Abstract
Previously, we found that enteric lactoferrin (eLF) could reduce the visceral fat accumulation known to associate strongly with metabolic syndrome symptoms and consequently with an increased risk of atherosclerosis. In this study, the atherosclerosis-preventive potential of LF was assessed in a high-fat and high-cholesterol diet (HFCD)-induced hypercholesterolemia and atherosclerosis model using Microminipig™. Eight-week orally administered eLF remarkably reduced the HFCD-induced serum total and low-density lipoprotein cholesterol levels but not high-density lipoprotein cholesterol levels. A histological analysis of 15 arteries revealed that eLF systemically inhibited the development of atherosclerotic lesions. Pathway analysis using identified genes that characterized eLF administration in liver revealed significant changes in the steroid biosynthesis pathway (ssc00100) and all affected genes in this pathway were upregulated, suggesting that cholesterol synthesis inhibited by HFCD was recovered by eLF. In summary, eLF could potentially prevent the hypercholesterolemia and atherosclerosis through protecting homeostasis from HFCD-induced dysfunction of cholesterol metabolism.
Atherosclerosis is a characteristic risk factor of cardiovascular disease and is closely related to metabolic syndrome (MetS) symptoms such as high blood sugar, hyperlipidemia, and hypertension.Citation1,2) Atherosclerosis is characterized by the accumulation of lipids within macrophages, leading to inflammation and the formation of atheroma plaques. A link between this process and oxidative modifications for circulating low-density lipoproteins (LDL) has been recognized, and often no symptoms are apparent until the arterial damage has become sufficiently severe to restrict blood flow. These silent disease characteristics increase the risk of atherosclerosis progression; therefore, it is important to establish therapeutic and preventive measures.
Lactoferrin (LF), a member of the transferrin family, is a well-known multi-functional glycoprotein with antibacterial, antiviral, immunostimulatory, antioxidant, and cancer-preventive potential.Citation3–7) As a natural component of breast milk, LF is considered safe. Therefore, it has been categorized as “generally recognized as safe” in the USA and has been approved as a food additive in Japan.
We found that enteric LF (eLF) improved lipid metabolism disorders. An orally administered enteric-coated bovine LF tablet (300 mg/day as LF) significantly reduced visceral fat accumulation, a phenomenon that has been well established as the main cause of MetS in a double-blind clinical trial.Citation8) From the results of in vitro experiments conducted in preadipocytes derived from rat mesenteric fat, the antiadipogenic and lipolytic effects of LF were proposed as possible effector mechanisms.Citation9,10) Moreno-Navarrete et al. and Yagi et al. also demonstrated the antiadipogenic activity of LF in the MC3T3-G2/PA6 and 3T3-L1 cell line, respectively.Citation11,12) In further investigations, we found that pepsin-degraded LF did not exhibit the antiadipogenic and lipolytic activities, suggesting that the enteric formulation was necessary for efficacy against lipid metabolism disorders.Citation9,10)
We further evaluated the effect of LF on hypercholesterolemia in a preliminary animal study and found that administering LF via the drinking water inhibited the progression of high-cholesterol diet-induced hypercholesterolemia in mice (unpublished data). Takeuchi et al. also reported that LF supplementation yielded significant reductions in the serum total cholesterol and triglyceride (TG) levels in mice fed a normal diet.Citation13) These results suggest that LF may possess inhibitory activity against hypercholesterolemia-induced atherosclerosis. However, a previous report found that mice are generally resistant to high-cholesterol diet-induced atherosclerosis and that cholesterol metabolism differs considerably between mice and humans.Citation14) Therefore, experimental human atherogenesis studies will require an appropriate animal model that mimics human physiology and pathology because the pathogenesis of this disease includes both genetic and environmental factors.
Swine represent a potentially useful animal model because the anatomy, physiology, and feeding and sleep habits are very similar to those of humans.Citation15) Recently, it is reported the development of a hypercholesterolemia-induced atherosclerosis model involving the smallest available miniature pigs, “Microminipigs™” (MMPigs), which differ from experimental miniature pig strains such as Clawn and Göttingen.Citation16–18) A different study found that the expression of genes regulating cholesterol metabolism in liver such as low-density lipoprotein receptor (LDLR), 3-hydroxy-3-methylglutaryl-CoA reductase (HMGCR), and sterol regulatory element-binding protein 2 (SREBP-2) were correlated and markedly downregulated following the consumption of high-fat and high-cholesterol diet (HFCD).Citation19) The regulation of these genes and the LDL cholesterol-rich lipid profile in MMPigs are therefore very similar to those observed in humans. In this study, we investigated the effect of eLF on HFCD-induced hypercholesterolemia and atherosclerosis in MMPigs to clarify the antiatherogenic potential of eLF.
Materials and methods
Materials
Commercially available bovine LF was purchased from FrieslandCampina DMV (LE, Amersfoort, Netherlands). According to the certificate of analysis, typical protein purity is 98%.
Animals and diet
Sixteen-week-old male MMPigs were maintained in a special facility with the following environmental conditions: room temperature, 24 ± 3 °C; relative humidity, 50% ± 20%; and light/dark cycle, 12-h each. Tap water was available ad libitum. The animals were fed 3% of its own body weight in a special diet once a day in the morning and administered 4 enteric capsules (E-capsule, major axis: 1.6 cm, minor axis: 0.6 cm; Sanseiiyaku, Shizuoka, Japan) filled with or without LF after the meal. Nine MMPigs were divided into 3 groups (3 animals per group). One group (Control group; n = 3) was fed a normal chow diet (Kodakara 73; Marubeni Nisshin Feed, Tokyo, Japan) and administered empty enteric capsules. A second group was fed a HFCD and administered empty enteric capsules (HFCD group). The third group was fed a HFCD and administered capsules filled with LF (500 mg LF/body/day, 125 mg LF/capsule; HFCD + eLF group). According to the product specification, normal chow diet was composed of less than 9.0% carbohydrate (w/w), greater than 15.0% protein (w/w), greater than 2.0% fat (w/w), and less than 9.0% fiber (w/w). HFCD comprised 6% lard (w/w) (Miyoshi Oil & Fat, Tokyo, Japan) and 0.5% cholesterol (w/w) (Wako Pure Chemical Industries, Osaka, Japan) combined with a normal chow diet. Body weight was measured every week. After 8 weeks, all MMPigs were anesthetized and sacrificed via bilateral auxillary artery exsanguination. All protocols were approved by the Ethics Committee of Animal Care and Experimentation, Kagoshima University (A09001), and all research was performed according to the institutional guidelines for animal experiments and in compliance with the Japanese law concerning the protection and control of animals (Law No. 105 and Notification No. 6). This study was also performed in accordance with the animal welfare bylaws of Shin Nippon Biomedical Laboratories Ltd., a facility with full accreditation from the Association for Assessment and Accreditation of Laboratory Animal Care International and approval from the International Animal Care and Use Committee.
Biochemical analysis
Blood samples were collected every 2 weeks for lipid profiling. The total cholesterol, LDL cholesterol, high-density lipoprotein (HDL) cholesterol, and TG levels were analyzed with an automated agarose gel electrophoresis apparatus (Epalyzer 2; Helena Laboratories, Saitama, Japan).
Extraction of hepatic lipids
Lipids were extracted from a portion of the liver according to the Folch method. Concentrated lipids were reconstituted in 2-propanol, and the TG and total cholesterol levels were analyzed using triglyceride E-Test Wako and total cholesterol E-Test Wako, respectively (Wako Pure Chemical Industries, Osaka, Japan).
Pathological examination
During the necropsy, the aorta, arteries, liver, spleen, heart, kidney, and omental fat were removed from each animal. The liver, spleen, heart, kidney, and omental fat were weighed. Each aorta and artery was cut into about 3 mm sections, which were fixed in 10% phosphate-buffered formalin and routinely processed as 4 μm thick paraffin-embedded tissue sections followed by staining with hematoxylin and eosin. The degree of atherosclerosis development in each animal was classified according to the Stary Type Classification.Citation20) Immunostaining for atherosclerotic lesions was performed on the paraffin-embedded sections using primary antibodies and Envision kit (Dako Cytomation, Kyoto, Japan). The primary antibodies and concentrations were as follows: anti-ionized calcium-binding adaptor molecule-1 (Iba-1) polyclonal antibody (1:250; Wako Pure Chemical Industries Ltd.) and mouse monoclonal α-smooth muscle actin (α-SMA) (1:100; clone 1A4; Dako Cytomation, Kyoto, Japan).
DNA microarray analysis
Total RNA was isolated from the liver samples of HFCD and HFCD + eLF animals using TRIzol (Invitrogen Japan, Tokyo, Japan) and subsequently purified using the RNeasy Mini Kit (Qiagen, Tokyo, Japan). The quality and quantity of the total RNA were evaluated on an Agilent 2100 Bioanalyzer (Agilent Technologies Japan, Tokyo, Japan). The total RNA integrity number exceeded 7. Total RNA samples from these animals were subjected to DNA microarray analysis as previously described.Citation21) The Affymetrix Porcine Genome ArrayTM (Affymetrix, Santa Clara, CA) was used, and fluorescence signals were scanned with the Affymetrix GeneChip System. Affymetrix GeneChip Command Console software was used to reduce the array images to the intensity values for each probe (CEL files).
DNA microarray data analysis
Using the R software (ver. 2.7.1; The R Project, http://www.r-project.org), the CEL files were quantified according to the distribution free weighted method (DFW).Citation22) A statistical comparison of HFCD and HFCD + eLF groups was performed with the Rank Products and Benjamini and Hochberg false discovery rate (FDR) corrections.Citation23) The Porcine Genome Array annotation file was downloaded from the Affymetrix website [Porcine Annotations, CSV format, Release 33 (06/22/12)]. The selected probe sets were classified according to gene ontology (GO) and the Kyoto Encyclopedia of Genes and Genomes (KEGG) pathways using the functional annotation tool of the Database for Annotation, Visualization, and Integrated Discovery (DAVID).Citation24) The GO analysis was performed on the basis of the biological process in GOTERM_BP_ALL. The related statistical analysis was performed using Fisher’s exact test and FDR corrections. A significant difference was defined as an FDR-corrected P-value < 0.05.
Statistical analysis
The measured values have all been presented as means with standard deviations. The data between HFCD and HFCD + eLF group were compared using Welch’s test by assuming that the data obtained in this experiment were normally distributed as well as those in many cholesterol-administered animal experiments. Statistical significance was set at a P-value < 0.05. Data were analyzed using JMP version 11. 2. 0 (SAS Institute Inc., Cary, NC, USA).
Results
Effect of eLF on HFCD-induced hypercholesterolemia and atherosclerosis
The growth curve in HFCD + eLF group was slightly lower than that in HFCD group (Fig. (A)). However, the difference was not statistically significant at week 8. Diet intake was also not significantly different at any time point (Fig. (B)). There were no organs whose weights were significantly different between HFCD and HFCD + eLF group (Table ). The hepatic TG and cholesterol levels also did not exhibit the significant difference between HFCD and HFCD + eLF groups. However, eLF treatment tended to reduce HFCD-induced cholesterol levels (35% decrease). Serum total, LDL, and HDL cholesterol levels in HFCD group but not TG levels were remarkably increased and reached plateau at week 2 (Fig. (A)–(D)). eLF treatment exhibited the decrease of total (52%) and LDL cholesterol levels (42%) at week 2. These effects were observed during an experimental period. Because serum total, LDL, and HDL cholesterol levels reached plateau at week 2, statistical analysis for the hypercholesterolemia preventive efficacy of eLF was conducted at week 2 (Table ). eLF treatment significantly decreased the serum total and LDL cholesterol levels (p < 0.05). Serum total and LDL cholesterol levels were positively correlated with hepatic cholesterol levels, respectively (Fig. (A) and (B)). During the necropsy, no atherosclerotic lesions were observed in the right coronary artery (RCA) from the Control group (Fig. (A) and (B)). The arteries from the HFCD group exhibited intimal thickening with foam cell infiltration (Fig. (C) and (D)). Immunohistochemistry also confirmed the presence of macrophage-derived ionized calcium-binding adaptor molecule 1-positive cells and α-smooth muscle actin-positive cells in the intima of RCA (Fig. (A) and (B)). In the HFCD + eLF group (Animal No. 8, Table ), no atherosclerotic lesions were observed except for very slight focal regions (Fig. (E) and (F)). The type of RCA atherosclerosis was classified according to the Stary Type classification (Table ). The atherosclerosis type in the Control group could not be classified because no lesions had developed. All three MMPigs from the HFCD group were classified as having atherosclerosis Type II. In the HFCD + eLF group, 1 MMPig was classified as Type I and the other 2 MMPigs could not be classified. The classifications of the other 14 arteries revealed the remarkable inhibitory effect of eLF in terms of atherosclerosis development (Table ).
Fig. 1. Changes of body weight and diet intake during an 8-week period.
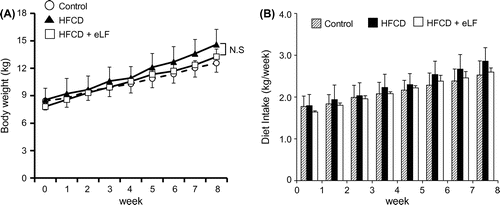
Table 1. Organs weight and hepatic lipid concentration at week 8.
Fig. 2. Changes in the serum lipid concentrations during the 8-week period.
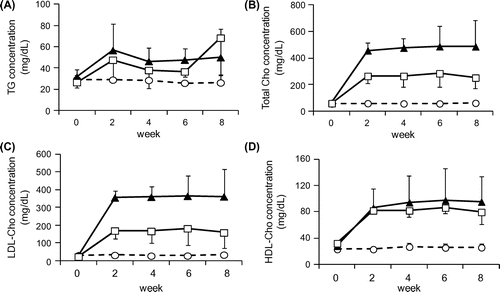
Table 2. Effect of eLF on the serum lipid concentration at week 2.
Fig. 3. Correlation between the serum cholesterol and hepatic cholesterol levels at week 8. Control group (○), HFCD group (▲), and HFCD + eLF group (□).
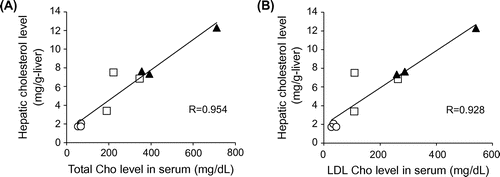
Fig. 4. Histological analysis of the right coronary artery at week 8.
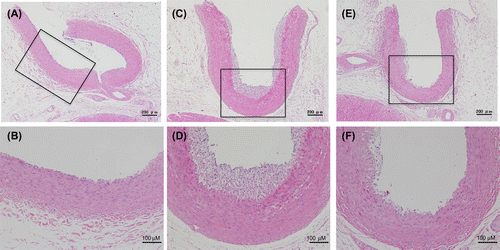
Fig. 5. Immunohistochemistry of atherosclerotic lesions in the high-fat and high-cholesterol diet (HFCD) group. The right coronary artery was analyzed via immunohistochemistry with primary antibodies against the ionized calcium-binding adaptor molecule 1 (Iba-1) and a-smooth muscle actin (α-SMA) and Envision Kit. The sections exhibit positive (A) Iba-1 and (B) α-SMA expression. All bars indicate 100 μm.
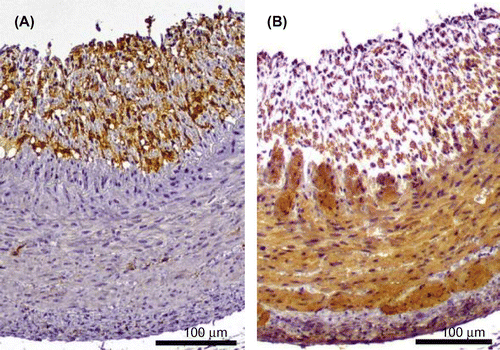
Table 3. Atherosclerotic scores of 15 arteries according to the Stary type classification.
Effect of eLF on hepatic gene expression profiles
The statistical comparison of the HFCD and HFCD + eLF group data according to the RankProducts method with multiple testing corrections using the Benjamini and Hochberg FDR (p < 0.05) resulted in the selection of 444 probes (data not shown). In these probe sets, LDLR and HMGCR were both found to be upregulated (Table ) and the SREBP-2, a key transcriptional factor for these genes, also displayed the tendency toward upregulation (Ssc_16976.1.S1_at, p = 0.055; data not shown). Subsequently, we used the online DAVID software module to identify the overrepresented GO terms and metabolic pathways in the selected genes. A GO analysis of the selected 444 probes demonstrated that the effects of eLF administration indicated significant involvement in the oxidation reduction (GO: 005514) biological process (Supplemental Table S1, S2). A KEGG pathway analysis revealed that seven significantly affected pathways (Table ). The affected genes in steroid biosynthesis (ssc00100) category were CYP family 51, subfamily A, polypeptide 1 (CYP51), NAD(P)-dependent steroid dehydrogenase-like (NSDHL), squalene epoxidase (SQLE), and methylsterol monooxygenase 1 (MSMO1), all of which encode enzymes that promote cholesterol synthesis (Table ). All these genes were upregulated in the HFCD + eLF group compared with the HFCD group.
Table 4. Significantly affected cholesterol metabolism-related gene expression.
Table 5. Significantly affected pathways.
Table 6. Significantly affected steroid biosynthesis genes.
Discussion
In this study, we proved that eLF administration could attenuate the development of HFCD-induced hypercholesterolemia and atherosclerosis in MMPigs (Tables and and Fig. ). Although the effects of LF on lipid metabolism have been reported in clinical trials and animal studies,Citation8,13,25–27) no previous reports clarified the beneficial effects of LF with respect to hypercholesterolemia and atherosclerosis in MMPigs, which are very similar to humans in various aspects such as the regulation of cholesterol homeostasis.
In mammals, cholesterol homeostasis is primarily controlled in the liver, which regulates the blood cholesterol concentration and cholesterol synthesis–catabolism balance. Therefore, in this study, the hepatic gene expression profiles were analyzed via DNA microarray to clarify the effects of eLF on hepatic function. Recently, the RankProducts method with a DFW preprocessing algorithm was reported to be among the best analytical combinations for the accurate detection of differentially expressed genes.Citation22) Therefore, we applied DFW as a quantification method for our DNA microarray data. In this experiment, eLF administration increased the expression of genes related to the cholesterol metabolism (LDLR and HMGCR) and cholesterol synthesis-promoting enzymes (CYP51, MSMO1, NSDHL, and SQLE) (Tables –).Citation28–31) We confirmed that the expression levels of these genes were significantly downregulated in the HFCD group relative to the Control group (data not shown). These results suggest that eLF restores the cholesterol metabolism and synthesis inhibited by HFCD intake. Furthermore, eLF administration exhibited 35% decrease of hepatic cholesterol levels (Table ), and serum total and LDL cholesterol levels were strongly correlated with hepatic cholesterol levels, respectively (Fig. (A) and (B)). Combined with these results, it is suggested that eLF protected the homeostasis from HFCD-induced cholesterol metabolism dysregulation in liver through the decrease of serum cholesterol levels. As one of the action mechanisms, eLF would inhibit cholesterol absorption from the small intestine. Because LF is a cationic protein (isoelectric point: 8.2–8.9)Citation32) and bile acids are anionic substances, LF may interact with bile acids to decrease the micellar solubility of cholesterol. The cationic resin cholestyramine promotes fecal cholesterol excretionCitation33) and has been approved for use as a lipid-lowering agent in several countries. Furthermore, β-conglycinin, a representative cationic protein from soybeans, was shown to promote reduced total blood cholesterol levels in mice and rats,Citation34,35) and β-conglycinin-derived peptides were found to interact with bile acids.Citation8) Takeuchi et al.Citation36) reported that LF supplementation did not promote the bile acid and cholesterol excretion in fecals in mice fed a HFCD. However, unlike our experiment, mice were administered normal LF but not eLF, and high concentration of bile acid (0.25%) was added to the diet. Because experimental conditions are quite different between 2 experiments, the effect of LF on cholesterol and bile acid excretion is still unclear. Therefore, further studies are needed to validate this hypothesis and to clarify the inhibitory potential of LF with respect to cholesterol absorption.
The prevention of hypercholesterolemia is one of the major mechanisms of the inhibition of the atherosclerosis. We hereby propose additional possible action mechanism of eLF in a circulation. Reportedly, endothelial cell injury causes the infiltration of smooth muscle cells and macrophages into the intima in the early stage of atherosclerosis.Citation37) As the next step, macrophages have been reported to take up oxidized LDL deposits from the blood vessel walls via scavenger receptors and to subsequently develop into foam cells.Citation38,39) Reportedly, bovine LF (1 μg/mL) promotes the tube formation and cell proliferation of human aortic endothelial cells.Citation40) Kajikawa et al. reported that bovine LF (0.2–1 mg/mL) remarkably inhibited scavenger receptor-mediated cholesteryl ester accumulation in macrophages.Citation41) Fischer et al.Citation42) reported that administered bovine LF by intragastric intubation to mice could be detected in the plasma as an intact form. Therefore, inhibition of endothelial cell dysfunction and foam cell development from macrophages may be an antiatherogenic effect of eLF. By contrast, in this study, eLF was administered to MMPigs during study periods in the dosage of 37–65 mg/kg/body same range as the Fischer’s report (about 35 mg/kg/body), in which the maximum detected LF was about 50–60 ng/mL in serum.Citation14) Therefore, it is an important issue to clarify whether LF of lower concentration exhibits the inhibitory effect on endothelial cell injury and cholesteryl ester accumulation in macrophages or not. In this study, we demonstrated that eLF exerted inhibitory effects on hypercholesterolemia and atherosclerosis development in MMPigs. Together with these results and the existing food safety profile of LF, eLF appears to be a promising food additive for the prevention of hypercholesterolemia and atherosclerosis.
Authors contributions
H. Kawaguchi, T. Ono, M. Murakoshi, K. Sugiyama, A. Tanimoto, and H. Nishino conceived and designed the experiments. S. Morishita, H. Kawaguchi, T. Ono, and A. Tanimoto performed the experiments. S. Morishita, H. Kawaguchi, T. Ono, and N. Miura analyzed the data. H. Kato contributed DNA microarray reagents/materials/analysis tools. S. Morishita, H. Kawaguchi, T. Ono, M. Murakoshi, and A. Tanimoto wrote the manuscript. All authors reviewed and approved the final manuscript.
Disclosure statement
No potential conflict of interest was reported by the authors.
Funding
This work was supported by Health Labour Sciences Research Grant from the Ministry of Health, Labour and Welfare of Japan [grant number 33361105]; Adaptable Seamless Technology Transfer Program from the Ministry of Education, Culture, Sports, Science and Technology of Japan [A-Step No. AS2316907E]; the Suzuken Memorial Foundation. Authors have no other conflict of interests associated with this study.
Supplemental material
The supplemental material for this paper is available at http://dx.doi.org/10.1080/09168451.2015.1091713.
Supplemental_Table_S2.docx
Download MS Word (16 KB)Supplemental_Table_S1.docx
Download MS Word (15.7 KB)Acknowledgments
The authors thank Prof. Yuji Nakai (Institute for Food Sciences, Hirosaki University) and Mr. Kenji Saito (Organization for Interdisciplinary Research Projects, The University of Tokyo) for their technical advice regarding the microarray analysis.
References
- Dekker JM, Girman C, Rhodes T, et al. Metabolic syndrome and 10-year cardiovascular disease risk in the Hoorn Study. Circulation. 2005;112:666–673.10.1161/CIRCULATIONAHA.104.516948
- Hunt KJ, Resendez RG, Williams K, et al. National Cholesterol Education Program versus World Health Organization metabolic syndrome in relation to all-cause and cardiovascular mortality in the San Antonio Heart Study. Circulation. 2004;110:1251–1257.10.1161/01.CIR.0000140762.04598.F9
- Tomita M, Bellamy W, Takase M, et al. Potent antibacterial peptides generated by pepsin digestion of bovine lactoferrin. J. Dairy Sci. 1991;74:4137–4142.10.3168/jds.S0022-0302(91)78608-6
- Harmsen MC, Swart PJ, de Bethune MP, et al. Antiviral effects of plasma and milk proteins: lactoferrin shows potent activity against both human immunodeficiency virus and human cytomegalovirus replication in vitro. J. Infect. Dis. 1995;172:380–388.10.1093/infdis/172.2.380
- Zimecki M, Wlaszczyk A, Cheneau P, et al. Immunoregulatory effects of a nutritional preparation containing bovine lactoferrin taken orally by healthy individuals. Arch. Immunol. Ther. Exp. (Warsz). 1998;46:231–240.
- Shoji H, Oguchi S, Shinohara K, et al. Effects of iron-unsaturated human lactoferrin on hydrogen peroxide-induced oxidative damage in intestinal epithelial cells. Pediatr. Res. 2007;61:89–92.10.1203/01.pdr.0000250198.22735.20
- Kozu T, Iinuma G, Ohashi Y, et al. Effect of orally administered bovine lactoferrin on the growth of adenomatous colorectal polyps in a randomized, placebo-controlled clinical trial. Cancer Prev. Res. (Phila). 2009;2:975–983.10.1158/1940-6207.CAPR-08-0208
- Ono T, Murakoshi M, Suzuki N, et al. Potent anti-obesity effect of enteric-coated lactoferrin: decrease in visceral fat accumulation in Japanese men and women with abdominal obesity after 8-week administration of enteric-coated lactoferrin tablets. Br. J. Nutr. 2010;104:1688–1695.10.1017/S0007114510002734
- Ono T, Morishita S, Fujisaki C, et al. Effects of pepsin and trypsin on the anti-adipogenic action of lactoferrin against pre-adipocytes derived from rat mesenteric fat. Br. J. Nutr. 2011;105:200–211.10.1017/S0007114510003259
- Ono T, Fujisaki C, Ishihara Y, et al. Potent lipolytic activity of lactoferrin in mature adipocytes. Biosci. Biotechnol. Biochem. 2013;77:566–571.10.1271/bbb.120817
- Moreno-Navarrete JM, Ortega FJ, Ricart W, et al. Lactoferrin increases (172Thr)AMPK phosphorylation and insulin-induced (p473Ser)AKT while impairing adipocyte differentiation. Int. J. Obes. (Lond). 2009;33:991–1000.10.1038/ijo.2009.143
- Yagi M, Suzuki N, Takayama T, et al. Lactoferrin suppress the adipogenic differentiation of MC3T3-G2/PA6 cells. J. Oral Sci. 2008;50:419–425.10.2334/josnusd.50.419
- Takeuchi T, Shimizu H, Ando K, et al. Bovine lactoferrin reduces plasma triacylglycerol and NEFA accompanied by decreased hepatic cholesterol and triacylglycerol contents in rodents. Br. J. Nutr. 2004;91:533–538.10.1079/BJN20041090
- Breslow JL. Mouse models of atherosclerosis. Science. 1996;272:685–688.10.1126/science.272.5262.685
- Forster R, Ancian P, Fredholm M, et al. The minipig as a platform for new technologies in toxicology. J. Pharmacol. Toxicol. Methods. 2010;62:227–235.10.1016/j.vascn.2010.05.007
- Kawaguchi H, Miyoshi N, Miura N, et al. Microminipig, a non-rodent experimental animal optimized for life science research: novel atherosclerosis model induced by high fat and cholesterol diet. J. Pharmacol. Sci. 2011;115:115–121.10.1254/jphs.10R17FM
- Akioka K, Kawaguchi H, Kitajima S, et al. Investigation of necessity of sodium cholate and minimal required amount of cholesterol for dietary induction of atherosclerosis in microminipigs. In Vivo. 2014;28:81–90.
- Miyoshi N, Horiuchi M, Inokuchi Y, et al. Novel microminipig model of atherosclerosis by high fat and high cholesterol diet, established in Japan. In Vivo. 2010;24:671–680.
- Kawaguchi H, Yamada T, Miura N, et al. Rapid development of atherosclerosis in the world’s smallest microminipig fed a high-fat/high-cholesterol diet. J. Atheroscler. Thromb. 2014;21:186–203.10.5551/jat.21246
- Stary HC. Natural history and histological classification of atherosclerotic lesions: an update. Arterioscler. Thromb. Vasc. Biol. 2000;20:1177–1178.10.1161/01.ATV.20.5.1177
- Saito K, Ohta Y, Sami M, et al. Effect of mild restriction of food intake on gene expression profile in the liver of young rats: reference data for in vivo nutrigenomics study. Br. J. Nutr. 2010;104:941–950.10.1017/S0007114510001625
- Chen Z, McGee M, Liu Q, et al. A distribution free summarization method for Affymetrix GeneChip arrays. Bioinformatics. 2007;23:321–327.10.1093/bioinformatics/btl609
- Kadota K, Nakai Y, Shimizu K. Ranking differentially expressed genes from Affymetrix gene expression data: methods with reproducibility, sensitivity, and specificity. Algorithms Mol. Biol. 2009;4:7. Available from: http://www.almob.org/10.1186/1748-7188-4-7
- Huang DW, Sherman BT, Lempicki RA. Systematic and integrative analysis of large gene lists using DAVID bioinformatics resources. Nat. Protoc. 2009;4:44–57.
- Shi J, Finckenberg P, Martonen E, et al. Metabolic effects of lactoferrin during energy restriction and weight regain in diet-induced obese mice. J. Funct. Foods. 2012;4:66–78.10.1016/j.jff.2011.08.001
- Morishita S, Ono T, Fujisaki C, et al. Bovine lactoferrin reduces visceral fat and liver triglycerides in ICR mice. J. Oleo. Sci. 2013;62:97–103.10.5650/jos.62.97
- Kawashima M, Kawakita T, Inaba T, et al. Dietary lactoferrin alleviates age-related lacrimal gland dysfunction in mice. PLoS ONE. 2012;7:e33148.10.1371/journal.pone.0033148
- Tuck SF, Patel H, Safi E, et al. Lanosterol 14 alpha-demethylase (P45014DM): effects of P45014DM inhibitors on sterol biosynthesis downstream of lanosterol. J. Lipid Res. 1991;32:893–902.
- Li L, Kaplan J. Characterization of yeast methyl sterol oxidase (ERG25) and identification of a human homologue. J. Biol. Chem. 1996;271:16927–16933.
- Ohashi M, Mizushima N, Kabeya Y, et al. Localization of mammalian NAD(P)H steroid dehydrogenase-like protein on lipid droplets. J. Biol. Chem. 2003;278:36819–36829.10.1074/jbc.M301408200
- Hidaka Y, Hotta H, Nagata Y, et al. Effect of a novel squalene epoxidase inhibitor, NB-598, on the regulation of cholesterol metabolism in Hep G2 cells. J. Biol. Chem. 1991;266:13171–13177.
- Shimazaki K, Kawaguchi A, Sato T, et al. Analysis of human and bovine milk lactoferrins by rotofor and chromatofocusing. Int. J. Biochem. 1993;25:1653–1658.10.1016/0020-711X(93)90524-I
- Hassan AS, Rampone AJ. Intestinal absorption and lymphatic transport of cholesterol and beta-sitostanol in the rat. J. Lipid Res. 1979;20:646–653.
- Adams MR, Golden DL, Franke AA, et al. Dietary soy beta-conglycinin (7S globulin) inhibits atherosclerosis in mice. J. Nutr. 2004;134:511–516.
- Ferreira Ede S, Silva MA, Demonte A, et al. Soy β-conglycinin (7S globulin) reduces plasma and liver cholesterol in rats fed hypercholesterolemic diet. J. Med. Food. 2011; 14: 94–100.10.1089/jmf.2009.0204
- Nagaoka S, Nakamura A, Shibata H, et al. Soystatin (VAWWMY), a novel bile acid-binding peptide, decreased micellar solubility and inhibited cholesterol absorption in rats. Biosci. Biotechnol. Biochem. 2010;74:1738–1741.10.1271/bbb.100338
- Ross R. The pathogenesis of atherosclerosis: a perspective for the 1990s. Nature. 1993;362:801–809.10.1038/362801a0
- Hazell LJ, Stocker R. Oxidation of low-density lipoprotein with hypochlorite causes transformation of the lipoprotein into a high-uptake form for macrophages. Biochem. J. 1993;290:165–172.10.1042/bj2900165
- Jialal I, Devaraj S. The role of oxidized low density lipoprotein in atherogenesis. J. Nutr. 1996;126:1053s–1057s.
- Ikeda Y, Tajima S, Izawa-Ishizawa Y, et al. Bovine milk–derived lactoferrin exerts proangiogenic effects in an Src-Akt-eNOS–dependent manner in response to ischemia. J. Cardiovasc. Pharmacol. 2013;61:423–429.10.1097/FJC.0b013e318287d526
- Kajikawa M, Ohta T, Takase M, et al. Lactoferrin inhibits cholesterol accumulation in macrophages mediated by acetylated or oxidized low-density lipoproteins. Biochim. Biophys. Acta. 1994;1213:82–90.10.1016/0005-2760(94)90225-9
- Fischer R, Debbabi H, Blais A, et al. Uptake of ingested bovine lactoferrin and its accumulation in adult mouse tissues. Int. Immunopharmacol. 2007;7:1387–1393.10.1016/j.intimp.2007.05.019