation3) In nematodes, as mentioned above, chronic nicotine exposure augmented the gustatory plasticity. It is possible that the augment of gustatory plasticity in nematodes, by chronic nicotine exposure, may be regulated by release of neurotransmitters, a mechanism similar to that found in rodents.
Modulation of dopamine signaling to augment gustatory plasticity due to chronic nicotine exposure
Augment of gustatory plasticity was observed in tph-1 mutants but not in bas-1 and cat-2 mutants (Fig. ). When tph-1 mutants were maintained on growth medium containing nicotine until their YA stage, significant difference in the chemotaxis index was observed between nicotine−/NaCl+ and nicotine+/NaCl+ mutants (p < 0.001). In contrast, the chemotaxis indices of nicotine+/NaCl+ bas-1 and cat-2 mutants were almost the same as those of nicotine−/NaCl+ mutants (Fig. ). The genes bas-1, cat-2, and tph-1 encode enzymes involved in serotonin and/or dopamine production, and mutations in these genes result in deficiencies in serotonin and/or dopamine secretion. bas-1 encodes a serotonin- and dopamine-synthetic aromatic amino acid decarboxylase that is required for the synthesis of serotonin from 5-hydroxytryptophan;Citation33,34) therefore, bas-1 mutants have a deficiency in both serotonin and dopamine secretion. Dopamine synthesis involves the CAT-2 tyrosine hydroxylase, and therefore cat-2 mutants have a defect in dopamine biosynthesis in the nervous system.Citation34) tph-1 encodes tryptophan hydroxylase, an enzyme that catalyzes the rate-limiting first step in serotonin biosynthesis, and mutations of tph-1 impair serotonin secretion.Citation33,34) The inability of the bas-1 and cat-2 mutants to augment gustatory plasticity in response to chronic nicotine exposure, and its reversal when they were bred on NGM plates containing nicotine with dopamine (Fig. ), suggests that dopamine is the main mediator in this response.
Serotonin and dopamine play a role during the plasticity response.Citation39) In salt-associative learning, involvement of both serotonin and dopamine as a component of food signaling and insulin-like signaling pathways is reported.Citation15,32,39) In the present study, bas-1, tph-1, and cat-2 mutants subjected to nicotine−/NaCl+ treatment displayed gustatory plasticity (Figs. and ) that is similar to the results of Wang et al.Citation18) Hukema et al. also reported that gustatory plasticity was significantly, but not completely, inhibited in these mutants.Citation39) Serotonin and dopamine signals may partially affect the gustatory plasticity although they play important roles in the learning process of nematodes.
Involvement of dopamine signaling pathway in the effects of chronic nicotine exposure
Nicotine is an agonist to acetylcholine, which plays a significant role in learning processes in the brain in many species. unc-29 and lev-1 encode non-alpha subunits of nAChRs, and UNC-29 and LEV-1 proteins are required for normal locomotion, regulation of egg-laying behavior, and formation of a cation channel expressed with UNC-38.Citation21,31) In the present study, the inability of nicotine to augment gustatory plasticity in both unc-29 and lev-1 mutants was observed (Fig. ). The results suggest that nicotine affects the nAChR involved in salt chemotaxis learning and augmentation of gustatory plasticity.
One possible mechanism that explains this effect upon chronic nicotine exposure paradigm is the dopamine-mediated modulation of gustatory plasticity. It is well known that nAChR detects nicotine, leading to release of dopamine. As mentioned, dopamine is associated with learning behavior.Citation39) We speculate that chronic exposure to low nicotine concentration from first larva to YA hermaphrodite causes a successive release of dopamine through nAChRs, and that these signals augment gustatory plasticity. Previously, we reported that acute nicotine exposure during the learning period, e.g. associative conditioning between NaCl and food quantity, caused a release of serotonin signals through nAChRs and inhibited gustatory plasticity.Citation23) Nicotine-mediated modulatory effects on gustatory plasticity may involve a mechanism that is partially similar to both acute and chronic exposures, but could be different with respect to the efficacy of nicotine exposure. Our next question is when and how the acute and chronic effects of nicotine on gustatory plasticity are switched during developmental period. To clarify this question, further behavioral analysis and gene expression, which are concerned with change in gustatory plasticity due to nicotine exposure, are required.
In conclusion, chronic nicotine exposure augments gustatory plasticity, salt chemotaxis learning, in C. elegance. The augmentation of gustatory plasticity due to the chronic exposure is mediated through dopamine signaling.
Acknowledgments
The nematode strains were provided by the Caenorhabditis Genetics Center, which is funded by the NIH Office of Research Infrastructure Programs (P40 OD010440).
Additional information
Funding
References
- Herman AI, Sofuoglu M. Cognitive effects of nicotine: genetic moderators. Addict. Biol. 2010;15:250–265.10.1111/adb.2010.15.issue-3
- Scheufele PM, Faraday MM, Grunberg NE. Nicotine administration interacts with housing conditions to alter social and non-social behaviors in male and female Long-Evans rats. Nicotine Tod. Res. 2000;2:69–78.
- Levin ED, McClernon FJ, Rezvani AH. Nicotinic effects on cognitive function: behavioral characterization, pharmacological specification, and anatomic localization. Psychopharmacology. 2006;184:523–539.10.1007/s00213-005-0164-7
- Allison C, Shoaib M. Nicotine improves performance in an attentional set shifting task in rats. Neuropharmacology. 2013;64:314–320.10.1016/j.neuropharm.2012.06.055
- Tejeda HA, Natividad LA, Orfila JE, Torres OV, O’Dell LE. Dysregulation of kappa-opioid receptor systems by chronic nicotine modulate the nicotine withdrawal syndrome in an age-dependent manner. Psychopharmacology. 2012;224:289–301.10.1007/s00213-012-2752-7
- Levin ED, Torry D. Acute and chronic nicotine effects on working memory in aged rats. Psychopharmacology. 1996;123:88–97.10.1007/BF02246285
- Vicens P, Carrasco MC, Redolat R. Effects of early training and nicotine treatment on the performance of male NMRI mice in the water maze. Neural Plast. 2003;10:303–317.10.1155/NP.2003.303
- Leach PT, Cordero KA, Gould TJ. The effects of acute nicotine, chronic nicotine, and withdrawal from chronic nicotine on performance of a cued appetitive response. Behav. Neurosci. 2013;127:303–310.10.1037/a0031913
- Hambsch B, Keyworth H, Lind J, Otte DM, Racz I, Kitchen I, Bailey A, Zimmer A. Chronic nicotine improves short-term memory selectively in a G72 mouse model of schizophrenia. Br. J. Pharmacol. 2014;171:1758–1771.10.1111/bph.12578
- White JG, Southgate E, Thomson JN, Brenner S. The structure of the nervous system of the nematode Caenorhabditis elegans. Philos. Trans. R. Soc. Lond. [Biol.]. 1986;314:1–340.10.1098/rstb.1986.0056
- Colbert HA, Bargmann CI. Odorant-specific adaptation pathways generate olfactory plasticity in C. elegans. Neuron. 1995;14:803–812.10.1016/0896-6273(95)90224-4
- Nishino A, Kanno R, Matsuura T. The role of oxygen intermediates in the retention time of diacetyl adaptation in the nematode Caenorhabditis elegans. J. Exp. Zool. A. 2013;319:431–439.10.1002/jez.v319.8
- Nuttley WM, Atkinson-Leadbeater KP, van der Kooy D. Serotonin mediates food-odor associative learning in the nematode Caenorhabditis elegans. Proc. Natl. Acad. Sci. USA. 2002;99:12449–12454.10.1073/pnas.192101699
- Wen JY, Kumar N, Morrison G, Rambaldini G, Runciman S, Rousseau J, van der Kooy D. Mutations that prevent associative learning in C. elegans. Behav. Neurosci. 1997;111:354–368.10.1037/0735-7044.111.2.354
- Tomioka M, Adachi T, Suzuki H, Kunitomo H, Schafer W, Iino Y. The insulin/PI 3-kinase pathway regulates salt chemotaxis learning in Caenorhabditis elegans. Neuron. 2006;51:613–625.10.1016/j.neuron.2006.07.024
- Bargmann CI, Horvitz HR. Chemosensory neurons with overlapping functions direct chemotaxis to multiple chemicals in C. elegans. Neuron. 1991;7:729–742.10.1016/0896-6273(91)90276-6
- Lee J, Jee C, McIntire SL. Ethanol preference in C. elegans. Genes Brain Behav. 2009;8:578–585.10.1111/gbb.2009.8.issue-6
- Wang Y, Tang L, Feng X, Du W, Liu BF. Ethanol interferes with gustatory plasticity in Caenorhabditis elegans. Neurosci. Res. 2011;71:341–347.
- Ward A, Walker VJ, Feng Z, Xu XZ. Cocaine modulates locomotion behavior in C. elegans. PLoS ONE. 2009;4:e5946.10.1371/journal.pone.0005946
- Carvelli L, Matthies DS, Galli A. Molecular mechanisms of amphetamine actions in Caenorhabditis elegans. Mol. Pharmacol. 2010;78:151–156.10.1124/mol.109.062703
- Feng Z, Li W, Ward A, Piggott BJ, Larkspur ER, Sternberg PW, Xu XZS. A C. elegans model of nicotine-dependent behavior: regulation by TRP-family channels. Cell. 2006;127:621–633.10.1016/j.cell.2006.09.035
- Sobkowiak R, Kowalski M, Lesicki A. Concentration- and time-dependent behavioral changes in Caenorhabditis elegans after exposure to nicotine. Pharmacol. Biochem. Behav. 2011;99:365–370.10.1016/j.pbb.2011.05.019
- Matsuura T, Miura H, Nishino A. Inhibition of gustatory plasticity due to acute nicotine exposure in the nematode Caenorhabditis elegans. Neurosci. Res. 2013;77:155–161.10.1016/j.neures.2013.09.001
- Matsuura T, Endo S, Iwamoto R, Takahashi H, Ichinose M. Developmental changes in chemotactic response and choice of two attractants, sodium acetate and diacetyl, in the nematode Caenorhabditis elegans. Comp. Biochem. Physiol. A. 2007;147:920–927.10.1016/j.cbpa.2007.02.023
- Matsuura T, Oda T, Hayashi G, Sugisaki D, Ichinose M. Enhancement of chemotactic response to sodium acetate in the nematode Caenorhabditis elegans. Zool. Sci. 2010;27:629–637.10.2108/zsj.27.629
- Matsuura T, Oikawa T, Wakabayashi T, Shingai R. Effect of simultaneous presentation of multiple attractants on chemotactic response of the nematode Caenorhabditis elegans. Neurosci. Res. 2004;48:419–429.10.1016/j.neures.2003.12.008
- Matsuura T, Sato T, Shingai R. Interactions between Caenorhabditis elegans individuals during chemotactic response. Zool. Sci. 2005;22:1095–1103.10.2108/zsj.22.1095
- Bargmann CI, Hartwieg E, Horvitz HR. Odorant-selective genes and neurons mediate olfaction in C. elegans. Cell. 1993;74:515–527.10.1016/0092-8674(93)80053-H
- Sanyal S, Wintle RF, Kindt KS, Nuttley WM, Arvan R, Fitzmaurice P, Bigras E, Merz DC, Hébert TE, van der Kooy D, Schafer WR, Culotti JG, Van Tol HH. Dopamine modulates the plasticity of mechanosensory responses in Caenorhabditis elegans. EMBO J. 2004;23:473–482.10.1038/sj.emboj.7600057
- Duhon SA, Johnson TE. Movement as an index of vitality: comparing wild type and the age-1 mutant of Caenorhabditis elegans. J. Gerontol. A Biol. Sci. Med. Sci. 1995;50A:B254–B261.10.1093/gerona/50A.5.B254
- Fleming JT, Squire MD, Barnes TM, Tornoe C, Matsuda K, Ahnn J, Fire A, Sulston JE, Barnard EA, Sattelle DB, Lewis JA. Caenorhabditis elegans levamisole resistance genes lev-1, unc-29, and unc-38 encode functional nicotinic acetylcholine receptor subunits. J. Neurosci. 1997;17:5843–5857.
- Saeki S, Yamamoto M, Iino Y. Plasticity of chemotaxis revealed by paired presentation of a chemoattractant and starvation in the nematode Caenorhabditis elegans. J. Exp. Biol. 2001;204:1757–1764.
- Loer CM, Kenyon CJ. Serotonin-deficient mutants and male mating behavior in the nematode Caenorhabditis elegans. J. Neurosci. 1993;13:5407–5417.
- Sawin ER, Ranganathan R, Horvitz HR. C. elegans locomotory rate is modulated by the environment through a dopaminergic pathway and by experience through a serotonergic pathway. Neuron. 2000;26:619–631.10.1016/S0896-6273(00)81199-X
- Waggoner LE, Dickinson KA, Poole DS, Tabuse Y, Miwa J, Schafer WR. Long-term nicotine adaptation in Caenorhabditis elegans involves PKC-dependent changes in nicotinic receptor abundance. J. Neurosci. 2000;20:8802–8811.
- Gottschalk A, Almedom RB, Schedletzky T, Anderson SD, Yates JR, Schafer WR. Identification and characterization of novel nicotinic receptor-associated proteins in Caenorhabditis elegans. EMBO J. 2005;24:2566–2578.10.1038/sj.emboj.7600741
- Matta SG, Balfour DJ, Benowitz NL, Boyd RT, Buccafusco JJ, Caggiula AR, Craig CR, Collins AC, Damaj MI, Donny EC, Gardiner PS, Grady SR, Heberlein U, Leonard SS, Levin ED, Lukas RJ, Markou A, Marks MJ, McCallum SE, Parameswaran N, Perkins KA, Picciotto MR, Quik M, Rose JE, Rothenfluh A, Schafer WR, Stolerman IP, Tyndale RF, Wehner JM, Zirger JM. Guidelines on nicotine dose selection for in vivo research. Psychopharmacology. 2007;190:269–319.10.1007/s00213-006-0441-0
- Jansen G, Weinkove D, Plasterk RH. The G-protein gamma subunit gpc-1 of the nematode C. elegans is involved in taste adaptation. EMBO J. 2002;21:986–994.10.1093/emboj/21.5.986
- Hukema RK, Rademakers S, Jansen G. Gustatory plasticity in C. elegans involves integration of negative cues and NaCl taste mediated by serotonin, dopamine, and glutamate. Learn Mem. 2008;15:829–836.10.1101/lm.994408