Graphical Abstract
Changes of accumulation levels for lipid droplet (A) and caleosin mRNA from Chlorella vulgaris TISTR 8580 cells
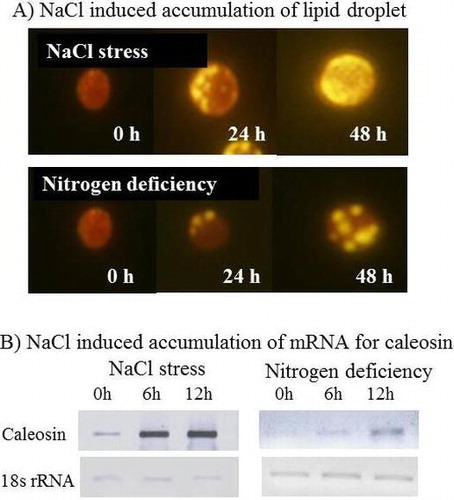
Abstract
Physiological and functional properties of lipid droplet-associated proteins in algae remain scarce. We report here the caleosin gene from Chlorella vulgaris encodes a protein of 279 amino acid residues. Amino acid sequence alignment showed high similarity to the putative caleosins from fungi, but less to plant caleosins. When the C. vulgaris TISTR 8580 cells were treated with salt stress (0.3 M NaCl), the level of triacylglycerol increased significantly. The mRNA contents for caleosin in Chlorella cells significantly increased under salt stress condition. Caleosin gene was expressed in E. coli. Crude extract of E. coli cells exhibited the cumene hydroperoxide-dependent oxidation of aniline. Absorption spectroscopy showed a peak around 415 nm which was decreased upon addition of cumene hydroperoxide. Native polyacrylamide gel electrophoresis suggests caleosin existed as the oligomer. These data indicate that a fresh water C. vulgaris TISTR 8580 contains a salt-induced heme-protein caleosin.
The caleosins are a group of calcium-binding proteins that can be found in multicellular plants and green algae.Citation1,2) Caleosins are frequently described as lipid droplet (LD)-associated proteins that occur in storage tissues, such as seeds. Triacylglycerols are major lipids in LD which is stabilized with a surface layer of phospholipids and structure proteins termed LD proteins. In higher plants, three classes of integral proteins—oleosin, caleosin, and steroleosin—are known as LD proteins, of which oleosin is the major protein in most cases.Citation1) In chlorophytes (green algae) such as Chlamydomonas reinhardtii,Citation3) Haematococcus pluvialis,Citation4) and Dunaliella,Citation5) a protein termed major lipid droplet protein (MLDP) rather than oleosin was reported as a LD protein. However, it was shown that MLDP is associated with endoplasmic reticulum (ER) in C. reinhardtii.Citation6) In C. reinhardtii, oleosin or oleosin-like gene can be detected, but their expression levels were extremely low.Citation7) In a marine Auxenochlorella protothecoides, no oleosin, steroleosin, and MLDP were detected, but caleosin was.Citation8) Thus, LD proteins in the single cell algae remain to be clarified. With the growing importance of algal feedstock for the production of biofuels and high-value lipids, functional characterization of LD proteins in microalgae is required.Citation9)
Caleosins are divided into three classes depending on the location of hydrophobic domain.Citation10,11) The hydrophobic region in class I is located centrally, the hydrophobic region is shifted to the N terminus in class II and appears randomly located in fungal caleosins (class III). Plant caleosins belong to class I and class II. In Arabidopsis, seven caleosin proteins (AtCLO1-7) were found, of which heme oxygenase activities have been detected in class I AtCLO1 (At4g26740) and in class II AtCLO4 (At1g70670) that are regulated by one or two conserved ferric-binding histidine residues.Citation10,11) The peroxygenase activities may be involved in the oxylipin metabolites that play prominent roles in plant responses to a range of biotic and abiotic stresses, from drought tolerance to fungal infection.Citation11,12) However, hitherto, no heme oxygenase activity has been shown in algae. In this study, we performed functional and expression analyses of the Chlorella caleosin through cDNA cloning, characterization of the enzymatic activity, and transcription analysis. Our results indicate that a fresh water Chlorella vulgaris TISTR 8580 contains a salt induced heme protein caleosin.
Materials and methods
Strains and growth conditions
Escherichia coli strain DH5α cells were grown in Luria-Bertani medium at 37 °C. C. vulgaris TISTR 8580 cells were grown photoautotrophically (120 μE m−2 sec−1) in Blue Green (BG) 11 liquid medium containing 18 mM NaNO3 at 30 °C.Citation13) The growth of E. coli and algae cells was monitored by measuring the absorbance at 620 and 730 nm, respectively, with a Shimadzu UV-160A spectrophotometer.Citation14)
Stress treatment
C. vulgaris cells were grown in the growth medium photoautotrophically (normal growth light 120 μE m−2 sec−1) until OD730 reach 0.4 prior to the up-shock and nitrogen deficient experiments. For up-shock experiment, the concentration of NaCl in growth medium was changed from 0.0 to 0.3 M and the growth was carried out for the indicated time. For nitrogen deficient experiment, NaNO3 in the culture medium was removed and the growth was carried out for the indicated time.
Cloning of caleosin gene from C. vulgaris TISTR 8580
Total RNA was extracted using RNeasy Plant Mini Kit (QIAGEN Inc., CA, USA). The cDNA was synthesized from total RNA (1 µg) using reverse transcription enzyme M-MLV (Takara, Shiga, Japan). A fragment of caleosin gene was amplified using the mixed primers, Clom-F 5-ACMKSACGGTSMTGCAGCAGCA-3 and Clom-R 5-TTYTCSGGSACAAARC GVCCCTC-3. Amplified fragment was ligated into pCR2.1 vector and sequenced. The full-length cDNA was obtained by RACE method using the primer set, CvClo-F3 5-ACCGCGACAACGACGGTGTGCTG-3 and CvClo-R5 5-CCAGGAGAAGGTGCCGTG GATGAC-3.
Expression of caleosin gene in E. coli
For the construction of an expression vector in E. coli, the regions containing coding region were PCR amplified using the primer pairs, CvClo-F 5′-ACATATGG TGTCGGCTTCCCAGATG-3’ and CvClo-R 5′-AGAATTCTCACTCCTCCTTGGCCCC TGCC-3, and thereby incorporating the 5′-NdeI and 3′-EcoRI restriction sites. The PCR product was cloned into pCR2.1 vector and sequenced to exclude PCR errors. The full-length caleosin fragment was prepared by double digestion with NdeI and EcoRI and ligated into the corresponding sites of pCold I vector (Takara, Shiga, Japan). The expression construct was transformed first into E. coli strain DH5α and then strain BL21.
RT-PCR analysis
Total RNA was extracted from C. vulgaris cells using the RNeasy kit (QIAGEN, Hilden, Germany). Five μg of the total RNA was reverse transcribed using the Superscript II RT kit (Invitrogen, CA, USA) as per the manufacturer’s instructions. The PCR amplification was performed using primer sets CvClo-F3 5-ACCGCGACAACGACGGTGTGCTG-3 and CvClo_PCR-R 5-TTAAAGACGTCAACCCGCCA-3 for Chlorella caleosin and CvrRNA-F 5-GGAGCCTGCGGCTTAATTTG-3 and CvrRNA-R 5-TCGCCAATCCGAACACTTCA-3 for 18s rRNA as a positive control. The PCR-amplified fragments were electrophoresed on 1.2% (w/v) agarose gels and stained with 0.1 μg/ml ethidium bromide.
Peroxidase activity
E. coli cells were harvested by centrifuge at 15,000 × g. The pellets were suspended in 0.1 M Tris–HCl (pH 8.0) and sonicated by INSONATOR 201 M (Kubota, Tokyo) at 4 °C for 10 min and centrifuged at 4000 × g for 15 min. The supernatant was used for the measurement of activity. The reaction mixture contained 1 M phosphate buffer (pH 7.2), 1 mM aniline, and 0.5 mg soluble protein.Citation10) The reaction was started by the addition of 0.1 mM cumene hydroperoxide. Peroxidase activity was monitored by measuring the absorbance change at 310 nm. For the measurement of changes of absorbance around 450–350 nm region (Soret band), 0.7 mg soluble protein was added.
Fluorescent microscopy
Stock solution of Nile red (5 mg/mL) (Sigma-Aldrich, USA) was prepared in acetone. The staining was performed by mixing the solution of the dye directly with the cultured suspension medium in 1:50 ratio and incubated for 30 min at room temperature. Cells were observed using Olympus BX53 microscopy equipped with 100 W high pressure mercury lamp, GFP fluorescence filter set. Pictures were acquired with DP-73 camera (Olympus Co., Tokyo, Japan).
Other methods
SDS-PAGE and Western blot analyses were performed as previously described.Citation15) Protein concentration was determined by Bradford method.Citation15) Protein bands on SDS-PAGE were detected with Coomassie Brilliant Blue (CBB R-250) stain. For Western blot analysis, SDS-PAGE-separated protein bands were transferred to a PVDF membrane. The anti-6-His (6x-His tag) antibody was purchased from R&D systems (Minneapolis, MN, USA).
Results and discussion
Accumulation of triacylglycerol was induced under salt-stressed condition
Hitherto, effects of salt stress on the accumulation of triacylglycerol in Chlorella have not been reported. We compared the effects of salt stress (0.3 M NaCl) and nitrogen deficiency on the accumulation of triacylglycerol in C. vulgaris TISTR 8580 cells. Fig. (A) shows the growth curves of C. vulgaris TISTR 8580 under normal, salt stressed and nitrogen deficient conditions. Both salt stress and nitrogen deficiency decreased the growth rate. But nitrogen deficiency decreased more severely than that of salt stress (Fig. (A)). Fig. (B) shows that the Nile red fluorescence of C. vulgaris TISTR 8580 was very faint or undetectable before stress. After 24 h of salt stress (0.3 M NaCl), significant Nile red fluorescence was observed (Fig. (B)). Its fluorescence was further increased after 48 h salt stress. These data clearly indicate that the accumulation of triacylglycerol was induced under salt-stressed conditions. The levels of triacylglycerol are also increased under nitrogen deficient conditions (Fig. (B)). Compared with nitrogen deficient conditions, the induction by salt stress was more significant (Fig. (C)).
Fig. 1. Accumulation of triacylglycerols in Chlorella cells during NaCl stress and N limitation.
Note: (A) Growth curves, (B) photograph of Nile red stained cells, and (C) fluorescence intensity of Nile red. C. vulgaris TISTR 8580 cells were grown photoautotrophically in BG11 liquid medium containing 18 mM NaNO3 at 30 °C until OD730 reach 0.4. For salt stress, the concentration of NaCl in growth medium was changed from 0.0 to 0.3 M and the growth was carried out for the indicated time. For nitrogen deficient experiment, NaNO3 in the culture medium was removed and the growth was carried out for the indicated time. (A) Growth of Chlorella cells was monitored by the absorbance at 730 nm. Control (open circle), normal BG11 media; (B) salt stress (open square), BG11 media containing 0.3 M NaCl; nitrogen deficiency (open triangle), nitrogen-free BG11 media. Error bars show standard errors (SE) and (C) fluorescence intensity of Nile red was quantified using ImageJ software. Data (the means ± SD) of three independent experiments.
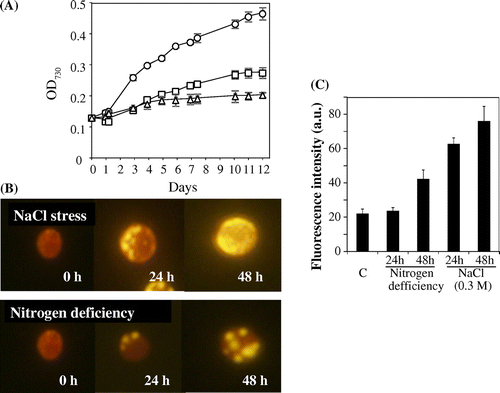
Cloning of putative caleosin gene from C. vulgaris TISTR 8580
A cDNA fragment encoding the putative Chlorella caleosin was obtained by PCR cloning using a primer set which was designed according to a highly conservative region among diverse caleosins. Then, a full-length cDNA fragment (accession No. LC003232) was obtained by both 5′- and 3′-RACE methods. It contains 1024 nucleotides, comprising an untranslated 5′-region of 34 nucleotides, an open reading frame of 840 nucleotides, and an untranslated 3′-region of 150 nucleotides. The deduced polypeptide comprises 279 amino acid residues with a molecular mass of 31,047 Da. Fig. (A) shows that the Chlorella caleosin comprises three structural domains: an N-terminal hydrophilic calcium-binding domain, a central hydrophobic oil-body anchoring domain, and a C-terminal hydrophilic domain. It was found that the insertion of 19 amino acid residues occurs in C. vulgaris TISTR 8580 cells. The insertion of 19 amino acid residues could not be found in any caleosins from plants as well as in other algae such as Chlamydomonas and A. protothecoides. We found one example in the genome sequenced C. variabilis NC64A.Citation16) Therefore, this insertion seems to be not due to the artifact of the sequencing. Physiological meaning and functional properties of the insertion remain to be clarified.
Fig. 2. Amino acid sequence and phylogenetic trees of caleosins.
Notes: (A) Sequence of caleosin from C. vulgaris TISTR 8580. Boxed His76 and His144 are the putative histidines which are involved in the histidine-dependent peroxygenase activity. Underline shows the putative calcium-binding domain region. Amino acids boxed by broken lines indicate the unique insertion. Stars show the amino acids involved in putative proline-knot motif, and (B) Phylogenetic tree-A phylogenetic tree was constructed using an alignment of full-length protein sequences generated using ClustalW. Protein sequences are from TISTR (CvCLO), AtCLO1 (At4g26740), AtCLO3 (At2g33380), AtCLO4 (At1g70670), AtCLO5 (At1g23240), AtCLO6 (At1g70680), AtCLO7 (At1g23250), Auxenochlorella (AEB77763), moss (XP001765592), cycad (FJ455154), Coccomyxa1 (EIE19761.1), Coccomyxa2 (EIE19762.1), Volvox (XP_002958325.1), NcPXG (Neurospora crassa, Q7S2T2), MgPXG (Magnaporthe grisea, XP_365887), and UmPXG (Ustilago maydis, Q4PAW0).
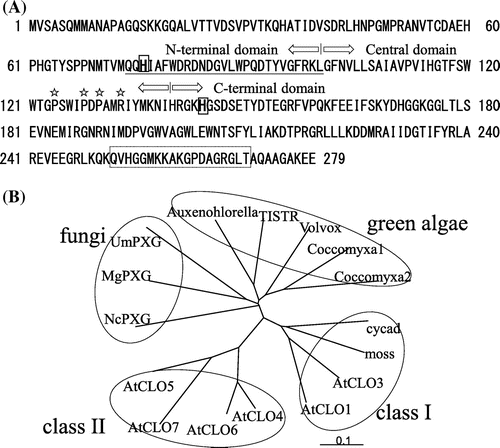
Phylogenetic tree analysis of the examined caleosin protein sequences shows that the class I caleosin contains AtCLO1, AtCLO3, moss, and cycad caleosins (Fig. (B)). Class II caleosin contains AtCLO4 and AtCLO5. Fungi are another class (Class III). Green algae caleosins are more similar to fungi caleosins. TISTR Chlorella caleosin exhibited the highest similarity with that from A. protothecoides (54% identity), then other algae (Cocomyxa, Volvox) (47–48% identity), fungi (37–42% identity), and plants (37% identity).
It has been reported that AtCLO1 and AtCLO4 are heme oxygenase with an EF-hand calcium-binding motif.Citation10,11) The amino acid alignment shows that Chlorella caleosin contains EF-hand motif (Fig. (A) and Supplemental Fig. S1). The amino acids that involved in Ca2+ binding at the X, Y, and Z positions are completely conserved among single cell alga and fungi, but different from Arabidopsis ones (AtCLO1 and AtCLO4). In Arabidopsis, AtCLO1 has a calcium-dependent heme oxygenase activity that is regulated by two conserved ferric-binding histidine residues.Citation10) These histidines are His76 and His134 in Chlorella caleosin (Fig. (A)) which were completely conserved among plant, fungi, and algae (Supplemental Fig. S1A). Proline rich region was also conserved although it is weak as shown in Supplemental Fig. S1B. Interestingly, five amino acid residues 138-KHGSD-142 in Chlorella were completely conserved (Supplemental Fig. S1(B)). Since this region is just after the second His135, this might play an important role for the heme oxygenase activity.
Expression of caleosin gene in E. coli
The plasmid pCold I was used to transform E. coli BL21. The His-tag sequence was introduced to the N-terminal of Chlorella caleosin. After addition of IPTG, significant amount of soluble protein was detected at 32 kDa on SDS-PAGE (Fig. (A)). Western blotting confirmed the band was the Chlorella caleosin (Fig. (B)).
Fig. 3. Expression of caleosin from C. vulgaris TISTR 8580 in E. coli cells.
Notes: Cells were harvested, sonicated and centrifuged. Soluble fraction and pellet fractions were obtained. (A) SDS-PAGE of cell lysate and supernatant, (B) Western blotting of supernatant. Antibody against His-tag was used for immunoblotting.
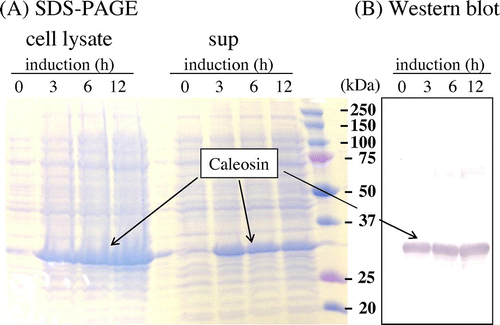
Previously, it was shown that SDS-PAGE of AtCLO1 and AtCLO4 exhibited a minor protein band with molecular mass of about double in addition to monomer band, and it was suggested that AtCLO1 and AtCLO4 exist as dimer as well as monomer.Citation10,11) Therefore, oligomerization of Chlorella caleosin was examined using native PAGE. When acrylamide concentration of PAGE was 12.5%, Chlorella caleosin migrated as a protein of which molecular mass was larger than 100 kDa (Fig. ). When the acrylamide concentration was decreased to 7.5%, its molecular mass was about 350 kDa. A protein band corresponding to monomer was hard to be detected, suggesting that the recombinant Chlorella caleosin exists as oligomer in vitro. Molecular masses of proteins generally cannot be determined in native gels. The in vivo oligomerization state of Chlorella caleosin remains to be clarified.
Fig. 4. Oligomerization state of Chlorella caleosin.
Notes: Chlorella caleosin was expressed in E. coli. After sonication and centrifugation, soluble fraction was obtained and applied to native PAGE. Left––the concentration of acrylamide was 12.5%. Right––the concentration of acrylamide was 7.5%. Caleosin proteins were detected by anti-His tag antibody.
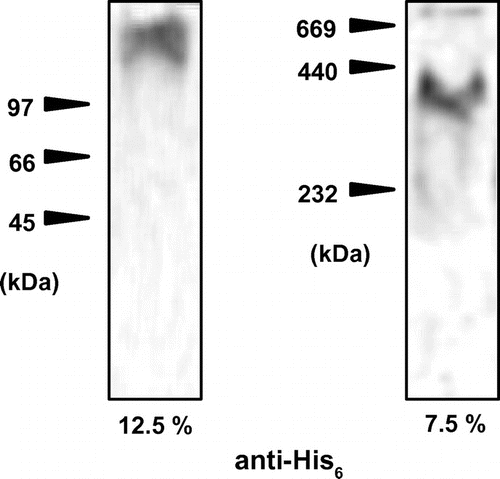
Chlorella caleosin is a heme-containing peroxygenase
It has been reported that Arabidopsis caleosins are heme oxygenase with an EF-hand calcium-binding motif.Citation10) Heme oxygenase activity of algae caleosin has never been reported. To examine its activity, Chlorella caleosin was expressed in E coli. Peroxygenase activity was assayed with aniline as a substrate.Citation10) As shown in Fig. (A), peroxidase activity was observed when Chlorella caleosin gene was expressed in E. coli, whereas E. coli transformed with empty vector did not show the activity.
Fig. 5. Peroxygenase activity of caleosin from C. vulgaris TISTR 8580.
Notes: Caleosin was expressed in E. coli. (A) Peroxidase activity, and (B) inactivation of peroxygenase activity. The peroxidase activity of caleosin was measured as described in materials and methods. In panel (B), the reaction mixture contained caleosin (0.7 mg) in 10 mM potassium buffer (pH 8). The Soret band of hemoproteins could be detected before addition of cumene hydroperoxide (solid line). The addition of cumene hydroperoxide (1 mM) in the absence of aniline caused the decrease of Soret band (dotted line) and inactivation. Data are the means ± SD) of three independent experiments.
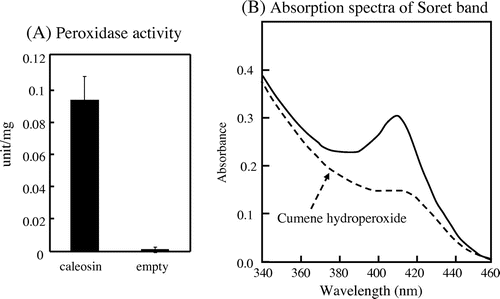
To test whether this peroxygenase activity was due to the heme protein, we examined the absorbance change in the Soret region. We could observe a peak at 407 nm region, representative of the Soret band of hemoprotein, in the absorbance spectra as shown in Fig. (B) (solid line). Addition of cumene hydroperoxide resulted in a gradual decrease of this Soret band (Fig. (B), dotted line). These results confirmed that Chlorella caleosin contains a heme that is responsible for peroxygenase activity.
Expression of caleosin gene is salt induced
Next, we examined the effect of nitrogen deficiency and salt stress on gene expression of caleosin in C. vulgaris TISTR 8580 cells. RT-PCR analysis revealed that the expression of caleosin gene was increased by 5.3-fold after 6 h of exposure of Chlorella cells to a high salinity medium containing 0.3 M NaCl (Fig. (A)). The expression of caleosin gene was increased by 4.3-fold after 6 h of depletion of nitrate (Fig. (B)). These results indicate that the expression of caleosin gene is induced upon nitrogen deficiency as well as salt stress.
Fig. 6. Expression of caleosin gene in C. vulgaris TISTR 8580 cells.
Notes: Cells were collected at 0, 6, and 12 h of exposure to salinity stress, and (A) nitrogen deficiency (B) Semi-quantitative RT-PCR analysis was performed as described in materials and methods. PCR products were subjected to electrophoresis, followed by calculation of relative values of the amount of DNA fragments. 18s rRNA gene was used as control whose mRNA abundance remained unchanged under stress conditions. The values at time zero of each gene were set to 1. Data are the means ± SD) of three independent experiments.
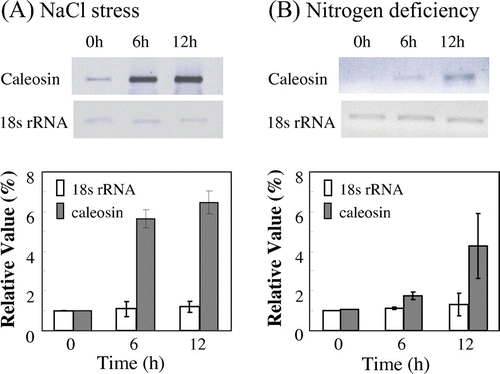
Conclusion
In this study, we isolated a putative caleosin gene from a fresh water C. vulgaris TISTR 8580. The isolated caleosin gene showed high similarity to the putative caleosin from genome sequenced algae and fungi, but less to type I and type II caleosin from plants. The mRNA contents for Chlorella caleosin significantly increased under salt stress condition. Caleosin gene was expressed in E. coli. Based on these data, we can conclude that C. vulgaris TISTR 8580 contains a salt inducible heme protein caleosin. In plants, it has been shown that the peroxygenase activities is involved in the oxylipin metabolites that play prominent roles in plant responses to a range of biotic and abiotic stresses, from drought tolerance to fungal infection.Citation11,12) The present data suggest the similar function of caleosin in Chlorella might occur, but has to wait the experimental evidence.
Induction of triacylglycerol has been shown by various environmental stresses such as nutrient stress (e.g. nitrogen, phosphate, and sulfur starvation), osmotic stress, radiation, pH, and temperature.Citation17) Under stress conditions, the pool of the major electron acceptor for photosynthesis, NADP+, can become depleted, leading to a dangerous situation for the cell. The increased synthesis of fatty acids (which in turn are stored as triacylglycerol) consumes NADPH and replenishes the pool of NADP+. The relevant enzymes and corresponding genes might be different depending on the applied stresses and microalgae species. The involvement of acyltransferase and nitrogen response regulator for the nitrogen starvation-induced triacylglycerol accumulation has been reported in the case of Chlamydomonas.Citation18) But, it is unclear for salt stress and remains to be clarified.
With the growing importance of algal feedstock for the production of biofuels and high-value lipids, the presented data provide the useful information for the study to produce the large scale of lipid in Chlorella.
Note
Nucleotide sequence data for Chlorella caleosin are available in the DDBJ databases under the accession number LC003232.
Supplemental material
The supplemental material for this paper is available at http://dx.doi.org/10.1080/09168451.2015.1010480.
Conflict of interest
We have no conflict of interest to declare.
Funding
This work was supported in part by Grants-in-Aid for Scientific Research from the Ministry of Education, Science and Culture of Japan and the International Research Center for Natural Environmental Science of Meijo University.
Notes
Abbreviations: Ap, Aphanothece halophytica; BG, Blue Green; CLO, caleosin; LB, Luria-Bertani; LD, lipid droplet; MLDP, major lipid droplet protein.
References
- Murphy DJ. The dynamic roles of intracellular lipid droplets: from archaea to mammals. Protoplasma. 2012;249:541–585.10.1007/s00709-011-0329-7
- Jiang PL, Tzen JT. Caleosin serves as the major structural protein as efficient as oleosin on the surface of seed oil bodies. Plant Signal. Behav. 2010;5:447–449.10.4161/psb.5.4.10874
- Moellering ER, Benning C. RNA interference silencing of a major lipid droplet protein affects lipid droplet size in Chlamydomonas reinhardtii. Eukaryot. Cell. 2010;9:97–106.10.1128/EC.00203-09
- Peled E, Leu S, Zarka A, Weiss M, Pick U, Khozin-Goldberg I, Boussiba S. Isolation of a novel oil globule protein from the green alga Haematococcus pluvialis (Chlorophyceae). Lipids. 2011;46:851–861.10.1007/s11745-011-3579-4
- Davidi L, Katz A, Pick U. Characterization of major lipid droplet proteins from Dunaliella. Planta. 2012;236:19–33.10.1007/s00425-011-1585-7
- Wang ZT, Ullrich N, Joo S, Waffenschmidt S, Goodenough U. Algal lipid bodies: stress induction, purification, and biochemical characterization in wild-type and starchless Chlamydomonas reinhardtii. Eukaryot. Cell. 2009;8:1856–1868.10.1128/EC.00272-09
- Huang NL, Huang MD, Chen TL, Huang AH. Oleosin of subcellular lipid droplets evolved in green algae. Plant Physiol. 2013;161:1862–1874.10.1104/pp.112.212514
- Lin IP, Jiang PL, Chen CS, Tzen JT. A unique caleosin serving as the major integral protein in oil bodies isolated from Chlorella sp. cells cultured with limited nitrogen. Plant Physiol. Biochem. 2012;61:80–87.10.1016/j.plaphy.2012.09.008
- Markou G, Nerantzis E. Microalgae for high-value compounds and biofuels production: a review with focus on cultivation under stress conditions. Biotechnol. Adv. 2013;31:1532–1542.10.1016/j.biotechadv.2013.07.011
- Hanano A, Burcklen M, Flenet M, Ivancich A, Louwagie M, Garin J, Blée E. Plant seed peroxygenase is an original heme-oxygenase with an EF-hand calcium binding motif. J. Biol. Chem. 2006;281:33140–33151.10.1074/jbc.M605395200
- Blée E, Flenet M, Boachon B, Fauconnier ML, Blée E, Flenet M, Boachon B, Fauconnier ML. A non-canonical caleosin from Arabidopsis efficiently epoxidizes physiological unsaturated fatty acids with complete stereo selectivity. FEBS J. 2012;279:3981–3995.10.1111/febs.2012.279.issue-20
- Shimada TL, Takano Y, Shimada T, Fujiwara M, Fukao Y, Mori M, Okazaki Y, Saito K, Sasaki R, Aoki K, Hara-Nishimura I. Leaf oil body functions as a subcellular factory for the production of a phytoalexin in Arabidopsis. Plant Physiol. 2014;164:105–118.10.1104/pp.113.230185
- Kageyama H, Tripathi K, Rai AK, Cha-Um S, Waditee-Sirisattha R, Takabe T. An alkaline phosphatase/phosphodiesterase, PhoD, induced by salt stress and secreted out of the cells of Aphanothece halophytica, a halotolerant cyanobacterium. Appl. Environ. Microbiol. 2011;77:5178–5183.10.1128/AEM.00667-11
- Waditee-Sirisattha R, Singh M, Kageyama H, Sittipol D, Rai AK, Takabe T. Anabaena sp. PCC7120 transformed with glycine methylation genes from Aphanothece halophytica synthesized glycine betaine showing increased tolerance to salt. Arch. Microbiol. 2012;194:909–914.10.1007/s00203-012-0824-z
- Waditee R, Tanaka Y, Aoki H, Hibino T, Jikuya H, Takano J, Takabe T, Takabe T. Isolation and functional characterization of N-methyltransferases that catalyze betaine synthesis from glycine in a halotolerant photosynthetic organism Aphanothece halophytica. J. Biol. Chem. 2003;278:4932–4942.10.1074/jbc.M210970200
- Blanc G, Duncan G, Agarkova I, Borodovsky M, Gurnon J, Kuo A, Lindquist E, Lucas S, Pangilinan J, Polle J, Salamov A, Terry A, Yamada T, Dunigan DD, Grigoriev IV, Claverie JM, Van Etten JL. The Chlorella variabilis NC64A genome reveals adaptation to photosymbiosis, coevolution with viruses, and cryptic sex. Plant Cell. 2010;22:2943–2955.10.1105/tpc.110.076406
- Sharma KK, Holger Schuhmann H, Schenk PM. High lipid induction in microalgae for biodiesel production. Energies. 2012;5:1532–1553.
- Boyle NR, Page MD, Liu B, Blaby IK, Casero D, Kropat J, Cokus SJ, Hong-Hermesdorf A, Shaw J, Karpowicz SJ, Gallaher SD, Johnson S, Benning C, Pellegrini M, Grossman A, Merchant SS. Three acyltransferases and nitrogen-responsive regulator are implicated in nitrogen starvation-induced triacylglycerol accumulation in Chlamydomonas. J. Biol. Chem. 2012;287:15811–15825.10.1074/jbc.M111.334052