Abstract
In this study, dual-cylindrical anaerobic digesters were designed and built on the pilot plant scale for the improvement of anaerobic digestion efficiency. The removal efficiency of organics, biogas productivity, yield, and microbial communities was evaluated as performance parameters of the digester. During the stable operational period in the continuous mode, the removal efficiencies of chemical oxygen demand and total solids were 74.1 and 65.1%, respectively. Biogas productivities of 63.9 m3/m3-FWW and 1.3 m3/kg-VSremoved were measured. The hydrogenotrophic methanogen orders, Methanomicrobiales and Methanobacteriales, were predominant over the aceticlastic methanogen order, Methanosarcinaceae, probably due to the tolerance of the hydrogenotrophs to environmental perturbation in the field and their faster growth rate compared with that of the aceticlastics.
Graphical Abstract
Innovative dual-cylindrical anaerobic digester
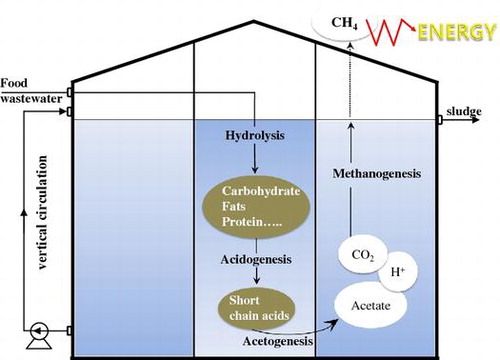
Food wastewater (FWW) is defined as high-strength organic wastewater mixed with food waste or process water from food recycling facilities. It has been reported that large amounts of FWW, containing high organic content of about 9431 m3 per day, were produced in South Korea in 2011, generating increasing concern about its environmental and economic burden.Citation1) For their efficient disposal, land disposal to just over half of FWW and marine treatment of the residue has been coupled. The dumping of food waste into the ocean was prohibited in 2013 in South Korea, promoting considerable interest in the development of alternative sustainable methods based on FWW treatment to replace current dumping practices.
Anaerobic digestion (AD) has been considered as a solution to this problem and is one of the most suitable processes owing to its cost-effectiveness, low sludge generation, and production of renewable biogas, including methane.Citation2) AD involves a series of microbial processes in which microbes of the domains Bacteria and Archaea decompose complex organic matters into methane and carbon dioxide as end products in the absence of oxygen. The complex biochemical AD process is divided into four major steps: hydrolysis, acidogenesis, acetogenesis, and methanogenesis. Complex organic matters are initially decomposed into simpler matters, such as carbohydrates, proteins, and lipids, and are then hydrolyzed into monosaccharides, amino acids, and fatty acids, respectively. These compounds are then further degraded by fermentation, or acidogenesis, into organic acids (e.g. propionates, butyrates). These acids are partially oxidized into hydrogens and acetates through acetogenesis. Methanogenesis is the final step of the process, where acetates and hydrogens are transformed by methanogens into methane and carbon dioxide. While microorganisms within the domain of bacteria are responsible for hydrolysis, acidogenesis, and acetogenesis, Archaea are responsible for methanogenesis.Citation3) Thus, understanding how these microorganisms affect each other by monitoring the microbial population in the digesters through treatment with various substrates would be beneficial to improve the AD performance.
Various anaerobic reactors have been developed as another solution to improve the AD process such as sequential batch, upflow anaerobic sludge blanket, and horizontal digester types. In particular, our newly designed digester is the characteristic of maximizing the methanogenic level and minimizing the dead volume in the reactor for improving AD efficiency. The dead space inside the AD reactor can increase during a general AD process owing to incomplete stirring; hence, an appropriate stirring intensity and effective removal of deposits forming in the lower area of the tank are mandatory to minimize the dead space.Citation4,5) The reactor used in this study was designed to meet these requirements described above.
Therefore, the aim of this study was to investigate the microbial community dynamics in relation to the process performance in a novel AD reactor system and examine the bioconversion of FFW into methane. For this purpose, a pilot-scale reactor was operated and the performance was regularly monitored. Culture-independent quantitative polymerase chain reaction (qPCR) was applied for the methanogenic quantification to allow for the interpretation of the field performance of AD.
Materials and methods
FWW
FWW for AD was collected from dry feed manufacturing plants at Incheon in South Korea. The FWW was screened, homogenized, and stored in a storage tank for subsequent use as a substrate. The FWW used here was acidic wastewater with high organic content; the characteristics are shown in Table . The average value of chemical oxygen demand (COD) of raw FWW was measured at 115.9 ± 8.0 g/L, and total solids (TS) was 83.7 ± 5.8 g/L. Total volatile fatty acid and long-chain fatty acid (LCFA) values were determined at 26.7 ± 3.3 and 8.5 ± 0.9 g/L, respectively.
Table 1. Characteristics of food wastewater and removal efficiency of pilot-scale AD.
Pilot plant and dual-cylindrical anaerobic digester
Pilot plants consist of pretreatment facilities, a dual-cylindrical anaerobic digester (600 m3 working volume), a gas holder, and odor control equipment. The digester was divided into two compartments with different capacities. The working volumes of the inner and outer reactors were 150 and 450 m3, respectively (Fig. (a)). The wastewater flows into the top of the inner reactor (1st reactor) and then flows out from the outer reactor (2nd reactor) (Fig. (b)). The inner reactor plays a role in the acidogenic phase, pH buffering, and relieving organic loading shock from the input substrate. The outer reactor was designed as the main reactor for the methanogenic process. The stirring system consists of three impellers (inner reactor), two mixers (outer reactor), and a pump for sludge circulation (Fig. (c)). The circulation water was heated with energy produced by the LNG boiler or the biogas generator, so that this heated water could be employed to maintain the temperature of the digesters at around 36–38 °C for mesophilic digestion.
DNA extraction and real-time PCR analysis
Total DNA was extracted from the anaerobic sludge using an automated nucleic acid extractor (Magtration System 6GC, PSS, Chiba, Japan). The extracted DNA was eluted with 100 μL of 10 mM Tris-HCl buffer (pH 8.0) and stored at −20 °C for quantitative real-time PCR (qPCR).
The methanogen concentrations in the anaerobic sludge were measured using the LightCycler 480 PCR system (Roche Diagnostics, Mannheim, Germany) with the gene-specific primer-probe sets developed previously (Table ) Citation6) for the specific detection of the domain Bacteria (BAC), the domain Archaea (ARC), the order Methanobacteriales (MBT), the order Methanomicrobiales (MMB), the order Methanosarcinaceae (MSL), and the order Methanococcales (MCC). All qPCR runs were performed in duplicates, and each reaction mixture was prepared using the LightCycler 480 Probes Master kit (Roche Diagnostics) in a total volume of 20:10 μL of 2× reaction solutions, 1 μL of each primer (final concentration, 500 nM), 1 μL of TaqMan probe (final concentration, 100 nM), 2 μL of template DNA, and 5 μL of PCR-grade water. The thermal cycling protocol was as follows: initial denaturation for 10 min at 94 °C followed by 45 cycles of 10 s at 94 °C and combined annealing and extension for 30 s at 60 °C (63 °C for the MMB set).Citation7) The standard curves used for real-time PCR analysis have been described previously.Citation8)
Table 2. Primer and probes sets used in this study.Citation6)
Analytic methods
The COD concentration and solids were determined according to the procedures described in standard methods.Citation9) Analysis for volatile fatty acids (VFAs), including formic acid, lactic acid, acetic acid, and propionic acid, was performed using a liquid chromatograph (Agilent HPLC 1100; Agilent, Palo Alto, CA, USA) equipped with an Agilent ZORBAX CB-Aq column.
Results and discussion
Start-up of the pilot plant
After seeding with the leachate anaerobic sludge in the Sudokwon landfill site, the anaerobic digesters were operated in the batch mode for process stabilization for 2 weeks. After that, feeding was started at a velocity of 0.4 m3/h, and the feed load was increased by 0.1 m3/h per week. After stabilization and gradual increase of organic loading, FWW was ultimately loaded to the digester at a velocity of 24 m3/d. FWW and anaerobic sludge samples were collected fifteen times at an interval of 6 days during the 3-month operation period for subsequent performance analysis of the anaerobic process.
The average pH value of FWW was about 3.5, and it contained a relatively high concentration of VFAs (Table ). When the substrate input with an acidic pH increases, a pH shock can potentially occur in the AD system. Both sludge samples of the 1st and 2nd reactors were taken from each height level (2 and 8 m). In the 1st reactor, the pH values of the sludge at 8 m and at 2 m were 5.8 and 6.7, respectively, implying that the pH effect of the substrate inlet sequentially decreased from the upper to the lower zone of the digesters. At the same time, the pH values of the lower and upper zone of the 2nd reactor were 7.2 and 7.8, respectively. Thus, the pH range of the 2nd reactor was more suitable for methanogen activity.Citation10) These results indicate that the acidic wastewater had no impacts at least on the 2nd methanogenic digester.
Performance of the dual-cylindrical anaerobic digester
Table shows the concentration of residual organic content and removal efficiencies of various organics in the pilot AD plant. The range of COD removals efficiency was between 61.5 and 81.8% (mean value: 74.1%). These results are comparable to the COD removal efficiency of 74.9% reported in a previous study,Citation7) where food waste was anaerobically digested. TS and VFA removal efficiencies were 65.1 and 99.6%, respectively. The VS/TS ratio of the anaerobic effluent was calculated as 54.2%, which was lower than the ratio of influent (81.0%), indicating that the inorganic matter was left relatively undegraded under anaerobic conditions. The concentration of residual VFAs was 0.1 g/L corresponding to less than 1% of input concentration, which demonstrates their successful conversion to methane in the system. The successful conversion of VFAs to methane was supported by a previous study referring to the fact that VFAs were converted into acetate or hydrogen by acetogenic or hydrogenic bacteria, and methane was then produced by biological activity of methanogens using the hydrogen or the acetate.Citation11) If VFAs had accumulated in the digester, the anaerobic process would have failed, because VFAs are precursors of methane, and methane accumulation is therefore closely related to the pH drop.Citation12,13) Table shows the profile of biogas production and its composition at the steady state level of anaerobic performance. Biogas and methane yield based on VS are shown in Table in comparison with previous studies. In order to objectively determine the process performance, the biogas yield was calculated instead of daily biogas productivity because the latter parameter tends to be variable depending on the characteristics of input substrate or organic loading rate. Compared to the previous measurements, the biogas productivity and its yield were superior to those in the laboratory scale.Citation14) Thus, the newly designed pilot plant in this study is a potential promising option for biogas production in the field.
Table 3. Profile of biogas production at steady state in pilot-scale anaerobic digesters.
Table 4. Comparative study of biogas and methane yield, based on removed VS.
Microbial population in AD
Fig. shows the copy number of the 16S rRNA gene in the anaerobic digester using group-specific primer-probe sets for methanogens. Three methanogenic data points represent a time-dependent change of microbial flora, obtained every month throughout the entire 3-month operation period. There was no big difference in each fraction of microbial community structure in Fig. in spite of slight fluctuation of gas productivity during the steady state phase, in view of the standard deviation of gas productivity shown in Table . The summarized 16S rRNA gene copy numbers for the orders [i.e. MBT, MMB, MSL] covered 81.1 ± 9.9% of all methanogens, indicating that the summarization of the three methanogens is valid, given that the nesting value was similar to the one in a previous report.Citation8) The order MCC was undetectable in this study, but because this order comprises halophilic methanogens, its detection in an anaerobic digester is not expected.Citation15)
Fig. 2. Population of the methanogen 16S rRNA gene in AD (each trial number reflects the order of sampling performed at an interval of a month during 3-month operation period).
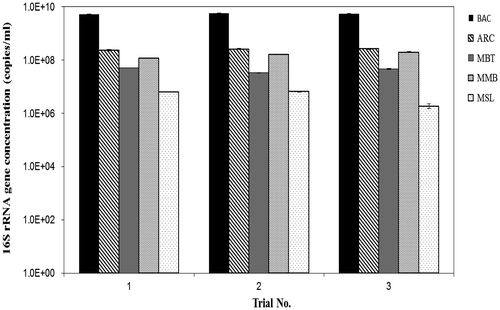
The 16S rRNA gene copy numbers of the domain Bacteria and Archaea were 5.5 × 109 and 2.6 × 108 copies/mL, respectively. The ratio of ARC and BAC was 4.6 ± 0.2%, which is similar to that reported from other anaerobic processes treating organic waste.Citation16,17) The copy numbers for MBT, MMB, and MSL were found to be 4.4 × 107, 1.6 × 108, 4.9 × 106 copies/mL, respectively. As a result of numerical conversion of these results to methanogenic fraction numbers, the factions of MMB, MBT, and MSL were calculated to cover 62, 17, and 2.0% of all methanogens, respectively, showing that MMB was predominant in this system. The hydrogenotrophic orders, MMB and MBT, were previously reported as the most dominant methanogenic group in an AD process treating swine wastewater.Citation18) However, the minor methanogens, namely aceticlastic methanogens such as MSL, are highly, sensitive to environmental change such as perturbations of the O2 concentration or LCFAs,Citation19,20) which support the dominance of hydrogenotrophic methanogens under severe environmental perturbations.Citation21,22) In addition, the maximum specific growth rate (μm) of aceticlastic methanogens is generally believed to be lower than that of hydrogenotrophic methanogens.Citation23) In another study, aceticlastic methanogens have been reported as the dominant microorganisms in an AD process fed by food wastewater; however, this process was performed on the laboratory scale without significant environmental perturbation.Citation24) Furthermore, hydrogenotrophs were predominant in all AD processes fed by whey permeate, sewage sludge, or swine wastewater.Citation16,25–29) Taking all these observations into consideration, the methanogenic dominance seems to be affected by the digesters scale in relation to the control of the operation conditions. Given the qPCR results for the methanogenic population dynamics, methane production seems to be achieved mainly by the use of hydrogen and carbon dioxide, coupled with acetate conversion into H2 and CO2 in this pilot plant.Citation27)
In conclusion, a novel dual-cylindrical anaerobic digester was as successfully designed for minimizing the dead volume and maximizing the stirring effect, and was operated in the batch and continuous modes. As a result, biogas productivity and yield were better than those in previous laboratory-scale studies.Citation7,30) In the methanogenic quantification, MMB were found to be dominant in field-scale pilot plants, showing that hydrogenotrophic methanogens would be more tolerant to the perturbation of environmental parameters than aceticlastic methanogens. Further studies need to determine the effect of acetate conversion to hydrogen and carbon dioxide on the AD efficiency in these plants.
Funding
This work was supported by the New & Renewable Energy of the Korea Institute of Energy Technology Evaluation and Planning (KETEP) grant funded by the Korea Government Ministry of Trade, Industry and Energy [grant number 20113010090010].
References
- Kim M, Song M, Jo M, Shin S, Khim J, Hwang S. Growth condition and bacterial community for maximum hydrolysis of suspended organic materials in anaerobic digestion of food waste-recycling wastewater. Appl. Microbiol. Biotechnol. 2010;85:1611–1618.10.1007/s00253-009-2316-x
- Kim W, Shin S, Lim J, Hwang S. Effect of temperature and hydraulic retention time on volatile fatty acid production based on bacterial community structure in anaerobic acidogenesis using swine wastewater. Bioproc. Biosys. Eng. 2013;36:791–798.10.1007/s00449-013-0905-7
- Lee SH, Kang HJ, Lee YH, Lee TJ, Han K, Choi Y, Park HD. Monitoring bacterial community structure and variability in time scale in full-scale anaerobic digesters. J. Environ. Monitor. 2012;14:1893–1905.10.1039/c2em10958a
- Stafford DA. The effects of mixing and volatile fatty acid concentrations on anaerobic digester performance. Biomass. 1982;2:43–55.10.1016/0144-4565(82)90006-3
- Terashima M, Goel R, Komatsu K, Yasui H, Takahashi H, Li YY, Noike T. CFD simulation of mixing in anaerobic digesters. Bioresour. Technol. 2009;100:2228–2233.10.1016/j.biortech.2008.07.069
- Yu Y, Lee C, Kim J, Hwang S. Group-specific primer and probe sets to detect methanogenic communities using quantitative real-time polymerase chain reaction. Biotechnol Bioeng. 2005;89:670–679.10.1002/(ISSN)1097-0290
- Shin SG, Zhou BW, Lee S, Kim W, Hwang S. Variations in methanogenic population structure under overloading of pre-acidified high-strength organic wastewaters. Proc. Biochem. 2011;46:1035–1038.10.1016/j.procbio.2011.01.009
- Song M, Shin SG, Hwang S. Methanogenic population dynamics assessed by real-time quantitative PCR in sludge granule in upflow anaerobic sludge blanket treating swine wastewater. Bioresour. Technol. 2010;101:S23–S28.10.1016/j.biortech.2009.03.054
- APHA-AWWA-WEF, Standard methods for the examination of water and wastewater. Washington (DC): American Public Health Association; 2005.
- Lay JJ, Li YY, Noike T. Influences of pH and moisture content on the methane production in high-solids sludge digestion. Water Res. 1997;31:1518–1524.10.1016/S0043-1354(96)00413-7
- Ferry JG. Methane from acetate. J. Bacteriol. 1992;174:5489–5495.
- Ahring BK, Sandberg M, Angelidaki I. Volatile fatty acids as indicators of process imbalance in anaerobic digestors. Appl. Microbiol. Biotechnol. 1995;43:559–565.10.1007/BF00218466
- Murto M, Björnsson L, Mattiasson B. Impact of food industrial waste on anaerobic co-digestion of sewage sludge and pig manure. J. Environ. Manage. 2004;70:101–107.10.1016/j.jenvman.2003.11.001
- Kim W, Shin SG, Cho K, Lee C, Hwang S. Performance of methanogenic reactors in temperature phased two-stage anaerobic digestion of swine wastewater. J. Biosci. Bioeng. 2012;114:635–639.10.1016/j.jbiosc.2012.07.012
- Boone DR, Castenholz RW. Bergey’s Manual® of systematic bacteriology. New York: Springer; 2001.10.1007/978-0-387-21609-6
- Moset V, Bertolini E, Cerisuelo A, Cambra M, Olmos A, Cambra-López M. Start-up strategies for thermophilic anaerobic digestion of pig manure. Energy. 2014;74:389–395.10.1016/j.energy.2014.07.003
- Shin SG, Lee S, Lee C, Hwang K, Hwang S. Qualitative and quantitative assessment of microbial community in batch anaerobic digestion of secondary sludge. Bioresour Technol. 2010;101:9461–9470.10.1016/j.biortech.2010.07.081
- Kim W, Lee S, Shin SG, Lee C, Hwang K, Hwang S. Methanogenic community shift in anaerobic batch digesters treating swine wastewater. Water Res. 2010;44:4900–4907.10.1016/j.watres.2010.07.029
- Hanaki K, Matsuo T, Nagase M. Mechanism of inhibition caused by long-chain fatty acids in anaerobic digestion process. Biotechnol. Bioeng. 1981;23:1591–1610.10.1002/(ISSN)1097-0290
- Tang Y, Shigematsu T. The effects of micro-aeration on the phylogenetic diversity of microorganisms in a thermophilic anaerobic municipal solid-waste digester. Water Res. 2004;38:2537–2550.10.1016/j.watres.2004.03.012
- Jaenicke S, Ander C, Bekel T, Bisdorf R, Dröge M, Gartemann KH, Jünemann S, Kaiser O, Krause L, Tille F, Zakrzewski M, Pühler A, Schlüter A, Goesmann A. Comparative and joint analysis of two metagenomic datasets from a biogas fermenter obtained by 454-pyrosequencing. PLoS ONE. 2011;6:e14519.10.1371/journal.pone.0014519
- O’Reilly J, Lee C, Chinalia F, Collins G, Mahony T, O’Flaherty V. Microbial community dynamics associated with biomass granulation in low-temperature (15°C) anaerobic wastewater treatment bioreactors. Bioresour. Technol. 2010;101:6336–6344.10.1016/j.biortech.2010.03.049
- Ahring BK. Biomethanation I. New York: Springer; 2003.
- Shin SG, Han G, Lim J, Lee C, Hwang S. A comprehensive microbial insight into two-stage anaerobic digestion of food waste-recycling wastewater. Water Res. 2010;44:4838–4849.10.1016/j.watres.2010.07.019
- Bialek K, Kim J, Lee C, Collins G, Mahony T, O’Flaherty V. Quantitative and qualitative analyses of methanogenic community development in high-rate anaerobic bioreactors. Water Res. 2011;45:1298–1308.10.1016/j.watres.2010.10.010
- Hagen LH, Vivekanand V, Linjordet R, Pope PB, Eijsink VGH, Horn SJ. Microbial community structure and dynamics during co-digestion of whey permeate and cow manure in continuous stirred tank reactor systems. Bioresour. Technol. 2014;171:350–359.10.1016/j.biortech.2014.08.095
- Kim J, Kim W, Lee C. Absolute dominance of hydrogenotrophic methanogens in full-scale anaerobic sewage sludge digesters. J. Environ. Sci. 2013;25:2272–2280.10.1016/S1001-0742(12)60299-X
- Kim J, Lee S, Lee C. Comparative study of changes in reaction profile and microbial community structure in two anaerobic repeated-batch reactors started up with different seed sludges. Bioresour. Technol. 2013;129:495–505.10.1016/j.biortech.2012.11.119
- Yu H, Wang Q, Wang Z, Sahinkaya E, Li Y, Ma J, Wu Z. Start-Up of an anaerobic dynamic membrane digester for waste activated sludge digestion: temporal variations in microbial communities. PLoS ONE 2014;9:e93710.10.1371/journal.pone.0093710
- Kim W, Ryu BG, Kim S, Heo SW, Kim D, Kim J, Jo H, Kwon JH, Yang J-W. Quantitative analysis of microbial community structure in two-phase anaerobic digesters treating food wastewater. Korean J. Chem. Eng. 2014;31:381–385.10.1007/s11814-014-0019-1
- Wang JY, Zhang H, Stabnikova O, Tay JH. Comparison of lab-scale and pilot-scale hybrid anaerobic solid-liquid systems operated in batch and semi-continuous modes. Proc. Biochem. 2005;40:3580–3586.10.1016/j.procbio.2005.03.047
- Cho JK, Park SC, Chang HN. Biochemical methane potential and solid state anaerobic digestion of Korean food wastes. Bioresour. Technol. 1995;52:245–253.10.1016/0960-8524(95)00031-9
- Wang C, Zuo J, Chen X, Xing W, Xing L, Li P, Lu X, Li C. Microbial community structures in an integrated two-phase anaerobic bioreactor fed by fruit vegetable wastes and wheat straw. J. Environ. Sci. 2014;26:2484–2492.10.1016/j.jes.2014.06.035
- Jang HM, Kim JH, Ha JH, Park JM. Bacterial and methanogenic archaeal communities during the single-stage anaerobic digestion of high-strength food wastewater. Bioresour. Technol. 2014;165:174–182.10.1016/j.biortech.2014.02.028
- Ganesh R, Torrijos M, Sousbie P, Lugardon A, Steyer JP, Delgenes JP. Single-phase and two-phase anaerobic digestion of fruit and vegetable waste: comparison of start-up, reactor stability and process performance. Waste Manage. 2014;34:875–885.10.1016/j.wasman.2014.02.023