Abstract
Dipeptides have unique physiological functions. This study focused on the salt-taste-enhancing dipeptide Met-Gly. BL00235, an l-amino acid ligase from Bacillus licheniformis NBRC12200, synthesizes Met-Gly as a major product as well as Met-Met as a by-product. To alter the substrate specificity of BL00235 and synthesize Met-Gly selectively, we chose to alter Pro85 residue based on the BL00235 crystal structure. We predicted that Met might be not recognized as a C-terminal substrate by occupying the space around C-terminal substrate. Pro85 was replaced with Phe, Tyr, and Trp, which have bulky aromatic side chains, by site-directed mutagenesis. These mutants lost the capacity to synthesize Met-Met, during the synthesis of Met-Gly. Furthermore, they did not synthesize Met-Met, even when methionine was used as a substrate. These results show that the amino acid residue at position 85 has a key role in C-terminal substrate specificity.
Graphical abstract
We achieved our goal of synthesizing Met-Gly selectively, the salt-taste-enhancing dipeptide, using Lal by site-directed mutagenesis based on the crystal structure.
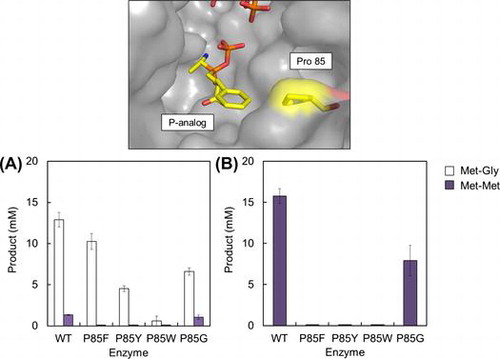
Dipeptides are composed of two amino acids joined by a peptide bond. Some dipeptides have unique physical properties and physiological functions that their constitute amino acids do not. For example, Ile-Trp,Citation1) Arg-Phe,Citation2) and Gly-TyrCitation3) have antihypertensive effects, and Ser-HisCitation4) has a sedative effect. Dipeptides also reportedly have taste-improving effects. The best-known tasty dipeptide is the artificial sweetener aspartame (Asp-Phe methyl ester). Aspartame is used extensively in beverages, desserts, and as a tabletop sweetener and has a sweetness that is 200 times that of sucrose.Citation5) Some dipeptides have taste-enhancing effect. Ala-Leu, Leu-Gln, and Leu-Thr are known as a kokumi-imparting effectCitation6), and Glu-Thr enhances the salt taste.Citation7) In recent years, consumers have become conscious about reducing sodium intake. Sodium is an essential nutrient for human beings; however, excessive salt intake increases blood pressure Citation8,9) and causes cardiovascular disease.Citation10) Salt consumption above recommended levels contributed to nearly 1.65 million deaths from cardiovascular disease worldwide in 2010.Citation11) In addition, salt intake is associated with gastric cancer.Citation12) Therefore, reduction in the use of salt is necessary, and the development of salt taste enhancers is expected to reduce the need for salt and keep tasty at the same time.
Recently, we identified the dipeptide of the new salt taste enhancer, Met-Gly.Citation13) The dipeptides we used to perform our screening were synthesized by l-amino acid ligase (Lal, EC 6.3.2.28). Lal, a member of the ATP-dependent carboxylate-amine/thiol ligase superfamily,Citation14) is a microbial enzyme that catalyzes dipeptide synthesis from unprotected l-amino acids by hydrolysis of ATP to ADP.Citation15) Lal was first reported in YwfE from Bacillus subtilis 168,Citation15) and its discovery led to identification of other Lals, including RSp1486a from Rastonia solanacearum JCM 10498,Citation16) BL00235 from Bacillus licheniformis NBRC 12200,Citation17) RizA from B. subtilis NBRC 3134,Citation18) and TabS from Pseudomonas syringae NBRC 14081.Citation19) Each of these Lal has substrate specificity characteristics. YwfE allows only Ala, Gly, Ser, Thr, and Met as the N-terminal substrate, but accommodates a wide variety of amino acids as the C-terminal substrate.Citation15) BL00235 accepts only Met and Leu as the N-terminal substrates and prefers small residues such as Ala and Ser as the C-terminal substrate.Citation17) YwfE has strict specificity for Arg as the N-terminal substrate.Citation18) By contrast, TabS has the broadest substrate specificity of any known Lal and can synthesize many kinds of dipeptides.Citation19) We have used to synthesize dipeptides in a search for salt-taste-enhancing dipeptides.Citation13) We previously reported that Met-Gly and Met-Met are produced by TabS, with the amount of Met-Met was exceeding that of Met-Gly.Citation13) If Met-Gly is to be supplied by Lal efficiently, it is necessary to synthesize it selectively without by-products.
In this study, we focused on BL00235, which has the substrate specificity characteristics described above,Citation17) to synthesize Met-Gly. First, we confirmed that BL00235 synthesizes Met-Gly as a major product and found that Met-Met is synthesized as well. We then attempted to alter the substrate specificity of BL00235 based on its structure (PDB ID: 3VOT) and synthesize Met-Gly selectively.Citation20) Alignment of amino acid sequences of five Lals are shown in Fig. . Glu84 residue of magnesium ion.Citation20,21) Shomura et al.Citation22) showed Asn108 and Leu110 residues of YwfE located on both sides of Glu109 are determine the C-terminal substrates based on its structure (PDB ID: 3VMM). Therefore, we focused on Phe83 and Pro85 residues of BL00235 corresponding to Asn108 and Leu110 residues of YwfE. As shown in Fig. , Pro85 and Phe83 residues narrow the space around the C-terminal substrate, and BL00235 prefers Gly, Ala, and Ser, which have slim side chains, as C-terminal substrates.Citation20) We deduced that Met is not recognized as a C-terminal substrate at all by taking up more space. According to this hypothesis, the Pro85 residue was replaced with bulky aromatic amino acids by site-directed mutagenesis. This approach yielded the P85F mutant (Pro85 was replaced with Phe) and the P85Y mutant (Pro85 was replaced with Tyr), which lost the capacity to synthesize Met-Met, during selective Met-Gly synthesis. Our results demonstrated a new and useful application for Lal.
Fig. 1. Alignment of primary structures of Lals.

Fig. 2. Structure of Ywfe in complex with the phosphinate L-alanyl-L-phenylalanine analog (P-analog) Citation22) is superimposed onto the BL00235 crystal structure (PDB code, 3VOT).Citation21)
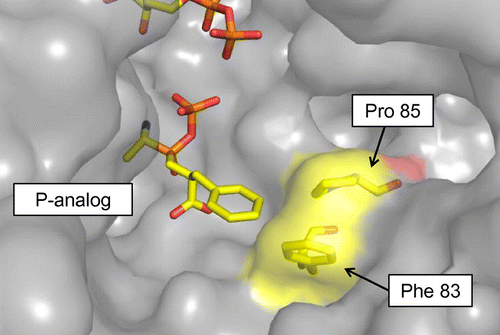
Materials and methods
Materials
All of the chemicals used in this study are commercially available and were of chemically pure grade.
Preparation of enzymes
The genes encoding BL00235 and TabS were previously cloned into the pET21d(+) or pET28a(+) vectors, respectively.Citation17,19) Recombinant Escherichia coli BL21 (DE3) cells were precultivated in 3 mL LB medium (1% bacto tryptone, 0.5% yeast extract, 1% NaCl) supplemented with 50 g/mL ampicillin (BL00235) or 30 g/mL kanamycin (TabS) at 37 °C for 5 h with shaking at 160 rpm. The cultivated cells were transferred to 150 mL (BL00235) or 200 mL (TabS) LB medium supplemented with 50 g/mL ampicillin (BL00235) or 30 g/mL kanamycin (TabS) and were cultivated with shaking at 37 °C at 120 rpm. After cultivation for 2 h (BL00235) or 1 h (TabS), 0.1 mM isopropyl-β-D-thiogalactopyranoside was added to the medium, and additional cultivation took place at 25 °C for 19 h with shaking at 160 rpm. The cells were harvested by centrifugation (4160 × g, 10 min, 4 °C) and washed twice with 100 mM Tris-HCl buffer (pH 8.0). After washing, the cells were resuspended in 100 mM NaHCO3–Na2CO3 buffer (pH 9.0) and then disrupted with sonication at 4 °C. Cellular debris was removed by centrifugation (20,000 × g, 30 min, 4 °C), and the supernatant was purified with a His GravitrapTM affinity column (GE Healthcare, Buckinghamshire, UK). The active fractions were desalted with a PD-10 column (GE Healthcare, Buckinghamshire, UK) and eluted with 100 mM NaHCO3–Na2CO3 buffer (pH 9.0).
Site-directed mutagenesis
Mutations were introduced by polymerase chain reaction with KOD-Plus-Neo DNA polymerase (Toyobo, Osaka, Japan) based on the method of a QuikChange site-directed mutagenesis kit (Agilent Technologies, CA, USA), and the primers listed in Supplemental Table S1. The generation of the desired mutations was confirmed through DNA sequencing, and the mutations were with DNA sequencing. Each plasmid was introduced into E. coli BL21 (DE3). The recombinant enzymes were prepared as described above.
Enzyme assay
The standard reaction mixtures (300 μL) contained 0.5 mg/mL of recombinant enzyme, 20 mM ATP, 20 mM MgSO4·7H2O, and amino acid substrates (20 mM Met and Gly or 40 mM Met) in 50 mM NaHCO3–Na2CO3 buffer (pH 9.0). The reaction was performed at 30 °C for 20 h. To confirm the linear relationship between the amount of Met-Gly and the concentration of wild-type BL00235 or mutant, we performed the reaction for 60 min with various concentrations of each protein (0.1–0.5 mg/mL) under standard conditions. To determine the kinetic parameters, we performed the reaction with various concentrations of Met (5–100 mM), Gly (2–60 mM), or ATP (0.5–15 mM) under standard conditions. The enzyme concentration was 0.25 mg/mL, and the reaction time was 60 min.
Analysis
The amounts of dipeptides were analyzed by HPLC (L-2000 series; Hitachi High Technologies, Tokyo, Japan). The details of the analytical procedure were described previously.Citation23)
Results
Synthesis of Met-Gly with TabS or BL00235
We found that Met-Gly has a salt-enhancing effect by synthesizing dipeptides using TabS and evaluating them with sensory tests and taste sensors.Citation13) However, TabS synthesized a smaller amount of Met-Gly than Met-Met.Citation20) Therefore, we compared the amounts of Met-Gly and Met-Met synthesized by TabS to those synthesized with BL00235, which accepts only Met or Leu as N-terminal substrates (Fig. ). These Lals produced opposite ratios of Met-Gly to Met-Met, and Met-Gly was synthesized as a major product by BL00235. In addition, neither the wild-type BL00235 nor the P85F mutant could synthesize Gly-Gly and Gly-Met. Therefore, we confirmed that BL00235 was a suitable Lal for Met-Gly synthesis.
Site-directed mutagenesis of BL00235 and Lal activity of BL00235 mutants
To alter substrate specificity and synthesize Met-Gly more selectively, we replaced the Pro85 residue of BL00235 with Phe (P85F), Tyr (P85Y), or Trp (P85W), which have bulky aromatic side chains. P85G (Pro85 was replaced with Gly) was also constructed as a mutant in which the replacement was an amino acid with a small side chain. The expression and purification of the wild-type BL00235 and BL00235 mutants were carried out, and the dipeptide-synthesizing reaction of each protein was performed using 20 mM Met and Gly, or 40 mM Met as substrates (Fig. ). The amounts of Gly-Gly and Gly-Met were not shown in this figure because they were not detected. P85F and P85Y mutants lost the capacity to synthesize Met-Met, but they synthesized Met-Gly from Met and Gly (Fig. (A)). Furthermore, Met-Met was not detected in the P85F and P85Y mutant reaction mixtures that contained Met (Fig. (B)). The P85W mutant synthesized a small amount of Met-Gly from Met and Gly, but did not synthesize Met-Met from Met and Gly, or from Met. By contrast, the wild-type BL00235 and the P85G mutant showed similar substrate specificities, but the amount of dipeptide synthesized by the P85G mutant was smaller than that by the wild-type BL00235. These results showed that the P85F and P85Y mutants synthesize Met-Gly selectively. We used the P85F mutant for further analysis because it synthesized more than twice the amount of Met-Gly as the P85Y mutant did and was considered more useful for Met-Gly synthesis.
Fig. 4. Synthesis of Met-Gly (white bars) or Met-Met (dark bars) by the wild-type BL00235 or mutants.
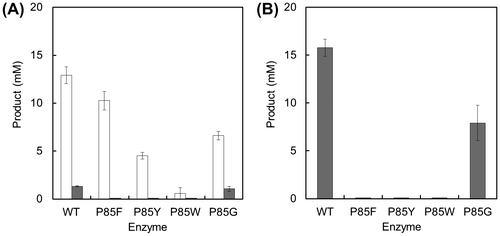
Characterization of P85F mutant
We first determined the kinetic properties of Met-Gly synthesis (Fig. ). The apparent Kms values for Met, Gly, and ATP of the wild-type BL00235 were 17.1 ± 1.0, 3.9 ± 0.4, and 3.1 ± 0.4, respectively, and those of the P85F mutant were 31.4 ± 4.8, 10.3 ± 0.8, and 4.0 ± 0.3 mM, respectively. The Vmax values for Met-Gly synthesis by the wild-type BL00235 and the P85F mutant were 407.1 ± 40.8 and 580.0 ± 40.1 nmol min−1 mg protein−1, respectively.
Fig. 5. Kinetic analysis of Met-Gly-synthesizing reaction by the wild-type BL00235 or the P85F mutant.
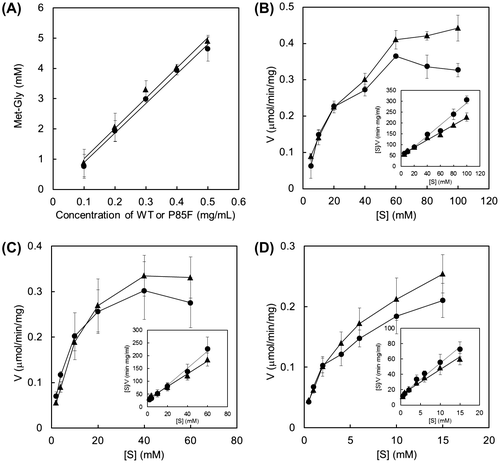
Next, we examined the relationship between the amino acid substrate concentration and the amount of Met-Gly obtained with the P85F mutant (Fig. ). The reaction was performed with various concentrations of Met or Gly. The amount of Met-Gly was increased as the concentration of Met increased, whereas it was barely affected by changes in Gly concentration. In addition, Met-Met was not synthesized in any of the reaction mixtures (data not shown).
Fig. 6. Effect of substrate concentration on Met-Gly synthesis by the P85F mutant.
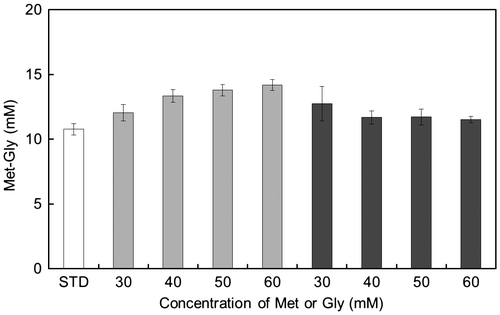
Discussion
Few reports on the alteration of Lals with structure-based site-directed mutagenesis have been published.Citation21,22) The objectives of mutant construction can be roughly divided into two types: (1) to reveal the catalytic functionCitation21,22) and (2) to alter substrate specificity to synthesize useful dipeptides.Citation21) This study had the latter objective. Tsuda et al. Citation21) showed that Trp332 affects the N-terminal substrate specificity of YwfE and suggested that alteration of substrate specificity of Lal has the possibility of functional dipeptides synthesis. However, practically, no study has demonstrated that changing specificity of Lals by a single mutation leads to dipeptide synthesis as planned. Therefore, the present report is the first of the successful selective synthesis of a useful dipeptide using Lals by site-directed mutagenesis.
In this study, we tried to synthesize a salt-taste-enhancing dipeptide, Met-Gly, efficiently with TabS or BL00235. Our previously reported Pi analysis showed that TabS tends to choose Met as an N-terminal substrate and Gly as C-terminal substrate.Citation19) We hypothesized that TabS synthesizes Met-Gly as a major product, but experimental results showed that Met-Met was the major product (Fig. ). The results of the present study showed that the types of dipeptides synthesized by TabS changed according to the amino acids combination. Hence, we selected BL00235, which synthesized Met-Gly more efficiently. The wild-type BL00235 synthesized Met-Gly as a major product, but also synthesized a small amount of Met-Met (Fig. ). The structure of BL00235 suggests that the C-terminal substrate preference for amino acids with small side chains is related to the Phe83 and Pro85 residues.Citation20) We predicted that Met is not recognized as a C-terminal substrate at all by occupying more space around C-terminal substrate than the space of the wild-type BL00235 and that Met-Gly can be synthesized selectively without the synthesis of Met-Met. Therefore, we selected Phe, Tyr, and Trp, which contain bulky aromatic side chains, to replace Pro85 residue.
Indeed, the P85F and P85Y mutants achieved selective Met-Gly synthesis without the synthesis of Met-Met (Fig. ). The P85F mutant synthesized more than twice as much Met-Gly as the P85Y mutant did; the P85W mutant had low activity. Interestingly, the amount of Met-Gly differed the three mutants according to the size of the side chain. We believe that the side chain of Phe at the 85 position might be suitable for synthesizing Met-Gly selectively. On the contrary, Gly does not enter the pocket of the P85Y mutant as easily as that of the P85F mutant as a C-terminal substrate because the Tyr side chain is slightly too large compared with that of Phe. Furthermore, the P85W mutant, which has the largest side chain of the three amino acids tested, prevents even Gly as a C-terminal substrate from entering the binding pocket and loses most of its capacity to synthesize Met-Gly as a result. Although there may be other explanations for those differences, our results support the hypothesis that amino acid residues with bulky side chains at position 85 effect C-terminal substrate specificity.
The P85F mutant increased the apparent Kms values for Met, Gly, and ATP, suggesting that the affinity with substrate for the P85F mutant is low compared with that for the wild-type BL00235. By contrast, the Vmax value for Met-Gly synthesis by the P85F mutant was higher than that by the wild-type BL00235. According to the analysis of the time course of Met-Gly synthesis, the amount of Met-Gly synthesized by the P85F mutant was appear to be slightly higher at the initial stage of reaction, but it was lower than that by the wild-type BL00235 gradually (Supplemental Fig. S1). We think these characteristics of the P85F mutant affect the Kms and Vmax values.
Compared with that or the wild type, the amount of Met-Gly synthesized by the P85F mutant was much lower. However, the amount of Met-Gly increased when the amount of Met was increased (see Fig. ). On the contrary, the amount of Met-Met synthesized by wild-type BL00235 increased with an increase in Met concentration (Supplemental Fig. S2). These results also confirmed that the Met in the P85F mutant is not recognized as a C-terminal substrate.
Studies of Lals have focused on finding new Lals, which have thus far been identified in YwfE, BL00235, TabS, and so others.Citation15–19) We are now exploring the application of Lals. In this study, we achieved our goal of synthesizing the salt-taste-enhancing dipeptide Met-Gly selectively. This advance will contribute to the synthesis of other useful dipeptides without by-products and makes Lals easier to use than ever before.
Author contribution
K.K. and H.K. designed research; H.K. performed research; H.K. analyzed date; and H.K. and K.K. wrote the article.
Supplemental material
The supplemental material for this study is available at http://dx.doi.org/10.1080/09168451.2015.1056511.
Disclosure statement
No potential conflict of interest was reported by the authors.
Funding
This work was supported by the Japan Society for the Promotion of Science (JSPS) [grant number 26289318].
150190R2_final_supplemental.pptx
Download MS Power Point (147.7 KB)References
- Enari H, Takahashi M, Tada M, et al. Identification of angiotensin I-converting enzyme inhibitory peptides derived from salmon and their antihypertensive effect. Fisheries Sci. 2010;74:911–920.
- Kagebayashi T, Kotani N, Yamada Y, et al. Novel CCK-dependent vasorelaxing dipeptide, Arg-Phe, decreses blood pressure and food intake in rodents. Mol. Nutr. Food Res. 2012;56:1456–1463.10.1002/mnfr.v56.9
- Uchida R, Nakahara T, Hanada Y, et al. Hypotensive effect and safety of less-sodium soy sauce containing soybean peptides in high-normal blood pressure or mild hypertensive subjects. Jpn. Pharmacol. Ther. 2008;36:837–850.
- Tsuneyoshi Y, Tomonaga S, Yamane H, et al. Central administration of L-Ser-L-His and L-Ile-L-His induced sedative effects under an acute stressful condition in chicks. Lett. Drug. Des. Discov. 2008;5:65–68.
- Marinovich M, Galli CL, Bosetti C, et al. Aspartame, low-calorie sweeteners and disease: regulatory safety and epidemiological issues. Food Chem. Toxicol. 2013;60:109–115.10.1016/j.fct.2013.07.040
- Liu J, Song H, Liu Y, et al. Discovery of kokumi peptide from yeast extract by LC-Q-TOF-MS/MS and sensomics approach. J. Sci. Food Agric. 2015 [cited 2015 Jan 19]. Available from http://onlinelibrary.wiley.com/doi/10.1002/jsfa.7058/abstract.
- Shimono M, Sugiyama K, Nippon Suisan Kaisha, Ltd., Salty taste enhancer and food or drink containing the same. United States patent 8,409,653. 2013 Apr 2.
- Prior I, Evans JG, Harvey HPB, et al. Sodium intake and blood pressure in two polynesian populations. New Engl. J. Med. 1068;279:515–520.
- Kesteloot H, Huang DX, Li YL, et al. The relationship between cations and blood pressure in the People’s Republic of China. Hypertension. 1987;9:654–659.10.1161/01.HYP.9.6.654
- Takachi R, Inoue M, Shimazu T, et al. Consumption of sodium and salted foods in relation to cancer and cardiovascular disease: the Japan public health center-based prospective study. Am. J. Clin. Nutr. 2010;91:456–464.10.3945/ajcn.2009.28587
- Mozaffarian D, Fahimi S, Singh GM, et al. Global sodium consumption and death from cardiovascular causes. New Engl. J. Med. 2014;371:634–634.
- Tsugane S, Sasazuki S, Kobayashi M, et al. Salt and salted food intake and subsequent risk of gastric cancer among middle-aged japanese men and women. Br. J. cancer. 2004;90:128–134.10.1038/sj.bjc.6601511
- Kino H, Kakutani M, Hattori K, et al. Screening of salt taste enhancing dipeptide based on a new strategy with l-amino acid ligase. Nippon Shokuhin Kagaku Kogaku Kaishi. 2015;62:274–281 (in Japanese).
- Galperin MY, Koonin EV. A diverse superfamily of enzymes with ATP-dependent carboxylate-amine/thiol ligase activity. Protein Sci. 1997;6:2639–2643.
- Tabata K, Ikeda H, Hashimoto S. Ywfe in Bacillus subtilis codes for a novel enzyme, l-amino acid ligase. J. Bacteriol. 2005;187:5195–5202.10.1128/JB.187.15.5195-5202.2005
- Kino K, Noguchi A, Arai T, et al. Dipeptide synthesis by l-amino acid ligase from Ralstnia solanacearum. Biochem. Biophys. Res. Commun. 2008;371:436–540.
- Kino K, Noguchi A, Yagasaki M. A novel l-amino acid ligase from Bacillus licheniformis. J. Biosci. Bioeng. 2008;106:313–315.10.1263/jbb.106.313
- Kino K, Kotanaka Y, Arai T, et al. A novel l-amino acid ligase from Bacillus subtilis NBRC3134, a microorganism producing peptide-antibiotic rhizocticin. Biosci. Biotechnol. Biochem. 2009;73:901–907.10.1271/bbb.80842
- Arai T, Arimura Y, Ishikura S, et al. l-amino acid ligase from Pseudomonas syringae producing tabtoxin can be used for enzymatic synthesis of various functional peptides. Appl. Environ. Microbiol. 2013;79:5023–5029.10.1128/AEM.01003-13
- Suzuki M, Takahashi Y, Noguchi A, et al. The structure of l-amino-acid ligase from Bacillus licheniformis. Acta. Cryst. 2012;D68:1535–1540.
- Tsuda T, Asami M, Koguchi Y, et al. Single mutation alters the substrate specificity of l-amino acid ligase. Biochemistry. 2014;53:2650–2660.10.1021/bi500292b
- Shomura Y, Hinokuchi E, Ikeda H, et al. Structure and enzymatic characterization of BacD, an l-Amino acid dipeptide ligase from Bacillus subtilis. Protein Sci. 2012;21:706–716.
- Arai T, Kino K. A cyanophycin synthetase from Themosynechococcus elongatus BP-1 catalyzes primer-independent cyanophysin synthesis. Appl. Microbiol. Biotechnol. 2008;81:69–78.10.1007/s00253-008-1623-y