Abstract
Lipid-derived electrophilic molecules are endogenously generated and are causally involved in many pathophysiological effects. Prostaglandin D2, a major cyclooxygenase product in a variety of tissues and cells, readily undergoes dehydration to yield the J-series PGs such as 15-deoxy-Δ12,14-PGJ2 (15d-PGJ2). Because of the electrophilic α,β-unsaturated ketone moiety present in its cyclopentenone ring, 15d-PGJ2 acts as an endogenous electrophile. 15d-PGJ2 can covalently react via the Michael addition reaction with critical cellular nucleophiles, such as the free cysteine residues of proteins that play a key role in the regulation of the intracellular signaling pathways. Covalent modification of cellular proteins by 15d-PGJ2 may be one of the most important mechanisms by which 15d-PGJ2 induces many biological responses involved in the pathophysiological effects associated with inflammation. This current review is intended to provide a comprehensive summary of 15d-PGJ2 as an endogenous electrophilic mediator of biological activities.
Graphical Abstract
15-Deoxy-Δ12,14-prostaglandin J2 (15d-PGJ2), a cyclopentenone-type PG metabolite of PGD2, acts as an endogenous electrophilic mediator.
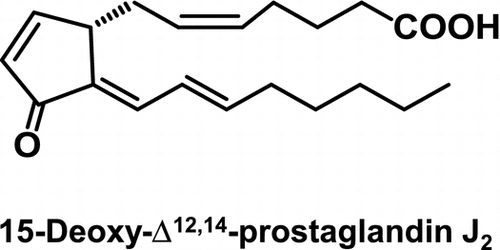
The prostaglandins (PGs) are a family of structurally related lipid mediators that are produced by cells in response to a variety of extrinsic stimuli and regulate differentiation, cellular growth, and homeostasis.Citation1,2) PGs are derived from fatty acids, primarily arachidonic acids, which are released from membrane phospholipids by phospholipases. Arachidonic acid is first converted to an unstable endoperoxide intermediate by cyclooxygenase (COX) and subsequently converted into one of several related products, including Prostaglandin D2 (PGD2), PGE2, PGF2α, prostacyclin, and thromboxane A2, through the action of specific PG synthases. Among them, PGD2 is one of the major COX products in a variety of tissues and cells and has significant effects on a number of biological processes, including relaxation of vascular and nonvascular smooth muscles, platelet aggregation, and nerve cell functions.Citation3) PGD synthase (PGDS) catalyzes the isomerization of the 9,10-endoperoxide group of PGH2, a precursor of PGs, to produce PGD2. Two distinct types of PGDS have been identified; that is, the hematopoietic-type PGDS and lipocalin-type PGDS. Although the lipocalin-type PGDS is expressed in the central nervous system and male genital organs of various mammals, the hematopoietic-type PGDS is widely expressed in the peripheral tissues as well as in antigen-presenting cells, megakaryocytes, and mast cells.Citation4) The PGs are physiologically present in body fluids in a picomolar-to-nanomolar concentration range,Citation5) however, arachidonic acid metabolism is significantly increased under various stimuli and several pathological conditions, including infection, hyperthermia, and inflammation,Citation6) and local concentrations of PG in the micromolar range have been detected at sites of acute inflammation.Citation7)
In the early 1980s, it was discovered that PGD2 readily undergoes dehydration in vivo and in vitro to yield biologically-active PGs of the J2 series, such as PGJ2, Δ12-PGJ2, and 15-deoxy-Δ12,14-PGJ2 (15d-PGJ2).Citation8,9) One of the most important findings regarding the cyclopentenone PGs is that 15d-PGJ2 acts as a ligand for the nuclear receptor, the peroxisome proliferator-activated receptor γ (PPARγ), a member of the nuclear receptor superfamily and a ligand-activated transcription factor with pleiotropic effects on glucose homeostasis, adipocyte differentiation, and lipid metabolism.Citation10,11) In addition, cyclopentenone-type PGs, unlike other classes of PGs, characterized by the presence of an electrophilic α,β-unsaturated ketone moiety in the cyclopentenone ring, have their own unique spectrum of biological activities. The reactive center of the cyclopentenone-type PGs has been proposed to account for some of their receptor-independent biological activities.Citation12,13) These PGs can act as a Michael addition acceptor and readily react with critical cellular nucleophiles, such as the free thiol group of the glutathione and cysteine residues in cellular proteins that play an important role in the regulation of the redox signaling pathways.Citation12–14) In the current review, we provide a comprehensive summary of 15d-PGJ2 as a lipid-derived endogenous electrophilic mediator and illustrate some recent approaches to establish the involvement of 15d-PGJ2 as a possible electrophilic mediator of cellular response in the pathogenesis of inflammatory disorders.
I. Formation of 15d-PGJ2
Fitzpatrick and WynaldaCitation8) first reported the in vitro formation of 15d-PGJ2. They have shown that human serum albumin (HSA) catalyzes the in vitro transformation of PGD2 into three dehydration products, 15d-PGD2, Δ12-PGJ2, and 15d-PGJ2. To establish a mechanism of transformation of PGD2 into the cyclopentenone-type PGJ2 derivatives, we developed a chiral-phase high-performance liquid chromatography method for separation of the PGD2 metabolites. Using this method, we investigated the conventional PGD2 metabolic pathway in detail and established a revised pathway of the PGD2 metabolism: (i) PGJ2 is directly converted into 15d-PGJ2 in an HSA-independent manner, (ii) PGJ2 is stoichiometrically converted into Δ12-PGJ2 in an HSA-dependent manner, and (iii) both 15d-PGD2 and Δ12-PGJ2 are not converted into 15d-PGJ2 (Fig. ). Citation15) It has been speculated that the formation of the α,β-unsaturated ketone in the cyclopentenone moiety of PGJ2 facilitates the Δ12 isomerization followed by dehydration of the hydroxyl group at C15 to generate 15d-PGJ2.
II. Immunochemical detection of 15d-PGJ2
15d-PGJ2 is believed to be physiologically produced through the nonenzymatic conversion of PGD2. However, it has never been definitively proven to exist in vivo. In view of its biological significance, it is crucial to provide evidence that 15d-PGJ2 is endogenously produced in vivo. To this end, we tried to develop a monoclonal antibody specific for 15d-PGJ2.Citation15) The monoclonal antibody (mAb11G2) was raised against the 15d-PGJ2-keyhole limpet hemocyanin conjugate and was found to specifically recognize the free 15d-PGJ2.
To examine whether 15d-PGJ2 is produced in response to inflammatory stimuli in vitro, we performed immunocytochemical experiments using the antibody mAb11G2 on the lipopolysaccharide (LPS)-stimulated mouse macrophage-like cell line RAW264.7 cell and found that exposure of the cells to LPS resulted in the appearance of the 15d-PGJ2 immunoreactivity in essentially all cells.Citation15) Similar results were also obtained in the cells treated with carrageenan and interleukin.Citation16–18) Using this antibody mAb11G2, we also examined the in vivo presence of 15d-PGJ2 in regions of atherosclerosis, which is considered to be a form of chronic inflammation and found that the intense immunoreactivities for COX-2 and 15d-PGJ2 were localized in the cytoplasm of the foamy macrophages.Citation15) These findings verified for the first time the intracellular accumulation of 15d-PGJ2 in vivo. In addition, the 15d-PGJ2 immunoreactivities were also detected in the spinal cord of sporadic amyotrophic lateral sclerosis patients.Citation19) These immunochemical studies with the antibody mAb11G2 showed that extracellular and intracellular 15d-PGJ2 could function as both paracrine and autocrine factors, respectively. These findings also suggested that the COX-2 up-regulation, through its pivotal role in inflammatory responses, followed by the enhanced intracellular production of 15d-PGJ2 might be ubiquitously involved in inflammation and its related diseases. Because PGD2 is one of the major PGs in most tissues, these cyclopentenone-type PGs are likely to be produced at a number of sites and may reach functionally significant levels in inflammatory processes.
III. 15d-PGJ2 as an electrophile
Some of the biological effects of 15d-PGJ2 are suggested to be mediated through its covalent modification of cellular target proteins. This is due to the reactive α,β-unsaturated ketone structure of 15d-PGJ2, which readily reacts with biological substances possessing a nucleophilic moiety such as the free cysteine residues of proteins via Michael addition reactions (Fig. ). On the basis of the observations that (i) 9,10-dihydro-15d-PGJ2, a 15d-PGJ2 analog lacking the endocyclic double bond, could not reproduce many biological activities of 15d-PGJ2Citation20–22) and that (ii) the site-directed mutation of cysteine residues in the target protein substantially abolishes the effect of 15d-PGJ2Citation12,23–25) the electrophilic moiety has been proposed to account for some of the receptor-independent biological activities of 15d-PGJ2. Interestingly, Shiraki et al.Citation23,24) reported the precise dynamic events that occur during ligand-binding and PPARγ-activation processes. The carbon at position 13, but not position 9, of 15d-PGJ2 reacts preferentially with the sulfur atom of the cysteine residue in ligand-binding domain of PPARγ. Thus, the mode of covalent binding of 15d-PGJ2 to the PPARγ appears to be different from that employed in PPARγ-independent actions.
IV. Antioxidant response
Elevation of the activities of the phase 2 detoxification enzymes provides protection against neoplasia. The electrophile-processing phase 2 enzymes, such as glutathione S-transferases (GSTs), are induced by a variety of chemical substances, including Michael addition acceptors.Citation26) The transcriptional activation of the phase 2 enzyme genes by the electrophiles has been traced to the antioxidant response element (ARE), a cis-acting transcriptional enhancer found in the 5ʹ-flanking region of the genes encoding many phase 2 enzymes.Citation27,28) Using a cell-based assay system that potently responds to the phenolic antioxidants resulting in the induction of the GST activity, Kawamoto et al.Citation29) first reported that 15d-PGJ2 is a strong inducer of the phase 2 response. Later, several studies have established that 15d-PGJ2 induces the phase 2 response via the Kelch-like ECH-associating protein 1 (Keap1)-nuclear factor-erythroid 2-related factor 2 (Nrf2) pathway.Citation17,18,30) It was first proposed that the inducers disrupt the Nrf2-Keap1 complex by oxidizing/modifying the cysteine residues of Keap1,Citation31) allowing Nrf2 to translocate to the nucleus, heterodimerize with the small Maf, and bind to ARE, eventually resulting in transcriptional activation of the phase 2 genes. More recently, however, several studies have shown that the electrophile modification of Keap1 inhibits ubiquitin conjugation to Nrf2 by the Keap1, an adaptor of the Cullin3-type ubiquitin ligase E3, thereby provoking the opening of the Keap1 gate and resulting in the nuclear accumulation of Nrf2.Citation32) The high cysteine content of Keap1 has suggested that it is a promising candidate as the sensor for 15d-PGJ2. Among the several candidate cysteines, Cys273 and Cys288 were first proposed to be critical for exposure to the electrophilic inducers. Meanwhile, based on other studies, Cys151 has been also suggested to be involved in the sensing system of electrophilic mediators.Citation33,34)
V. Induction of redox alteration
Kondo et al.Citation35) examined the oxidized fatty acid metabolites for their ability to induce intracellular reactive oxygen species (ROS) production in the human neuroblastoma cell line SH-SY5Y and found that the J2 series of the PGs represent the most potent inducers. Based on the observations that (i) the intracellular GSH levels were partially reduced by 15d-PGJ2, (ii) the 15d-PGJ2 treatment of the cells resulted in a significant decrease in the glutathione peroxidase activity, and (iii) the N-acetyl-L-cysteine pretreatment strongly reduced both the intracellular oxidative stress and cytotoxic effect by 15d-PGJ2, the intracellular redox status appeared to represent a crucial factor for the 15d-PGJ2-induced ROS production and cytotoxic effects. Moreover, the intracellular oxidative stress induction was accompanied by the change in the cellular redox conditions and the production of highly toxic aldehydic molecules, including 4-hydroxy-2-nonenal and acrolein.Citation35) Based on these observations, it has been suggested that the intracellular oxidative stress constitutes a pivotal step in the pathway of the cellular dysfunction induced by lipid-derived electrophiles such as 15d-PGJ2.
The intracellular oxidative stress induced by 15d-PGJ2 is associated with the production and accumulation of oxidatively modified proteins. Using the biotin-labeled hydrazide, Ishii et al.Citation36) determined the protein carbonyl generated in the 15d-PGJ2-treated SH-SY5Y cells and found that protein carbonylation specifically occurred on S6 ATPase, a regulatory subunit of 19 S proteasome. They also demonstrated (i) a significant increase in protein carbonylation within the S6 ATPase subunit, (ii) a dramatic decrease in the activities of S6 ATPase, and (iii) a decreased activity of the 26 S proteasome to degrade the substrates. In addition, the proteomic approach using the biotin-labeled cysteine, a probe for the cysteine-targeted oxidation of protein, revealed the 15d-PGJ2-induced oxidation of a range of proteins involved in various biological processes, including redox enzymes, glycolytic enzymes, chaperone protein, and cytoskeletal proteins.Citation37) The observation that oxidation occurs in specific proteins may indicate that this oxidative modification may serve a regulatory role, rather than a simple function in the protection of free thiol groups in proteins against irreversible oxidation.
15d-PGJ2 may alter the cellular redox status by its direct modification to cause the functional impairment of proteins involved in the regulation of intracellular antioxidant enzymes by direct modifications. Thioredoxin (Trx) and thioredoxin reductase (TrxR) play an important role in cell defense against oxidative stress. Indeed, it was observed that the 15d-PGJ2-induced oxidative stress and cell death were significantly suppressed by the Trx overexpression.Citation22) Using a biotinylated 15d-PGJ2, which retains the α,β-unsaturated ketone substituent and the electrophilic β-carbon of 15d-PGJ2, we found that 15d-PGJ2 directly modifies the endogenous Trx in intact cells.Citation22) Mass spectrometric studies revealed that (i) the 15d-PGJ2-treated Trx demonstrates the modification of one molecule of 15d-PGJ2 per Trx molecule and (ii) two cysteine residues, Cys35 and Cys69, are targets of 15d-PGJ2. In addition, Moos et al.Citation38) showed that the cyclopentenone-type PG modifies and inactivates the TrxR. These results suggest that the Trx and TrxR modification by 15d-PGJ2 may be one of the molecular mechanisms by which 15d-PGJ2 induces intracellular oxidative stress and cell death (Fig. ).
VI. Protein turnover
Proteasome has a very important role to play in regulating cellular processes, such as the cell cycle and metabolism, through the signal-mediated proteolysis of key enzymes and regulatory proteins.Citation39,40) It also operates during stress response by removing abnormal proteins and in the immune response by generating antigenic peptides. We found that 15d-PGJ2 significantly induced the accumulation of ubiquitinated proteins, whereas 9,10-dihydro-15d-PGJ2 had no significant effects on the turnover of these proteins.Citation41) 15d-PGJ2 induced the formation of the 15d-PGJ2-proteasome conjugatesCitation41) and oxidation of the S6 ATPase subunit of the 26S proteasomeCitation36) in the SH-SY5Y cells treated with 15d-PGJ2. These modifications could contribute to inhibition of the proteasome activity. Moreover, it has been showed that the cyclopentenone-type PG inhibits the ubiquitin-proteasome pathway via a decrease in the ubiquitin isopeptidase activity.Citation42) Wang et al.Citation43) examined the proteasome activity by an in-gel assay and showed a PGJ2-dependent decline in the proteasome activity. This decline paralleled a shift in the 26 S proteasome to the 20 S core particle, indicating that PGJ2 disrupted the assembly state of the 26 S proteasomes. These observations that 15d-PGJ2 inhibits the proteasome system provide a mechanism independent of the Keap1 modification that could explain the ARE activation. This may lead to an indirect action of 15d-PGJ2 as a trigger of the phase 2 responses. Moreover, substrates of proteasome include a number of cell regulatory proteins, such as p53; the controlled degradation of these molecules has been linked to the regulation of cell cycle progression and cell proliferation.Citation39,40) Thus, it is likely that the 15d-PGJ2-induced disruption of the ubiquitin-proteasome system results in the buildup of not only antioxidant response impairment, but also pro-apoptotic molecules, such as p53, and ubiquitinated proteins (Fig. ).
Fig. 4. Schematic mechanism of p53-dependent apoptosis induced by 15d-PGJ2.
Notes: 15d-PGJ2 activates p53 pathway through covalent modification of ATM, a protein kinase that phosphorylates p53, and thereby induces p53-dependent apoptotic cell death. 15d-PGJ2 also inhibits the degradation of p53 protein via covalent modification of proteasome.
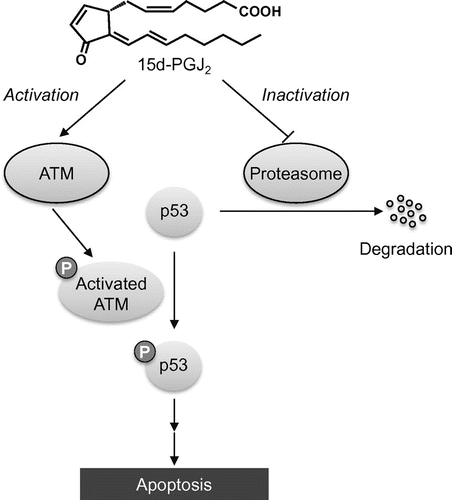
VII. Apoptosis induction
15d-PGJ2 is known to play a role in the resolution of inflammatory response by inducing apoptotic cell death of activated macrophages.Citation44) Kondo et al.Citation19) investigated the molecular mechanisms involved in the 15d-PGJ2-induced neuronal cell apoptosis and found that the 15d-PGJ2 activates the p53-dependent pathway, leading to the induction of a death-inducing caspase cascade mediated by Fas/Fas ligand. In addition, 15d-PGJ2 has been reported to activate the ataxia-telangiectasia mutated (ATM), a protein kinase that activates p53 once it is phosphorylated by DNA damage, leading to p53 activation and apoptotic cell death (Fig. ).Citation45) Using the biotin-labeled 15d-PGJ2, they demonstrated that 15d-PGJ2 modifies with ATM, presumably through the alkylation of the ATM’s cysteine residues. Moreover, it has also been shown that (i) 15d-PGJ2 inhibits the G2-M phase progression via the down-regulation of the anti-apoptotic molecules including cyclin B1,Citation46) and (ii) 15d-PGJ2 induces the expression of death receptor 5 at both the mRNA and protein levels in human malignant tumor cells in a PPARγ-independent manner.Citation47)
VIII. Cytoskeletal dysfunction
Using a biotin-labeled analog of 15d-PGJ2, potential targets of 15d-PGJ2 have been identified in cultured cells.Citation48,49) Aldini et al.Citation50) identified β-actin as one of the major target proteins in the human neuroblastoma cell line SH-SY5Y. In addition, they found that (i) 15d-PGJ2 induces morphological changes and depolymerization of the actin filament network, (ii) using a mass spectrometric technique, 15d-PGJ2 reacts with the isolated G-actin in a 1:1 stoichiometric ratio and selectively modifies at Cys374 by Michael addition reaction, and (iii) the covalent modification by 15d-PGJ2 induces unfolding of the actin structure and, in particular, 15d-PGJ2 distorts actin subdomains 2 and 4 that define the nucleotide binding sites impeding the nucleotide exchange. It has also been reported that 15d-PGJ2 directly modifies to vimentin and induces morphologic changes in the vimentin filament network in mesangial cells.Citation48) In addition, Han et al.Citation51) reported that cyclopentenone PGs, such PGA2 and PGJ2, can interact with microtubules to inhibit their function like paclitaxel. These findings suggest that cyclopentenone-type PGs modify cytoskeletal proteins and may cause cytoskeletal derangement.
IX. Conclusion
Based on many reports regarding the detection of 15d-PGJ2 in human disorders, there is no doubt that the steady-state levels of 15d-PGJ2 increase under inflammation-associated pathophysiological conditions. Considerable progress has also been recently made toward understanding the molecular mechanisms of the biological effects of 15d-PGJ2. As described in the current review, cyclopentenone-type PGs, including 15d-PGJ2, could trigger diverse aspects of cellular responses, including detoxification, oxidative stress, and apoptosis induction, through redox-sensitive signaling mechanisms. However, there are many interesting questions remaining to be answered. Particularly, the physiological role of 15d-PGJ2 in vivo remains an intriguing issue. Because 15d-PGJ2 exerts its biological effects at least in part through the modification of cellular proteins, identification of the target molecules of 15d-PGJ2 may facilitate efforts to elucidate novel aspects of endogenous electrophilic mediators.
Acknowledgements
The work described in this review was conducted in the Laboratory of Food and Biodynamics, Graduate School of Bioagricultural Sciences, Nagoya University. I would like to express special thanks to Prof. Koji Uchida (Nagoya University) for his continued encouragement and fruitful discussion, which has been indispensable to the progress in this study. I am grateful to Prof. Toshihiko Osawa (Nagoya University, currently Aichi Gakuin University) for his guidance and valuable discussion. I would like to also thank the past and present members of our laboratory and all our collaborators for their considerable encouragement and generous cooperation.
Notes
Abbreviations: 15d-PGJ2, 15-deoxy-Δ12,14-PGJ2; ALS, amyotrophic lateral sclerosis; ARE, antioxidant response element; ATM, ataxia-telangiectasia mutated; COX, cyclooxygenase; GST, glutathione S-transferases; HPLC, high performance liquid chromatography; HSA, human serum albumin; Keap1, Kelch-like ECH-associating protein 1; IL, interleukin; LPS, lipopolysaccharide; Nrf2, nuclear factor-erythroid 2-related factor 2; PG, prostaglandin; PGDS, prostaglandin D synthase; PPAR, peroxisome proliferator-activated receptor; ROS, reactive oxygen species; Trx, thioredoxin; TrxR, thioredoxin reductase.
This review was written in response to the author’s receipt of the JSBBA Award for Young Scientists in 2014.
This article was originally published with errors. This version has been corrected. Please see Erratum (http://dx.doi.org/10.1080/09168451.2015.1068527).
References
- Smith WL. The eicosanoids and their biochemical mechanisms of action. Biochem. J. 1989;259:315–324.
- Smith WL. Prostanoid biosynthesis and mechanisms of action. Am. J. Physiol. 1992;263:F181–F191.
- Giles H, Leff P. The biology and pharmacology of PGD2. Prostaglandins. 1988;35:277–300.10.1016/0090-6980(88)90093-7
- Urade Y, Eguchi N. Lipocalin-type and hematopoietic prostaglandin D synthases as a novel example of functional convergence. Prostaglandins Other Lipid Mediat. 2002;68–69:375–382.10.1016/S0090-6980(02)00042-4
- Fukushima M. Prostaglandin J2: anti-tumour and anti-viral activities and the mechanisms involved. Eicosanoids. 1990;3:189–199.
- Herschman HR, Reddy ST, Xie W. Function and regulation of prostaglandin synthase-2. Adv. Exp. Med. Biol. 1997;407:61–66.10.1007/978-1-4899-1813-0
- Offenbacher S, Odle BM, Van Dyke TE. The use of crevicular fluid prostaglandin E2 levels as a predictor of periodontal attachment loss. J. Periodontal Res. 1986;21:101–112.10.1111/jre.1986.21.issue-2
- Fitzpatrick FA, Wynalda MA. Albumin-catalyzed metabolism of prostaglandin D2. Identification of products formed in vitro. J. Biol. Chem. 1983;258:11713–11718.
- Kikawa Y, Narumiya S, Fukushima M, Wakatsuka H, Hayaishi O. 9-Deoxy-Δ9, Δ12-13,14-dihydroprostaglandin D2, a metabolite of prostaglandin D2 formed in human plasma. Proc. Natl. Acad. USA. 1984;81:1317–1321.10.1073/pnas.81.5.1317
- Forman BM, Tontonoz P, Chen J, Brun RP, Spiegelman BM, Evans RM. 15-Deoxy-Δ12,14-prostaglandin J2 is a ligand for the adipocyte determination factor PPARγ. Cell. 1995;83:803–812.10.1016/0092-8674(95)90193-0
- Kliewer SA, Lenhard JM, Willson TM, Patel I, Morris DC, Lehmann JM. A prostaglandin J2 metabolite binds peroxisome proliferator-activated receptor γ and promotes adipocyte differentiation. Cell. 1995;83:813–819.10.1016/0092-8674(95)90194-9
- Rossi A, Kapahi P, Natoli G, Takahashi T, Chen Y, Karin M, Santoro MG. Anti-inflammatory cyclopentenone prostaglandins are direct inhibitors of IκB kinase. Nature. 2000;403:103–108.10.1038/47520
- Bui T, Straus DS. Effects of cyclopentenone prostaglandins and related compounds on insulin-like growth factor-I and Waf1 gene expression. Biochim. Biophys. Acta. 1998;1397:31–42.10.1016/S0167-4781(97)00214-5
- Fukushima M. Biological activities and mechanisms of action of PGJ2 and related compounds: an update. Prostaglandins Leukot. Essent. Fatty Acids. 1992;47:1–12.10.1016/0952-3278(92)90178-L
- Shibata T, Kondo M, Osawa T, Shibata N, Kobayashi M, Uchida K. 15-Deoxy-Δ12,14-prostaglandin J2: a prostaglandin D2 metabolite generated during inflammatory processes. J. Biol. Chem. 2002;277:10459–10466.10.1074/jbc.M110314200
- Coste A, Dubourdeau M, Linas MD, Cassaing S, Lepert JC, Balard P, Chalmeton S, Bernad J, Orfila C, Seguela JP, Pipy B. PPARγ promotes mannose receptor gene expression in murine macrophages and contributes to the induction of this receptor by il-13. Immunity. 2003;19:329–339.10.1016/S1074-7613(03)00229-2
- Itoh K, Mochizuki M, Ishii Y, Ishii T, Shibata T, Kawamoto Y, Kelly V, Sekizawa K, Uchida K, Yamamoto M. Transcription factor Nrf2 regulates inflammation by mediating the effect of 15-deoxy-Δ12,14-prostaglandin J2. Mol. Cell. Biol. 2004;24:36–45.10.1128/MCB.24.1.36-45.2004
- Mochizuki M, Ishii Y, Itoh K, Iizuka T, Morishima Y, Kimura T, Kiwamoto T, Matsuno Y, Hegab AE, Nomura A, Sakamoto T, Uchida K, Yamamoto M, Sekizawa K. Role of 15-deoxy-Δ12,14-prostaglandin J2 and Nrf2 pathways in protection against acute lung injury. Am. J. Respir. Crit. Care Med. 2005;171:1260–1266.10.1164/rccm.200406-755OC
- Kondo M, Shibata T, Kumagai T, Osawa T, Shibata N, Kobayashi M, Sasaki S, Iwata M, Noguchi N, Uchida K. 15-Deoxy-Δ12,14-prostaglandin J2: the endogenous electrophile that induces neuronal apoptosis. Proc. Natl. Acad. Sci. USA. 2002;99:7367–7372.10.1073/pnas.112212599
- Sanchez-Gomez FJ, Cernuda-Morollon E, Stamatakis K, Perez-Sala D. Protein thiol modification by 15-deoxy-Δ12,14-prostaglandin J2 addition in mesangial cells: role in the inhibition of pro-inflammatory genes. Mol. Pharmacol. 2004;66:1349–1358.10.1124/mol.104.002824
- Kim HJ, Kim JY, Meng Z, Wang LH, Liu F, Conrads TP, Burke TR, Veenstra TD, Farrar WL. 15-Deoxy-Δ12,14-prostaglandin J2 inhibits transcriptional activity of estrogen receptor-alpha via covalent modification of DNA-binding domain. Cancer Res. 2007;67:2595–2602.10.1158/0008-5472.CAN-06-3043
- Shibata T, Yamada T, Ishii T, Kumazawa S, Nakamura H, Masutani H, Yodoi J, Uchida K. Thioredoxin as a molecular target of cyclopentenone prostaglandins. J. Biol. Chem. 2003;278:26046–26054.10.1074/jbc.M303690200
- Shiraki T, Kamiya N, Shiki S, Kodama TS, Kakizuka A, Jingami H. Unsaturated ketone is a core moiety of natural ligands for covalent binding to peroxisome proliferator-activated receptor γ. J. Biol. Chem. 2005;280:14145–14153.10.1074/jbc.M500901200
- Shiraki T, Kodama TS, Shiki S, Nakagawa T, Jingami H. Spectroscopic analyses of the binding kinetics of 15d-PGJ2 to the PPARγ ligand-binding domain by multi-wavelength global fitting. Biochem. J. 2006;393:749–755.
- Oliva JL, Perez-Sala D, Castrillo A, Martinez N, Canada FJ, Bosca L, Rojas JM. The cyclopentenone 15-deoxy-Δ12,14-prostaglandin J2 binds to and activates H-Ras. Proc. Natl. Acad. Sci. USA. 2003;100:4772–4777.10.1073/pnas.0735842100
- Prestera T, Zhang Y, Spencer SR, Wilczak CA, Talalay P. The electrophile counterattack response: protection against neoplasia and toxicity. Adv. Enzyme Regul. 1993;33:281–296.10.1016/0065-2571(93)90024-8
- Rushmore TH, King RG, Paulson KE, Pickett CB. Regulation of glutathione S-transferase Ya subunit gene expression: identification of a unique xenobiotic-responsive element controlling inducible expression by planar aromatic compounds. Proc. Natl. Acad. Sci. USA. 1990;87:3826–3830.10.1073/pnas.87.10.3826
- Friling RS, Bensimon A, Tichauer Y., Daniel V. Xenobiotic-inducible expression of murine glutathione S-transferase Ya subunit gene is controlled by an electrophile-responsive element. Proc. Natl. Acad. Sci. USA. 1990;87:6258–6262.10.1073/pnas.87.16.6258
- Kawamoto Y, Nakamura Y, Naito Y, Torii Y, Kumagai T, Osawa T, Ohigashi H, Satoh K, Imagawa M, Uchida K. Cyclopentenone prostaglandins as potential inducers of phase II detoxification enzyme: 15-deoxy-Δ12,14-prostaglandin J2-induced expression of glutathione S-transferases. J. Biol. Chem. 2000;275:11291–11299.10.1074/jbc.275.15.11291
- Hosoya T, Maruyama A, Kang M-I, Kawatani Y, Shibata T, Uchida K, Warabi E, Noguchi N, Itoh K, Yamamoto M. Differential responses of the Nrf2-Keap1 system to laminar and oscillatory shear stresses in endothelial cells. J. Biol. Chem. 2005;280:27244–27250.
- Wakabayashi N, Dinkova-Kostova AT, Holtzclaw WD, Kang MI, Kobayashi A, Yamamoto M, Kensler TW, Talalay P. Protection against electrophile and oxidant stress by induction of the phase 2 response: fate of cysteines of the Keap1 sensor modified by inducers. Proc. Natl. Acad. Sci. USA. 2004;101:2040–2045.10.1073/pnas.0307301101
- Kobayashi A, Kang MI, Watai Y, Tong KI, Shibata T, Uchida K, Yamamoto M. Oxidative and electrophilic stresses activate Nrf2 through inhibition of ubiquitination activity of Keap1. Mol. Cell. Biol. 2006;26:221–229.10.1128/MCB.26.1.221-229.2006
- Zhang DD, Hannink M. Distinct cysteine residues in Keap1 are required for Keap1-dependent ubiquitination of Nrf2 and for stabilization of Nrf2 by chemopreventive agents and oxidative stress. Mol. Cell. Biol. 2003;23:8137–8151.10.1128/MCB.23.22.8137-8151.2003
- Eggler AL, Liu G, Pezzuto JM, van Breemen RB, Mesecar AD. Modifying specific cysteines of the electrophile-sensing human Keap1 protein is insufficient to disrupt binding to the Nrf2 domain Neh2. Proc. Natl. Acad. Sci. USA. 2005;102:10070–10075.10.1073/pnas.0502402102
- Kondo M, Oya-Ito T, Kumagai T, Osawa T, Uchida K. Cyclopentenone prostaglandins as potential inducers of intracellular oxidative stress. J. Biol. Chem. 2001;276:12076–12083.10.1074/jbc.M009630200
- Ishii T, Sakurai T, Usami H, Uchida K. Oxidative modification of proteasome: identification of an oxidation-sensitive subunit in 26 S proteasome. Biochemistry. 2005;44:13893–13901.10.1021/bi051336u
- Ishii T, Uchida K. Induction of reversible cysteine-targeted protein oxidation by an endogenous electrophile 15-deoxy-Δ12,14-prostaglandin J2. Chem. Res. Toxicol. 2004;17:1313–1322.10.1021/tx049860+
- Moos PJ, Edes K, Cassidy P, Massuda E, Fitzpatrick FA. Electrophilic prostaglandins and lipid aldehydes repress redox-sensitive transcription factors p53 and hypoxia-inducible factor by impairing the selenoprotein thioredoxin reductase. J. Biol. Chem. 2003;278:745–750.10.1074/jbc.M211134200
- Ciechanover A. The ubiquitin-proteasome proteolytic pathway. Cell. 1994;79:13–21.10.1016/0092-8674(94)90396-4
- Jentsch S, Schlenker S. Selective protein degradation: a journey's end within the proteasome. Cell. 1995;82:881–884.10.1016/0092-8674(95)90021-7
- Shibata T, Yamada T, Kondo M, Tanahashi N, Tanaka K, Nakamura H, Masutani H, Yodoi J, Uchida K. An endogenous electrophile that modulates the regulatory mechanism of protein turnover: inhibitory effects of 15-deoxy-Δ12,14-prostaglandin J2 on proteasome. Biochemistry. 2003;42:13960–13968.10.1021/bi035215a
- Mullally JE, Moos PJ, Edes K, Fitzpatrick FA. Cyclopentenone prostaglandins of the J series inhibit the ubiquitin isopeptidase activity of the proteasome pathway. J. Biol. Chem. 2001;276:30366–30373.10.1074/jbc.M102198200
- Wang Z, Aris VM, Ogburn KD, Soteropoulos P, Figueiredo-Pereira ME. Prostaglandin J2 alters pro-survival and pro-death gene expression patterns and 26 S proteasome assembly in human neuroblastoma cells. J. Biol. Chem. 2006;281:21377–21386.10.1074/jbc.M601201200
- Hortelano S, Castrillo A, Alvarez AM, Bosca L. Contribution of cyclopentenone prostaglandins to the resolution of inflammation through the potentiation of apoptosis in activated macrophages. J. Immunol. 2000;165:6525–6531.10.4049/jimmunol.165.11.6525
- Kobayashi M, Ono H, Mihara K, Tauchi H, Komatsu K, Shibata T, Shimizu H, Uchida K, Yamamoto K. ATM activation by a sulfhydryl-reactive inflammatory cyclopentenone prostaglandin. Genes Cells. 2006;11:779–789.10.1111/gtc.2006.11.issue-7
- Kamagata C, Tsuji N, Moriai M, Kobayashi D, Watanabe N. 15-Deoxy-Δ12,14-prostaglandin J2 inhibits G2-M phase progression in human breast cancer cells via the down-regulation of cyclin B1 and survivin expression. Breast Cancer Res. Treat. 2007;102:263–273.10.1007/s10549-006-9336-3
- Nakata S, Yoshida T, Shiraishi T, Horinaka M, Kouhara J, Wakada M, Sakai T. 15-Deoxy-Δ12,14-prostaglandin J2 induces death receptor 5 expression through mRNA stabilization independently of PPARγ and potentiates TRAIL-induced apoptosis. Mol. Cancer Ther. 2006;5:1827–1835.10.1158/1535-7163.MCT-06-0023
- Stamatakis K, Sanchez-Gomez FJ, Perez-Sala D. Identification of novel protein targets for modification by 15-deoxy-Δ12,14-prostaglandin J2 in mesangial cells reveals multiple interactions with the cytoskeleton. J. Am. Soc. Nephrol. 2006;17:89–98.
- Gayarre J, Sanchez D, Sanchez-Gomez FJ, Terron MC, Llorca O, Perez-Sala D. Addition of electrophilic lipids to actin alters filament structure. Biochem. Biophys. Res. Commun. 2006;349:1387–1393.10.1016/j.bbrc.2006.09.005
- Aldini G, Carini M, Vistoli G, Shibata T, Kusano Y, Gamberoni L, Dalle-Donne I, Milzani A, Uchida K. Identification of actin as a 15-deoxy-Δ12,14-prostaglandin J2 target in neuroblastoma cells: mass spectrometric, computational and functional approaches to investigate the effect of cytoskeletal deagangement. Biochemistry. 2007;46:2707–2718.10.1021/bi0618565
- Han C, Qi J, Shi X, Sakagami Y, Shibata T, Uchida K, Ojika M. Prostaglandins from a zoanthid: paclitaxel-like neurite-degenerating and microtubule-stabilizating activities. Biosci. Biotechnol. Biochem. 2006;70:706–711.10.1271/bbb.70.706