Abstract
Biofilms are sessile microbial aggregates on the interfaces, and they were usually considered as microbial contamination sources in medical care and various industries. We studied the control and application of biofilms formed by food-related microorganisms, and mechanism of the biofilm formation was also investigated. We studied the biofilm formation in mixed cultures using various combinations of two strains of food-related microorganisms. There were various microorganisms that showed decreased or increased biofilm formation in the mixed culture in comparison with that in a single culture. Biofilm formed by lactic acid bacteria and yeast isolated from traditional fermented food, Fukuyama pot vinegar, exhibited unique feature in that structure and formation mechanism, and expected to be used as an immobilized microorganism in fermentation production. Here our studies on the control and application of biofilms and the mechanisms of its formation were described.
Graphical Abstract
We studied the control and application of biofilms formed by food-related microorganisms, and mechanism of the biofilm formation was also investigated.
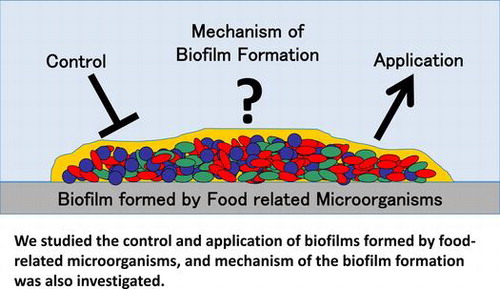
Key words:
Biofilms are attached microbial colonies on the interfacesCitation1–5) (Fig. ). They are usually recognized as contamination source of microorganisms in various industrial fields.Citation1–5) Especially higher drug resistance of biofilms was the serious problem in medical field.Citation1–5) On the other hand, almost all microorganisms would potentially have an ability to form biofilm, and it was considered that there would be some biofilms that contributed to various industries. In fact, biofilms have contributed to biological water clarification.Citation5)
Fig. 1. Schematic model of mixed-species biofilm.Citation14–30)
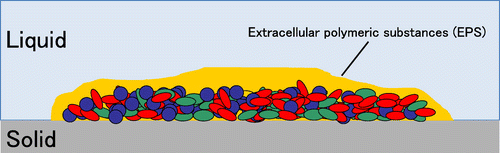
In nature, microorganisms usually exist with other microorganisms and usually they interact with each other. We have firstly studied the mixed-species biofilm formation using 38 food-related microorganisms.Citation6–8) Those approaches told us that formation of biofilms is usually increased or decreased in mixed culture compared to their single culture. This study was the start of our study on the biofilms; we tried to search the microorganisms that could inhibit the biofilm formation of hazardous bacteria and those that could increase the biofilm formation of useful bacteria.
We have studied the control method or application in fermentation production of biofilms formed by food-related microorganisms. Following three studies were conducted.
(1) | Development of control method of biofilms formed by food spoilage or poisoning microorganisms. | ||||
(2) | Application of biofilms formed by fermentation-related microorganisms in fermentation production. | ||||
(3) | Elucidation of biofilm formation mechanism in model microorganisms. |
Control of the biofilms is a very important issue in the food industry and also in the medical fields. We tried to decrease the biofilm formation of food-related microorganisms by food additives and food stuffs such as spices for finding the biofilm controlling factors usable in food industry.Citation7–10) We also tried to control biofilms by non-thermal sterilization treatment.Citation7)
Application of biofilms as immobilized cells is also important and challenging approach in food industry. Here, we isolated yeast, lactic acid bacteria (LAB) and acetic acid bacteria (AAB) from Fukuyama pot vinegar,Citation11) traditional rice vinegar produced in Japan.Citation11–13) Among these brewing-related microorganisms, we found one unique LAB strain that could form thick mixed-species biofilm in combination culture with budding yeasts.Citation14) The mechanism of the mixed-species biofilm formation, and the role and significance of that mixed-species biofilm were also considered and investigated.Citation16–26) Application of the mixed-species biofilm as immobilized cells was also investigated.Citation27–30) Artificial immobilization of cells usually needs high costs for manufacturing; on the other hand, biofilms are automatically formed by culturing microorganisms and their immobilized cells are renewed automatically under appropriate culturing conditions.
Elucidation of biofilm formation mechanism using model microorganisms was also important approach for understanding the properties and mechanism of biofilm formation by microorganisms. In this approach, Escherichia coli K-12 strain was used. Postgenomic analysis of the planktonic and biofilm cells of E. coli K-12 was conducted, and transposon (Tn) mutant library was also used for isolating biofilm mutants.Citation31,32) We could find various biofilm-related genes and also could get some basic information of bacterial biofilm formation.Citation33–35)
Biofilm formation assay was performed according to the method described previously.Citation33) Overnight, cultures were diluted 1:100 into fresh medium and grown in 96-well microtiter plates at 30 °C for various cultivation periods. Biofilm formation was estimated by measuring the crystal violet staining of the cells attached to the microtiter plate wells.Citation2,3,33)
In this review, we described the general outline of above our studies, and we would like to discuss the prospects of the control and application of biofilms formed by food-related microorganisms.
I. Development of control methods of biofilms formed by food spoilage or poisoning microorganisms
We investigated the biofilm formation of food-related 38 microorganisms including food-poisoning and also food- spoilage bacteria such as LAB and yeast.Citation6–8) It was showed that microorganisms tend to form higher biofilm in mixed culture more than in single culture, indicating that interspecies interaction would contribute to increasing the co-cultured biofilm formation.
On the other hand, biofilm formation of some microorganisms decreased in co-culture (Fig. ). In particular, biofilm formation of major food-poisoning bacteria, Staphylococcus aureus, extremely decreased in the co-culture with bacteria included in Enterobacteriaceae such as Yersinia intermedia (Fig. ). Biofilm formation of S. aureus was inhibited by the conditioned medium (CM) of Y. intermedia. The CM of Y. intermedia was considered to contain the inhibitor for the biofilm formation of S. aureus. However, we have given up the further investigation on the inhibitor, because biofilm inhibitors of Enterobacteriaceae origin were difficult to apply in food industry from the viewpoint of food hygiene.
We also investigated the biofilm formation of oral bacteria in their mixed culture.Citation6) Streptococcus mutans and S. sobrinus are known as caries inducible bacteria.Citation6) It was indicated that S. salivarius inhibited the biofilm formation of S. mutans and S. sobrinus, and it was showed that fructanase produced by S. salivarius inhibited the biofilm formation of S. mutans and S. sobrinusCitation6) (Fig. ).
Next, we tried to screen the biofilm inhibitor from the food additives usually used in food industry. We tested various food additives such as emulsifiers, preservatives, and gelling agentsCitation7–10) and found that sugar fatty acid esters showed high activities in inhibiting the biofilm formation of various microorganisms, such as S. aureus, E. coli, S. mutans, L. monocytogenes and P. aeruginosaCitation7–10) (Fig. ). Other emulsifiers, surfactants, also showed inhibitory activities, and it was deduced that surfactants tend to have a biofilm inhibitory activities.
In general, usage of food additives controlled under legal regulation, and therefore the application of food additives for biofilm control, is difficult without improvement of the legislation. Then, we again tried to screen the biofilm inhibitor from the food stuffs, especially spices. We tested hot water extracts of about 60 spices available in Japan and found that there were many spices that could inhibit the biofilm formation of S. aureus, E. coli, S. mutans, and L. monocytogenesCitation7–10) (Fig. ). Such extracts could be easily applied in food industry as biofilm inhibitors.
We also tried to control biofilm by non-thermal treatment such as high hydrostatic pressure and high-pressure carbon dioxide treatment. High hydrostatic pressure and high-pressure carbon dioxide are promising non-thermal disinfecting treatment, and we investigate the effect of above treatments on the inactivation of bacterial cells and spores.Citation36–44) We also studied the mechanism of the inactivation of above treatment.Citation36–44) There were some effective non-thermal treatments such as high-pressure carbon dioxide treatment to inactivate the biofilm,Citation7) and there were also some other potential treatment such as pulsed xenon flash light treatment and gaseous chlorine dioxide treatment in inactivation of microorganisms attached on surface of food materials including vegetables.Citation45–47)
II. Application of biofilms formed by fermentation-related microorganisms
We found that there were some combinations showing of increase of biofilm formation in co-culture of yeast and LAB as described in previous study.Citation20) Then, the biofilm formation in co-culture of yeast and LAB was investigated, and it was showed that there were several combinations of increase in the biofilm formation in the co-culture such as Lactobacillus casei and Saccharomyces cerevisiae.Citation48) In that combination, CM of LAB induced the biofilm formation of yeast in its single culture.Citation48)
Usually, LAB and yeast co-exist in various traditional fermentations of all over the world because both favor non-oxygenic environment and also request saccharide such as glucose for their growth.Citation20–26,49–51) We tried to isolate LAB and yeast from Fukuyama pot vinegar that could form higher biofilm in co-culture, and we could isolate the higher mixed-species biofilm-forming combination, Lactobacillus plantarum ML11-11 and S. cerevisiae Y11-43.Citation14) (Fig. ). Fukuyama pot vinegar is one of the most oldest type of rice vinegar in East Asia including Japan, and it has been produced without any artificial manipulation during fermentationCitation11–13) (Fig. ).
Fig. 3. Fermentation process of Fukuyama pot vinegar (A), picture of landscape of fermentation (B), and SEM picture of mixed-species biofilm (C).Citation20–30)
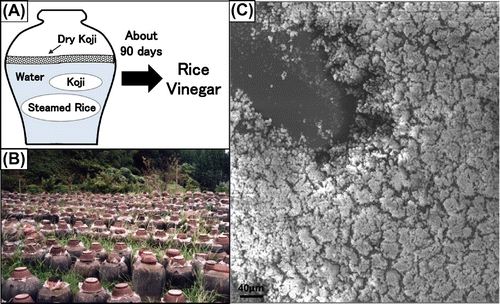
Mixed-species biofilm was also formed by L. plantarum ML11-11 and other S. cerevisiae strains including laboratory strains such as BY4741 or X2180.Citation14–16) Hereafter, biofilm assay was performed using laboratory S. cerevisiae strains.
It was indicated that L. plantarum ML11-11 and S. cerevisiae cells co-aggregated well and formed visible co-aggregates in few minutes, and co-aggregation was necessary for mixed-species biofilm formation.Citation14–16) Mixed-species biofilm was observed by SEM and FISH, and it was indicated that bottom of biofilm was LAB.Citation14–16) Therefore, LAB cells firstly attached abiotic surface, LAB cells co-aggregate with yeast secondly, co-aggregates sediment on the attached LAB cells thirdly, then LAB and yeast cells grew in the biofilm and matured mixed-species biofilm was completedCitation14–16) (Fig. ).
Fig. 4. LAB and yeast mixed-species biofilm formation process.Citation14–30)
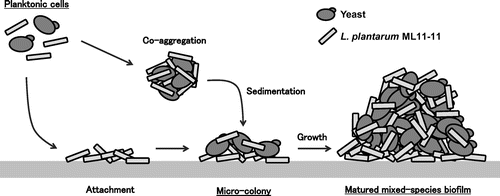
Interaction between unidentified lectin-like protein on L. plantarum ML11-11 cell and mannan on S. cerevisiae cell was necessary for that co-aggregation.Citation14–16) Non-co-aggregative mutans were isolated by culturing the non-co-aggregative cells repeatedly,Citation14–16) and mannan structure necessary for the co-aggregation was clarified.Citation17) Now, we are identifying the aggregation factor of L. plantarum ML11-11 using non-co-aggregative mutants.
Almost all S. cerevisiae strains tested could form mixed-biofilm and co-aggregates with L. plantarum ML11-11; on the other hand, few LAB strains showed the ability of mixed-species biofilm formation, all of them were L. plantarum and Leuconostoc mesenteroides.Citation19,30) L. plantarum strains could co-aggregate with S. cerevisiae like ML11-11; however, one L. mesenteroides strain could not co-aggregate with S. cerevisiae cells.Citation19) In addition, L. plantarum ML11-11 formed more thicker mixed-species biofilm with non-foaming mutant strains of S. cerevisiae sake yeasts than with its parent, foaming, strain, and L. plantarum ML11-11 also showed higher co-aggregation with non-foaming mutant sake yeasts more than with its parent strain.Citation52) It was clarified that Awa1p present on the surface of sake yeast strain inhibits adhesion between yeast cells and L. plantarum ML11-11.Citation52)
Biofilms are one of the immobilized cells, and biofilms on various interfaces could be in various fermentations (Fig. ). Biofilms are formed various microorganisms autonomously and their cells are renewed automatically. We tried to produce ethanol using above mixed-species biofilm of LAB, L. plantarum ML11-11, and yeast, S. cerevisiae.Citation27–30) That mixed-species biofilm are tolerant to physical stress and could produce ethanol well.Citation27–30) Especially, low pH brought about by lactic acid significantly contributed to decreasing the contamination of the biofilm fermentation system.Citation27–30) This biofilm fermentation system would be applied to biomass fermentation process.
Fig. 5. Immobilized cell fermentation on various interfaces; liquid–solid (A), air–solid (B), liquid–liquid (C), and air–liquid (D).Citation20–30)
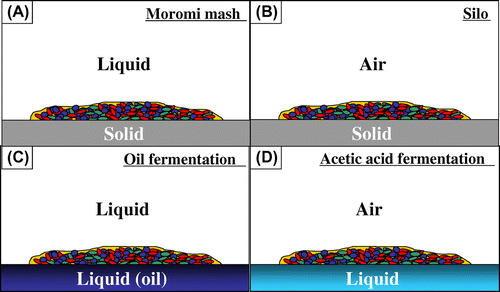
Next biofilm formation in the mixed culture of AAB and LAB isolated from Fukuyama pot vinegar was investigated. Almost all AAB, Acetobacter pasteurianus, formed biofilm, pellicle, on air–liquid interface well in mixed culture with LAB.Citation21,24,26,28) Lactic acid also increased the biofilm formation of AAB, A. pasteurianus A11-10Citation21,24,26,28) (Fig. ). For producing pyruvic acid in A. pasteurianu, glucose should go through the pentose-phosphate pathway, but lactic acid was simply transferred to pyruvic acidCitation21,24,26,28) (Fig. ). A. pasteurianus requests oxygen for acetic acid production, and therefore, the abundant pellicle formation on air–liquid interface is advantageous for efficient acetic acid production. In addition, A. pasteurianus requires ethanol for producing acetic acid. It was deduced that co-existence of lactic acid formed by LAB and ethanol formed by yeast is better for the acetic acid production of A. pasteurianus.Citation21,24,26,28) Thus, mixed-species biofilm of LAB and yeast formed on the bottom of fermentation pot considered to be important for the acetic acid fermentation in Fukuyama pot vinegar fermentationCitation21,24,26,28) (Fig. ). We want to apply this biofilm fermentation system to the biomass processing in the future for its robustness.
Fig. 6. Schematic model of fermentation process of Fukuyama pot vinegar, and lactate and ethanol metabolism of AAB.Citation20–30)
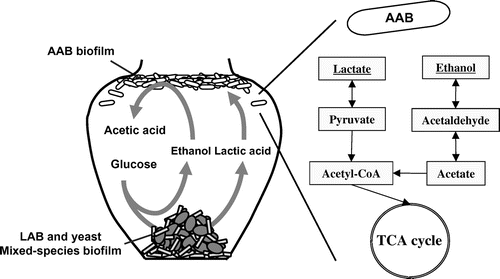
III. Elucidation of biofilm formation mechanism in model microorganisms
Biofilm formation of Escherichia coli K-12 and its mechanism was also investigated. Transcriptome and proteome of biofilm cells of E. coli K-12 were compared with those of planktonic cells, and non- or hyper-biofilm mutant strains were screened from Tn mutant libraryCitation31,32) (Fig. ). We found various biofilm-related genes from above approaches; there were various genes related to cell surface structures such as fimbriae, flagella, and LPS, and there were also genes related to primary metabolite production such as amino acid and nucleic acid. It was already known that fimbriae, flagella, and LPS were necessary for the bacterial biofilm formation.Citation3,53,54)
Fig. 7. Studies on the clarifying the mechanism of biofilm formation of E. coli K-12.Citation31)
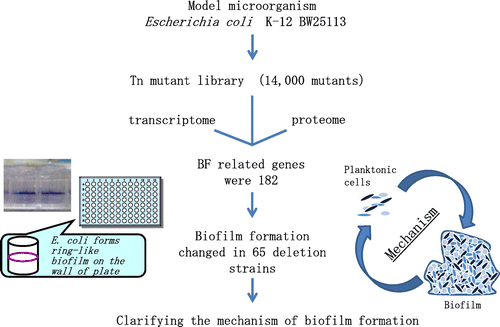
Decrease of intracellular concentration of L-tryptophan would stimulate the biofilm formation of L-tryptophan biosynthesis-deficient mutants of E. coli K-12, and addition of L-tryptophan decreased the biofilm formation of E. coli K-12.Citation32) On the other hand, increase of intracellular concentration of pyrimidine nucleotides would stimulate the biofilm formation of mutants with de-regulated pyrimidine nucleotide biosynthesis, and the addition of L-tryptophan also decreased the biofilm formation of these de-regulated mutants. Now, it was deduced that balance of amino acid and nucleic acid metabolism would be important for the cell lifestyle including biofilm formation, and especially, loss of the balance of amino acid and nucleic acid metabolism would induce the biofilm formation.Citation32,55)
The main objective of this approach using E. coli K-12 mutants was to clarify the mechanism of bacterial biofilm formation, and some important information was acquired from above approach. We want to apply the information acquired from above basic studies to the control and application of biofilm of food-related microorganisms in the future.
IV. Concluding remarks
Here, general outline of our studies on the control and application of food-related microbial biofilm is described.
In food industry, residue of disinfectant is unacceptable, and biofilm is usually highly resistant to chemical and physical treatment. Therefore, we should search the safe biofilm inhibitor that can be used in the food industry. This time, we could find that there were various spices that could inhibit various bacterial biofilms, and now, we are identifying the active components of them. We want to identify safe active components usable in food industry.
Next, we found LAB and yeast mixed-species thick biofilm, and also we indicated that biofilm could be used as immobilized cells in various fermentation processes because of their robustness in higher resistance to mechanical stress and contamination. Now, we want to apply this biofilm fermentation system to biomass fermentation process. In addition, we want to clarify the contribution of biofilms in the traditional fermentation. As described above, biofilms are usually resistant to various environmental stresses, and therefore, biofilm-forming cells could survive in the fermentation process, and then, they could be dominant strains.
Mechanism of the biofilm formation is also an important approach. Biofilm is a specific microbial lifestyle different from their planktonic lifestyle. Change of the lifestyle would be based on change of their gene expression and following change of their metabolism. These metabolic changes occurred with life style change in biofilm forming cells would affect local cells in single culture as well as in mixed culture. Therefore, study on the mechanism of biofilm formation would contribute to the understanding of the relationships between microbial global regulation of gene transcription and metabolism and their lifestyle in single and also in mixed culture.
Understanding on mechanism of the biofilm formation also contributes to the control and application of biofilms. We want to establish the technology on the control and application of biofilms in food industry with scientific background in the future. This technology would be naturally applied to medical field and also to other various industries.
Funding
This study was supported by the twenty-first century COE program (Project leader: Professor Teruhiko Beppu) from MEXT, a Grant-in-Aid for Scientific Research from JSPS and other various grants described in each related publications.
Acknowledgments
This study was conducted in the Laboratory of Food Microbiology, Department of Food Bioscience and Biotechnology, College of Bioresource Sciences, Nihon University. I would like to thank all students, especially T. Kawarai, N. Narisawa, and S. Hirayama, of our laboratory, and all collaborators for their considerable support to this study. Especially, I would like to express my appreciation to Professor Makari Yamasaki, Professor Yasushi Morinaga, and Professor Hirokazu Ogihara of Nihon University for their continuing encouragement, helpful suggestions, and considerable supports to this study. I am also very grateful to Professor Yusaku Fujio, Professor Isao Hayakawa, and Professor Mitsuya Shimoda of Kyushu University for their encouragements during the early days of this study. This study was supported by the twenty-first century COE program (Project leader: Professor Teruhiko Beppu) from MEXT, a Grant-in-Aid for scientific research from JSPS and other various grants described in each related publications.
References
- Costerton JW, Stewart PS, Greenberg E. Bacterial biofilms: a common cause of persistent infections. Science. 1999;284:1318–1322.10.1126/science.284.5418.1318
- Davey ME, O'toole GA. Microbial biofilms: from ecology to molecular genetics. Microbiol. Mol. Biol. Rev. 2000;64:847–867.10.1128/MMBR.64.4.847-867.2000
- Ghannoum M, O’Toole GA. Microbial. Biofilms. Washington (DC): ASM Press; 2004.
- Kolter R, Greenberg EP. Microbial sciences: the superficial life of microbes. Nature. 2006;441:300–302.
- Furukawa S, Kuchma SL, O’Toole GA. Keeping their options open: acute versus persistent infections. J. Bacteriol. 2006;188:1211–1217.10.1128/JB.188.4.1211-1217.2006
- Ogawa A, Furukawa S, Fujita S, Mitobe J, Kawarai T, Narisawa N, Sekizuka T, Kuroda M, Ochiai K, Ogihara H, Kosono S, Watanabe H, Morinaga Y, Uematsu H, Senpuku H. Inhibition of Streptococcus mutans biofilm formation by Streptococcus salivarius FruA. Appl. Environ. Microbiol. 2011;193:421–428.
- Furukawa S, Matsufuji H, Anzai H, Ogihara H, Morinaga Y. Biofilm formation of food-related microorganisms and its control. J. Jpn. Soc. Food Eng. 2013;33:33–39. Japanese.
- Furukawa S, Ogihara H, Morinaga Y. Food and biofilms. In: Kasumi T, editor. Application of microorganisms and enzymes in food processing. Tokyo: Kanzume-Gijyutsu-Kenkyukai; 2010. p. 58–65. Japanese.
- Furukawa S, Akiyoshi Y, Komoriya M, Ogihara H, Morinaga Y. Removing Staphylococcus aureus and Escherichia coli biofilms on stainless steel by cleaning-in-place (CIP) cleaning agents. Food Control. 2010;21:669–672.10.1016/j.foodcont.2009.10.005
- Furukawa S, Akiyoshi Y, O’Toole GA, Ogihara H, Morinaga Y. Sugar fatty acid esters inhibit biofilm formation by food-borne pathogenic bacteria. Int. J. Food Microbiol. 2010;138:176–180.10.1016/j.ijfoodmicro.2009.12.026
- Okazaki S, Furukawa S, Ogihara H, Kawarai T, Kitada C, Komenou A, Yamasaki M. Microbiological and biochemical survey on the transition of fermentative processes in Fukuyama pot vinegar brewing. J. Gen. Appl. Microbiol. 2010;56:205–211.10.2323/jgam.56.205
- Koizumi Y, Tuzuki J, Nakamura H, Yanagida F. Studies on the pot vinegar. Part I. Role of floating Koji on the manufacturing process of pot vinegar. Studies on the pot vinegar Part I, Nippon Shokuhin Kogyo Gakkaishi. 1988;35:670–677. Japanese.10.3136/nskkk1962.35.10_670
- Haruta S, Ueno S, Egawa I, Hashiguchi K, Fujii A, Nagano M, Ishii M, Igarashi Y. Succession of bacterial and fungal communities during a traditional pot fermentation of rice vinegar assessed by PCR-mediated denaturing gradient gel electrophoresis. Int. J. Food Microbiol. 2006;109:79–87.10.1016/j.ijfoodmicro.2006.01.015
- Furukawa S, Yoshida K, Ogihara H, Yamasaki M, Morinaga Y. Mixed-species biofilm formation by direct cell–cell contact between brewing yeasts and lactic acid bacteria. Biosci. Biotechnol., Biochem. 2010;74:2316–2319.10.1271/bbb.100350
- Furukawa S, Nojima N, Yoshida K, Hirayama S, Ogihara H, Morinaga Y. The importance of inter-species cell–cell co-aggregation between Lactobacillus plantarum ml11-11 and Saccharomyces cerevisiae by4741 in mixed-species biofilm formation. Biosci. Biotechnol. Biochem. 2011;75:1430–1434.10.1271/bbb.100817
- Furukawa S, Nojima N, Nozaka S, Hirayama S, Satoh A, Ogihara H, Morinaga Y. Mutants of Lactobacillus plantarum ML11-11 deficient in co-aggregation with yeast exhibited reduced activities of mixed-species biofilm formation. Biosci. Biotechnol. Biochem. 2012;76:326–330.10.1271/bbb.110714
- Hirayama S, Furukawa S, Ogihara H, Morinaga Y. Yeast mannan structure necessary for co-aggregation with Lactobacillus plantarum ML11-11. Biochem. Biophys. Res. Commun. 2012;419:652–655.10.1016/j.bbrc.2012.02.068
- Nozaka S, Furukawa S, Sasaki M, Hirayama S, Ogihara H, Morinaga Y. Manganese ion increases lab-yeast mixed-species biofilm formation. Biosci. Microbiota. Food Health. 2014;33:79–84.10.12938/bmfh.33.79
- Furukawa S, Isomae R, Tsuchiya N, Hirayama S, Yamagishi A, Kobayashi M, Suzuki C, Ogihara H, Morinaga Y. Screening of lactic acid bacteria that can form mixed-species biofilm with Saccharomyces cerevisiae. Biosci. Biotechnol., Biochem. Forthcoming.
- Furukawa S, Yamasaki M, Morinaga Y. Considerations on the interaction between lactic acid bacteria and yeast in mixed-species biofilm, Kagaku To Seibutsu. 2010;48:8–10. Japanese.
- Furukawa S, Hirayama S, Fukase S, Ogihara H, Morinaga Y. Mixed-species biofilm formation of yeast, lactic acid bacteria and acetic acid bacteria, and its application. Seibutsu-Kogaku Kaishi. 2011;89:478–481. Japanese.
- Furukawa S, Katakura Y. Co-existence and symbiosis between lactic acid bacteria and yeast. Seibutsu-Kogaku Kaishi. 2012;90:188–191. Japanese.
- Furukawa S, Morinaga Y. Cell adherence of microorganisms and its application. Seibutsu-Kogaku Kaishi. 2012;90:197. Japanese.
- Furukawa S, Watanabe T, Toyama H, Morinaga Y. Significance of microbial symbiotic coexistence in traditional fermentation. J. Biosci. Bioeng. 2013;116:533–539.10.1016/j.jbiosc.2013.05.017
- Abe A, Hirayama S, Furukawa S. Cell adhesion of lactic acid bacteria and bio-production. Jpn. J. Lactic acid bact. 2013;24:37. Japanese.
- Furukawa S, Hirayama S, Morinaga Y. Co-existence and symbiosis of microorganisms and traditional fermentation. J. Brew. Soc. Japan. 2014;109:228–238. Japanese.
- Abe A, Furukawa S, Watanabe S, Morinaga Y. Yeasts and lactic acid bacteria mixed-specie biofilm formation is a promising cell immobilization technology for ethanol fermentation. Appl. Biochem. Biotechnol. 2013;171:72–79.10.1007/s12010-013-0360-6
- Furukawa S, Abe A, Fukase S, Hirayama S, Ogihara H, Morinaga Y. Bio-production using mixed-species biofilm. J. Brew. Soc. Japan. 2012;107:292–299. Japanese.
- Furukawa S, Morinaga Y. Bio-production in complex microbial system. Kagaku Kogaku Kaishi. 2012;76:695–698. Japanese.
- Morinaga Y, Furukawa S. Yeast and lactic acid bacteria mixed-species biofilm: properties and application. Bioindustry. 2013;30:49–57. Japanese.
- Furukawa S, Yanagihara K, Ohta E, Maki H, Morinaga Y. Biofilm studies in agricultural field, bacterial adherence & biofilm. Forthcoming.
- Shimazaki J, Furukawa S, Ogihara H, Morinaga Y. L-Tryptophan prevents Escherichia coli biofilm formation and triggers biofilm degradation. Biochem. Biophys. Res. Commun. 2012;419:715–718.10.1016/j.bbrc.2012.02.085
- Narisawa N, Furukawa S, Ogihara H, Yamasaki M. Estimation of the biofilm formation of Escherichia coli K-12 by the cell number. J. Biosci. Bioeng. 2005;99:78–80.10.1263/jbb.99.78
- Kawarai T, Furukawa S, Narisawa N, Hagiwara C, Ogihara H, Yamasaki M. Biofilm formation by Escherichia coli in hypertonic sucrose media. J. Biosci. Bioeng. 2009;107:630–635.10.1016/j.jbiosc.2009.01.018
- Matsumura K, Furukawa S, Ogihara H, Morinaga Y. Roles of multidrug efflux pumps on the biofilm formation of Escherichia coli K-12. Biocontrol Sci. 2011;16:69–72.10.4265/bio.16.69
- Watanabe T, Furukawa S, Hirata J, Koyama T, Ogihara H, Yamasaki M. Inactivation of Geobacillus stearothermophilus spores by high-pressure carbon dioxide treatment. Appl. Environ. Microbiol. 2003;69:7124–7129.10.1128/AEM.69.12.7124-7129.2003
- Kawarai T, Wachi M, Ogino H, Furukawa S, Suzuki K, Ogihara H, Yamasaki M. SulA-independent filamentation of Escherichia coli during growth after release from high hydrostatic pressure treatment. Appl. Microbiol. Biotechnol. 2004;64:255–262.10.1007/s00253-003-1465-6
- Watanabe T, Furukawa S, Kitamoto K, Takatsuki A, Hirata R, Ogihara H, Yamasaki M. Vacuolar H+-ATPase and plasma membrane H+-ATPase contribute to the tolerance against high-pressure carbon dioxide treatment in Saccharomyces cerevisiae. Int. J. Food Microbiol. 2005;105:131–137.10.1016/j.ijfoodmicro.2005.05.008
- Kawarai T, Ogihara H, Aono R, Kishima M, Inagi Y, Irie A, Ida A, Furukawa S, Makari Y. High hydrostatic pressure treatment impairs AcrAB-TolC pump resulting in the differential loss of deoxycholate-tolerance in Escherichia coli. J. Biosci. Bioeng. 2006;100:613–616.
- Watanabe T, Furukawa S, Kawarai T, Wachi M, Ogihara H, Yamasaki M. Cytoplasmic acidification may occur in high-pressure carbon dioxide-treated Escherichia coli K12. Biosci. Biotechnol. Biochem. 2007;71:2522–2526.10.1271/bbb.70313
- Narisawa N, Furukawa S, Kawarai T, Ohishi K, Kanda S, Kimijima K, Negishi S, Ogihara H, Yamasaki M. Effect of skimmed milk and its fractions on the inactivation of Escherichia coli K12 by high hydrostatic pressure treatment. Int. J. Food Microbiol. 2008;124:103–107.10.1016/j.ijfoodmicro.2008.01.016
- Arai S, Kawarai T, Arai R, Yoshida M, Furukawa S, Ogihara H, Yamasaki M. Cessation of cytokinesis in Schizosaccharomyces pombe during growth after release from high hydrostatic pressure treatment. Biosci. Biotechnol., Biochem. 2008;72:88–93.10.1271/bbb.70451
- Furukawa S. Mechanism of inactivation of microorganisms by non-thermal sterilization. Nippon Shokuhin Kagaku Kogaku Kaishi. 2008;55:1–5.10.3136/nskkk.55.1
- Furukawa S, Ogihara H, Morinaga Y. New development of non-thermal sterilization process. New Food Ind. 2014;56:6–14. Japanese.
- Matsufuji H, Furukawa S, Teranishi K, Kawaharada K, Chino M, Yamagata K, Ogihara H, Yamasaki M. Effects of nonthermal processes on the inactivation of microorganisms and antioxidants in minimally processed vegetables. Food Sci. Technol. Res. 2009;15:153–162.10.3136/fstr.15.153
- Furukawa S, Matsufuji H, Ogihara H. New disinfection method for cut vegetables with protecting functional components. Shokuhin Kogyo. 2008;51:27–37. Japanese.
- Ogihara H, Morimura K, Uruga H, Miyamae T, Kogure M, Furukawa S. Inactivation of food-related microorganisms in liquid environment by pulsed xenon flash light treatment system. Food Control. 2013;33:15–19.10.1016/j.foodcont.2013.02.012
- Kawarai T, Furukawa S, Ogihara H, Yamasaki M. Mixed-species biofilm formation by lactic acid bacteria and rice wine yeasts. Appl. Environ. Microbiol. 2007;73:4673–4676.10.1128/AEM.02891-06
- Yamamoto K, Nakayama J, Igimi S, Ishikawa M, Saito T, Sasaki T, Sasaki Y, Sato E, Takano T, Morita H, Yasui H, Yanagida T, Yokota A, Watanabe K. Nyusankin-to-bifizusukin-no-saiensu [Science of lactic acid bacteria and bifidobacteria]. Kyoto: Kyoto University Press; 2010. Japanese.
- Wood BJB. Microbiology of fermented foods. London: Blackie Academic and Professional; 1998.
- Tochikura T, Yamada H, Beppu T, Soda K. Hakko-handbook. Tokyo: Kyoritsu Shuppan; 2001:445–446. Japanese.
- Hirayama S, Shimizu M, Tsuchiya N, Furukawa S, Watanabe D, Shimoi H, Takagi H, Ogihara H, Morinaga Y. Awa1p on the cell surface of sake yeast inhibits biofilm formation and the co-aggregation between Sake yeasts and Lactobacillus plantarum ML11-11. J. Biosci. Bioeng. Forthcoming.
- Kjelleberg S, Givskov M. The biofilm mode of life; mechanisms and adaptation. Norfolk: Horizon Bioscience; 2007.
- Romeo T. Bacterial biofilms. Hidelberg: Springer; 2008.10.1007/978-3-540-75418-3
- Furukawa S, Usui Y, Shimazaki J, Morinaga Y. Nucleic acids and amino acids control Escherichia coli biofilm formation, 60th nobel conference on biofilm formation, its clinical impact and potential treatment. Stockholm; 2013. p. 67.