Abstract
An NADPH-dependent sorbose reductase from Candida albicans was identified to catalyze the asymmetric reduction of ethyl 4-chloro-3-oxobutanoate (COBE). The activity of the recombinant enzyme toward COBE was 6.2 U/mg. The asymmetric reduction of COBE was performed with two coexisting recombinant Escherichia coli strains, in which the recombinant E. coli expressing glucose dehydrogenase was used as an NADPH regenerator. An optical purity of 99% (e.e.) and a maximum yield of 1240 mM (S)-4-chloro-3-hydroxybutanoate were obtained under an optimal biomass ratio of 1:2. A highest turnover number of 53,900 was achieved without adding extra NADP+/NADPH compared with those known COBE-catalytic systems.
Graphical Abstract
Asymmetric synthesis of (S)-4-chloro-3-hydroxybutanoate by an NADPH-dependent sorbose reductase (SOU1) in a two-strain co-existing system.
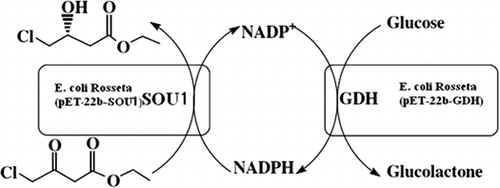
Ethyl (S)-4-chloro-3-hydroxybutanoate [(S)-CHBE] is a promising chiral intermediate in the synthesis of pharmacologically active compounds, such as hydroxymethylglutaryl-CoA reductase inhibitors and 4-hydroxypyrrolidone.Citation1) The asymmetric reduction of ethyl 4-chloro-3-oxobutanoate (COBE) by enantioselective oxidoreductases has been proved one of the most efficient methods for the production of (S)-CHBE.Citation2) However, two major limitations apply to the biotransformation process, namely, the preparation of high-performance oxidoreductase for COBE reduction and the efficient recycling of coenzymes NAD(P)+/NAD(P)H.
Several reductases have been reported to perform the (S)-CHBE synthesis, including CaCR from Candida albicans,Citation3) KaCR from Kluyveromyces aestuarii,Citation4) PsCRCitation5) and PsCRIICitation6) from Pichia stipitis, S1 from Candida magnoliae,Citation7) and CpCR from Candida parapsilosis.Citation8) Glucose dehydrogenase was coupled with all of these reductases for coenzyme regeneration because of its high stability and cheap substrate. The biocatalysts were usually obtained by coexpressing the reductases and glucose dehydrogenase in one strain, but the order of the genes, Shine-Dalgarno (SD) regions, or aligned spacing between the SD sequence and the translation initiation codon may affect the expression level of either enzyme.Citation9) In addition, the velocities of synthesis reaction and coenzyme regeneration may be difficult to regulate in a coexpression system.Citation10) Thus, a two-strain coexisting system was proposed based on the principle that the coenzyme regeneration system in one strain can supply the biosynthesis in another different strain.Citation11,12) The two-strain system is more flexible than the coexpression system because the balance between synthesis reaction and coenzyme regeneration can be easily achieved by adjusting the biomass of the two strains.
In this study, we reported an NADPH-dependent sorbose reductase (SOU1) from C. albicans to catalyze the reduction of COBE. Greenberg et al. Citation13) first reported SOU1 as an important reductase involved in sugar metabolism. However, the amino acid sequence analysis revealed that SOU1 shared high sequence homology with known COBE catalytic-enzymes. SOU1 exhibited a reduction activity toward COBE. Thus, a two-strain coexisting system for (S)-CHBE production was constructed using SOU1 and glucose dehydrogenase. The recombinant strain Escherichia coli Rosseta (pET-22b-SOU1) was mixed with E. coli Rosseta (pET-22b-GDH) expressing glucose dehydrogenase for both COBE reduction and coenzyme regeneration (Fig. ).
Materials and methods
Enzymes and chemicals
COBE, (R)-CHBE, and (S)-CHBE were obtained from Acros Organics. NADPH was supplied by Sigma-Aldrich Chemie GmbH. All restriction endonucleases, Taq polymerase, and T4 ligase were purchased from TaKaRa (Dalian) Co. All other chemicals used were of analytical grade purity and commercially available.
Strains and vectors
The gdh gene was cloned from Bacillus megaterium DSM 2894. The E. coli Rosseta (pET-22b-GDH) constructed earlier to produce recombinant GDH was used for the cofactor regeneration in (S)-CHBE biosynthesis.Citation3) C. albicans was preserved in our laboratory. E. coli Rosseta (DE3) and pET-22b were used for the overexpression of the recombinant SOU1.
Cloning and expression of the SOU1 gene
The genome of C. albicans was extracted using a TIANamp yeast DNA kit (TIANGEN, China). The SOU1 gene from C. albicans was amplified by PCR with designed primers of 5′-GGAATTCCATATGATGAGTGAAGAAATCATTTCA-3′ (NdeI site is underlined) and 5′-CCGGAATTCTTATGGACATGTATAACCCCCAT-3′ (EcoRI site is underlined). The PCR conditions were as follows: 35 cycles of 60 s at 94 °C, 30 s at 56 °C, and 60 s at 72 °C. The 846 bp DNA fragment was inserted into pET-22b, resulting in recombinant plasmid pET-22b-SOU1, with its structure verified by double-enzyme cleavage and gene sequence analysis. The recombinant E. coli Rosseta (DE3)/pET-22b-SOU1 was obtained by heat shock transformation.
The recombinant strains were grown to an optical density (OD 600 nm) of 0.6 at 37 °C and 200 rpm in LB medium containing 100 mg/L ampicillin and 20 mg/L chloramphenicol. The temperature was then changed to 30 °C, and expression was induced by 0.8 mM IPTG. After 10 h, the cells were harvested by centrifugation (5000 g, 5 min, 4 °C), washed with 100 mM potassium phosphate buffer (pH 6.2), and used for biotransformation.
Enzyme activity assay
Cells were disrupted ultrasonically in 100 mM potassium phosphate buffer (pH 6.2). The debris was removed by centrifugation at 13,000 g for 10 min. The supernatant was used as the cell-free extract. SOU1 activity was determined using 10 mM COBE with 1 mM NADPH, following changes in absorbance at 340 nm. The extinction coefficient of NADPH was 6.22 mM−1 cm−1. One unit of SOU1 was defined as the disappearance of 1 μmol NADPH per min. Protein concentration was measured by the Bradford method using bovine serum albumin as the standard. The repeatability was determined by three time measurements.
Bioconversion of COBE to (S)-CHBE
Cells were harvested by centrifugation (10,000 g, 20 min, 4 °C) and washed with 100 mM potassium phosphate buffer (pH 6.2). The bioconversion of COBE to (S)-CHBE was performed in an aqueous–butyl acetate (4:1, v/v) biphase system containing 100 mM potassium phosphate buffer (pH 6.2), 1500 mM COBE, 1550 mM glucose, 1550 mM Na2CO3, Triton X-100 (1‰, v/v), 0.1 g of dry cell weight (DCW) of E. coli Rosseta (pET-22b-SOU1), and E. coli Rosseta (pET-22b-GDH), respectively, at a total volume of 25 mL. The reaction was performed at 30 °C and 220 rpm for 5 h. The organic layer was isolated to determine the product concentration and optical purity.
To study the effect of cell mass ratio between E. coli Rosseta (pET-22b-SOU1) and E. coli Rosseta (pET-22b-GDH) on (S)-CHBE yield, we adjusted the mass ratio of the two strains under the same amount of total biomass, which was 200 mg of the gross dry cell weight in the same reaction system.
Analytical methods
The amount of COBE and CHBE was measured by gas chromatography and the optical purity of (S)-CHBE was determined by HPLC on a Chiralcel OB-H column (4.6 mm × 250 mm; Daicel Chemical Industries, Japan) as described previously.Citation9) The intracellular level of NADP+/NADPH was measured after extracting the reaction strain sample following a previously described method.Citation14)
Results
SOU1 activity toward COBE
SOU1 activity was determined using COBE as the substrate and NADPH as the electron donor. SOU1 exhibited an activity of 6.2 U mg−1 toward COBE. Meanwhile, the crude enzyme extract from control cells E. coli Rosseta (pET-22b) showed no activity on the substrate.
Bioconversion of COBE to (S)-CHBE with two coexisting E. coli strains
The SOU1 expressed in E. coli Rosseta (pET-22b-SOU1) functioned as main catalyst for the synthesis of COBE to (S)-CHBE, whereas the GDH expressed in E. coli Rosseta (pET-22b-GDH) catalyzed the reaction from NADP+ to NADPH to satisfy the needs of NADPH for the former reaction. Their DCW ratio was 1:1, and no NADP+ or NADPH was added in the mixture. After 5 h of bioconversion, the coexisting system produced 1050 mM of (S)-CHBE from 1500 mM COBE. The enantiomeric excess was over 99%.
Effect of cell mass ratio on (S)-CHBE yield
Given the differences between SOU1 activity toward COBE in E. coli Rosseta (pET-22b-SOU1) and the ability of NADPH regeneration catalyzed by E. coli Rosseta (pET-22b-GDH), the two-strain mass ratio was adjusted to establish favorable conditions for two enzymes functioning corporately in the reaction.
The bioconversion protocol was applied under the same amount of total biomass, except that different cell mass ratios of two strains were used. As shown in Fig. , the maximum yield of (S)-CHBE (1240 mM) was achieved when E. coli Rosseta (pET-22b-SOU1) and E. coli Rosseta (pET-22b-GDH) were added at a ratio of 1:2 after 5 h of biotransformation. The enantiomeric excess was more than 99%. The system performed poorly because of the low regeneration of NADPH without adding E. coli Rosseta (pET-22b-GDH).
Discussion
An NADPH-dependent SOU1 in C. albicans was identified to catalyze the reduction of COBE. SOU1 shared a high degree of similarity in three conserved segments with other COBE-catalytic reductases from SDR family. The recombinant SOU1 can catalyze the asymmetric reduction of COBE to (S)-CHBE with high activity and enantioselectivity.
The present work adopted a two-strain coexisting system for the production of (S)-CHBE. The SOU1 expressed in E. coli Rosseta(pET-22b-SOU1) catalyzed the biosynthesis of (S)-CHBE, whereas the GDH expressed in E. coli Rosseta(pET-22b-GDH) provided NADPH recycling. The system produced 1240 mM (S)-CHBE without adding extra NADP+/NADPH, and the e.e. value of (S)-CHBE was higher than 99%. The best production of (S)-CHBE was achieved under the mass ratio of 1:2 between SOU1 and GDH. A large amount of E. coli Rosseta (pET-22b-GDH) was required to maintain sufficient NADPH for the whole system, suggesting that the efficiency of NADPH regeneration is the major limiting factor for the production of (S)-CHBE.
Several reported biocatalytic processes for the synthesis of (S)-CHBE using GDH as cofactor regenerator were compared with this system (Table ). One of the advantages of our system is that NADP+/NADPH addition can be omitted. The internal cofactor of E.coli host cells is sufficient to start and maintain the reaction recycle. The turnover number of intracellular coenzyme to (S)-CHBE formed was 53,900 (mol/mol), which is higher than those known (S)-CHBE production systems. The space-time yield (31 mmol L−1h−1 g−1DCW) is the highest one and can compete against the one with crude enzyme reaction system (26.5 mmol L−1h−1 g−1DCW).Citation15) Moreover, the two strains would be cultivated individually at optimal culture conditions and mixed in an appropriate ratio to maximize the yield of (S)-CHBE production, making the process easy and flexible for practical operations.
Table 1. Comparison of several biocatalytic processes for the synthesis of (S)-CHBE.
Funding
This work was supported by the National Basic Research Program of China [grant number 2011CBA0807]; National High Technology Research and Development Program of China [grant number 2012AA022101]; Priority Academic Program Development of Jiangsu Higher Education Institutions [grant number 1001020B].
References
- Karanewsky DS, Badia MC, Ciosek CP Jr, Robl JA, Sofia MJ, Simpkins LM, DeLange B, Harrity TW, Biller SA, Gordon EM. Phosphorus-containing inhibitors of HMG-CoA reductase. 1. 4-[(2-arylethyl)hydroxyphosphinyl]-3-hydroxy-butanoic acids: a new class of cell-selective inhibitors of cholesterol biosynthesis. J. Med. Chem. 1990;33:2952–2956.10.1021/jm00173a007
- Ye Q, Ouyang P, Ying H. A review-biosynthesis of optically pure ethyl (S)-4-chloro-3-hydroxybutanoate ester: recent advances and future perspectives. Appl. Microbiol. Biotechnol. 2010;89:513–522.
- An MD, Cai P, Yan M, Hao N, Wang SS, Liu H, Li Y, Xu L. A novel reductase from Candida albicans for the production of ethyl (S)-4-chloro-3-hydroxybutanoate. Biosci. Biotechnol., Biochem. 2012;76:1210–1212.
- Yamamoto H, Mitsuhashi K, Kimoto N, Matsuyama A, Esaki N, Kobayashi Y. A novel NADH-dependent carbonyl reductase from Kluyveromyces aestuarii and comparison of NADH-regeneration system for the synthesis of ethyl (S)-4-chloro-3-hydroxybutanoate. Biosci. Biotechnol., Biochem. 2004;68:638–649.10.1271/bbb.68.638
- Ye Q, Yan M, Yao Z, Xu L, Cao H, Li Z, Chen Y, Li S, Bai J, Xiong J, Ying H, Ouyang P. A new member of the short-chain dehydrogenases/reductases superfamily: purification, characterization and substrate specificity of a recombinant carbonyl reductase from Pichia stipitis. Bioresour. Technol. 2009;100:6022–6027.10.1016/j.biortech.2009.06.014
- Ye Q, Cao H, Mi L, Yan M, Wang Y, He Q, Li J, Xu L, Chen Y, Xiong J, Ouyang P, Ying H. Biosynthesis of (S)-4-chloro-3-hydroxybutanoate ethyl using Escherichia coli co-expressing a novel NADH-dependent carbonyl reductase and a glucose dehydrogenase. Bioresour. Technol. 2010;101:8911–8914.10.1016/j.biortech.2010.06.098
- Yasohara Y, Kizaki N, Hasegawa J, Wada M, Kataoka M, Shimizu S. Molecular cloning and overexpression of the gene encoding an NADPH-dependent carbonyl reductase from Candida magnoliae, involved in stereoselective reduction of ethyl 4-Chloro-3-oxobutanoate. Biosci. Biotechnol., Biochem. 2000;64:1430–1436.10.1271/bbb.64.1430
- Kaliaperumal T, Kumar S, Gummadi SN, Chadha A. Asymmetric synthesis of (S)-ethyl-4-chloro-3-hydroxybutanoate using Candida parapsilosis ATCC 7330. J. Ind. Microbiol. Biotechnol. 2009;37:159–165.
- Ye Q, Cao H, Yan M, Cao F, Zhang Y, Li X, Xu L, Chen Y, Xiong J, Ouyang P, Ying H. Construction and co-expression of a polycistronic plasmid encoding carbonyl reductase and glucose dehydrogenase for production of ethyl (S)-4-chloro-3-hydroxybutanoate. Bioresour. Technol. 2010;101:6761–6767.10.1016/j.biortech.2010.03.099
- Kizaki N, Yasohara Y, Hasegawa J, Wada M, Kataoka M, Shimizu S. Synthesis of optically pure ethyl (S)-4-chloro-3-hydroxybutanoate by Escherichia coli transformant cells coexpressing the carbonyl reductase and glucose dehydrogenase genes. Appl. Microbiol. Biotechnol. 2001;55:590–595.10.1007/s002530100599
- Liu Y, Xu Z, Jing K, Jiang X, Lin J, Wang F, Cen P. Asymmetric reduction of ethyl 4-chloro-3-oxobutanoate to ethyl (R)-4-chloro-3-hydroxybutanoate with two co-existing, recombinant Escherichia coli strains. Biotechnol. Lett 2005;27:119–125.10.1007/s10529-004-7336-0
- Xu Z, Liu Y, Fang L, Jiang X, Jing K, Cen P. Construction of a two-strain system for asymmetric reduction of ethyl 4-chloro-3-oxobutanoate to (S)-4-chloro-3-hydroxybutanoate ethyl ester. Appl. Microbiol. Biotechnol. 2006;70:40–46.10.1007/s00253-005-0037-3
- Greenberg JR, Price NP, Oliver RP, Sherman F, Rustchenko E. Candida albicans SOU1 encodes a sorbose reductase required for L-sorbose utilization. Yeast. 2005;22:957–969.10.1002/(ISSN)1097-0061
- Zhang Z, Yu J, Stanton RC. A method for determination of pyridine nucleotides using a single extract. Anal. Biochem. 2000;285:163–167.10.1006/abio.2000.4701
- You ZY, Liu ZQ, Zheng YG. Characterization of a newly synthesized carbonyl reductase and construction of a biocatalytic process for the synthesis of ethyl (S)-4-chloro-3-hydroxybutanoate with high space-time yield. Appl. Microbiol. Biotechnol. 2013;98:1671–1680.
- Michihiko Kataoka, Akiko Hoshino-Hasegawa, Rungruedee Thiwthong, Nanami Higuchi, Takeru Ishige, Shimizu S, Gene cloning of an NADPH-dependent menadione reductase from Candida macedoniensis, and its application to chiral alcohol production. Enzyme Microb. Technol. 2006;38:944–951.10.1016/j.enzmictec.2005.08.029
- Ye Q, Cao H, Zang G, Mi L, Yan M, Wang Y, Zhang Y, Li X, Li J, Xu L, Xiong J, Ouyang P, Ying H. Biocatalytic synthesis of (S)-4-chloro-3-hydroxybutanoate ethyl ester using a recombinant whole-cell catalyst. Appl. Microbiol. Biotechnol. 2010;88:1277–1285.10.1007/s00253-010-2836-4