Abstract
Matrix metalloproteinases (MMPs) and their endogenous inhibitors (TIMPs) need to be finely modulated in physiological processes. However, oxygen tension influences MMP/TIMP balances, potentially leading to pathology. Intriguingly, new 2H,3H-decafluoropentane-based oxygen-loaded nanodroplets (OLNDs) have proven effective in abrogating hypoxia-dependent dysregulation of MMP and TIMP secretion by single cell populations. This work explored the effects of different oxygen tensions and dextran-shelled OLNDs on MMP/TIMP production in an organized and multicellular tissue (term human placenta). Chorionic villous explants from normal third-trimester pregnancies were incubated with/without OLNDs in 3 or 20% O2. Explants cultured at higher oxygen tension released constitutive proMMP-2, proMMP-9, TIMP-1, and TIMP-2. Hypoxia significantly altered MMP-2/TIMP-2 and MMP-9/TIMP-1 ratios enhancing TIMP-2 and reducing proMMP-2, proMMP-9, and TIMP-1 levels. Intriguingly, OLNDs effectively counteracted the effects of low oxygen tension. Collectively, these data support OLND potential as innovative, nonconventional, and cost-effective tools to counteract hypoxia-dependent dysregulation of MMP/TIMP balances in human tissues.
Graphical abstract
Oxygen-loaded nanodroplets counteract hypoxia-induced dysregulation of balances between gelatinases and inhibitors in human placental explants.
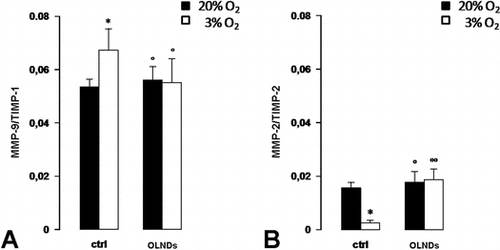
Matrix metalloproteinases (MMPs) are a family of zinc-dependent proteolytic enzymes secreted as latent zymogens activated locally by other proteases and inhibited by their secreted endogenous inhibitors [tissue inhibitor of metalloproteinases (TIMPs)].Citation1) MMPs can collectively process all the components of the basement membrane and the extracellular matrix.Citation2) In addition, MMPs can cleave other molecules, including cytokines, chemokines, growth factors, enzymes, and membrane-bound proteins, thus promoting their activation, inhibition, degradation, or shedding.Citation3) Due to the large kaleidoscope of MMP substrates, these enzymes play pivotal roles along with their inhibitors both in physiology (normal development, wound healing) and pathology (skeletal dysplasiasis, cancer, arthritis, atherosclerosis, fibrosis, emphysema, placental disorders, and neuroinflammation).Citation2,4,5)
The regulation of MMP activity is highly complex and is established at five different levels.Citation6) Gene transcription is induced or repressed by a large variety of soluble factors, including cytokines, growth factors, and hormones as well as by cellular contacts acting through specific signaling pathways. The regulation of protein secretion occurs in cells storing MMP latent forms in granules. After secretion, MMP pro-forms must be activated through proteolysis. Enzymatic activity is further regulated by inhibitors as well as by other mechanisms, such as fine-tuning and stabilization by glycosylation.Citation6)
A unique characteristic of gelatinases (MMP-2 and MMP-9) is the ability of their zymogens to form tight noncovalent and stable complexes with TIMPs. Binding of TIMP-1 and TIMP-2 to MMP-9 and MMP-2 is mediated by two distinct domains of the inhibitors and two domains of the metalloproteinases. The N-terminal domains of TIMPs bind to the enzymes within the active site domains of the enzymes (low affinity sites), whereas the C-terminal domains of TIMPs bind to the C-terminal regions of gelatinases (high affinity sites). Notably, the biphasic binding is consistent with a 1:1 stoichiometry of gelatinase-TIMP complexes.Citation7–9) Interestingly, Olson and colleaguesCitation10) have shown that TIMP-1 binds to the latent (92 kDa) and active (82 kDa) forms of MMP-9 and to the active (62 kDa) form of MMP-2. On the contrary, it fails to interact with the latent (72 kDa) form of MMP-2. The Ki values of TIMP-1 for proMMP-9, MMP-9, and MMP-2 are 35.0, 23.9, and 28.6 nM for the high affinity site and 7.4, 3.1, and 8.5 μM for the low affinity site, respectively. Similarly, TIMP-2 binds to the latent and active forms of MMP-2 and to the active form of MMP-9, but fails to interact with MMP-9 latent form. The Ki values of TIMP-2 for proMMP-2, MMP-2, and MMP-9 are 5.2, 23.1, and 57.9 nM for the high affinity site and 0.2, 2.7, and 12.7 μM for the low affinity site, respectively. Tissue oxygenation plays an important role in modulating MMP/TIMP homeostasis through transcriptional and post-translational mechanisms.Citation11,12) However, the effects of oxygen tension on MMP and TIMP secretion are highly variable depending on the considered cell type, as demonstrated in a series of recent studies from our group.Citation13–15) In particular, hypoxia has been shown: (i) to reduce MMP-9 and increase TIMP-1 without affecting TIMP-2 secretion by primary human monocytes isolated from peripheral bloodCitation13); (ii) to reduce MMP-2, MMP-9, and TIMP-2, without affecting TIMP-1 release from HaCaT keratinocytic cell lineCitation14); and (iii) to enhance MMP-2 and reduce TIMP-1 secretion without affecting TIMP-2 levels in HMEC-1 dermal endothelial cell line.Citation15) These findings lead to two important considerations. On the one hand, caution should be taken when extrapolating data from different cellular models to elaborate general theories on the mechanisms underlying MMP regulation by oxygen tension. On the other hand, although hypoxia appears to impair MMP activity in a cell-specific manner, thus supporting the rationale for future development of personalized therapies, further research on broader therapeutic approaches may not be fully cut out, provided that cell and tissue phenotypes instead of intracellular pathways are targeted.
In this context, intensive research has been performed in the last years to develop new approaches to face hypoxia by delivering exogenous oxygen. Intriguingly, several innovative and nonconventional systems for oxygen release to hypoxic tissues are currently under investigation, including hemoglobin- and perfluorocarbon-based oxygen carriers.Citation16) Among the latter, perfluoropentane-based oxygen-loaded nanobubbles (OLNBs) and 2H,3H-decafluoropentane (DFP)-based oxygen-loaded nanodroplets (OLNDs), both coated with biocompatible polysaccharides such as dextran or chitosan, were recently developed by our group, proving effective in oxygen delivery in vitro and in vivo, either in the absence or presence of complementary ultrasound administration.Citation17–20)
Intriguingly, OLNDs were recently shown to effectively abrogate the effects of hypoxia on MMP and TIMP secretion by human monocytes, keratinocytes, and endothelial cells, independently from the above-mentioned differences in cell type-related dysregulation.Citation13–15) Based on this evidence, the present work aimed at moving on from single cell populations to an organized and multicellular tissue. Notably, throughout pregnancy both oxygen levels and MMP/TIMP balances need to be finely modulated within the human placenta, since altered placental levels of MMPs and tissue inhibitors of metalloproteinase TIMPs compromise efficient utero-placental circulation.Citation21,22) For this reason, term human placental chorionic villous explants were chosen here as a representative in vitro tissue model. Therefore, the effects of different oxygen tensions on the secretion of gelatinases (MMP-2 and MMP-9) and their inhibitors (TIMP-2 and TIMP-1) were firstly studied. Then, the efficacy of newly developed dextran-shelled OLNDs in counteracting the effects of low oxygen tension was evaluated.
Materials and methods
Materials
All materials were from Sigma-Aldrich (St Louis, MO), apart from those listed as follows: ethanol (96%) was from Carlo Erba (Milan, Italy); Epikuron 200® (soya phosphatidylcholine 95%) was from Degussa (Hamburg, Germany); palmitic acid, DFP, perfluoropentane, dextran sodium salt (MW = 100.000), and polyvinylpyrrolidone (PVP) were from Fluka (Buchs, Switzerland); ultrapure water was obtained using a 1-800 Millipore system (Molsheim, France); Ultra-Turrax SG215 homogenizer was from IKA (Staufen, Germany); Philips CM10 instrument was from Philips (Eindhoven, The Netherlands); Formvar-coated copper grid was from Polysciences Europe GmbH (Eppelheim, Germany); XDS-3FL microscope was from Optika (Ponteranica, Italy); Delsa Nano C analyzer was from Beckman Coulter (Brea, CA); polarizing microscope was from Spencer Lens Company (Buffalo, NY); Discovery HR1 Hybrid Rheometer was from TA instruments (Milan, Italy); cell culture Ham’s F12 and RPMI 1640 media were from Invitrogen (Carlsbad, CA); PEN-STREP was from Cambrex Bio Science (Vervies, Belgium); hMMP-2 ELISA kit was from Abnova (Taipei, Taiwan); hMMP-9, hTIMP-1, and hTIMP-2 ELISA kits were from RayBiotech (Norcross, GA); Synergy HT microplate reader was from Bio-Tek Instruments (Winooski, VT); Geldoc densitometer was from Biorad (Hercules, CA).
Preparation and sterilization of OLND solutions
Dextran-shelled OLNDs were prepared as previously described.Citation20) Briefly, 1.5 mL DFP along with 0.5 mL PVP and 1.8 mL Epikuron® 200 solved in 1% w/v ethanol and 0.3% w/v palmitic acid solution were homogenized in 30 mL phosphate buffered saline (PBS) for 2 min at 24000 rpm by using Ultra-Turrax SG215 homogenizer. Thereafter, the solution was saturated with O2 for 2 min. Finally, 1.5 mL dextran solution was added drop-wise while the mixture was homogenized at 13000 rpm for 2 min. OLNDs were sterilized through UV-C exposure for 20 min. Thereafter, UV-C-treated OLNDs were incubated with cell culture RPMI 1640 medium in a humidified CO2/air-incubator at 37 °C up to 72 h, not displaying any signs of microbial contamination when checked by optical microscopy. Moreover, UV-C-sterilized O2-containing solutions underwent further analyses through O3 measurement and electron paramagnetic resonance spectroscopy, showing no O3 generation and negligible singlet oxygen levels immediately after UV exposure.
Characterization of OLNDs
The morphology of OLNDs was determined by transmitting electron microscopy (TEM) and by optical microscopy. TEM analysis was carried out using a Philips CM10 instrument, whereas optical microscopy was carried out using an XDS-3FL microscope. For TEM analysis, OLND formulations were dropped onto a Formvar-coated copper grid and air-dried before observation. Furthermore, average diameters and shell thickness were elaborated from TEM images. Sizes, polydispersity indexes, and zeta potentials of OLNDs were determined by dynamic light scattering using Delsa Nano C instrument, displaying a (0.6 nm–7 μm) range for measurements of particle size distribution. The polydispersity index indicates the size distribution within a nanodroplet population. For zeta potential determination, formulation samples were placed into an electrophoretic cell, where an electric field of approximately 30 V/cm was applied. Each sample was analyzed at least in triplicate. The electrophoretic mobility was converted into zeta potential using the Smoluchowski equation.Citation23) The refractive indexes of OLND formulations were calculated through a polarizing microscope. The viscosity and the shell shear modulus were determined through Discovery HR1 Hybrid Rheometer. Immediately after preparation, oxygen content of OLNDs was evaluated by adding known amounts of sodium sulfite and measuring generated sodium sulfate, according to the reaction:(1)
The stability of formulations stored at 4, 25, or 37 °C was evaluated over time up to six months by determining morphology, sizes, and zeta potential of OLNDs by optical microscopy and light scattering.
Villous explant cultures
Placental tissues from physiological term pregnancies, selected from the local central placental area, were collected immediately after delivery and processed as described previously.Citation24) Small portions of chorionic villi (35 mg) were placed in 24-well culture dishes, cultured in serum-free Ham’s F12 medium and incubated overnight at 37 °C and 5% CO2 to equilibrate. Thereafter, explants were incubated in fresh medium with/without 10% v/v OLNDs for 8 h either in 3 or 20% O2. Each condition was performed in quadruplicate.
Gelatin zymography
To distinguish whether latent or active forms of gelatinases were secreted, placenta explants were assayed by gelatin zymography as previously described.Citation25) Briefly, 15 μL supernatant/lane were separated on 8% polyacrylamide gels containing 0.1% gelatin under nondenaturing/nonreducing conditions. After washings in 2.5% Triton-X100 and incubation at 37 °C in collagenase buffer (with/without 5 mM EDTA), gels were stained with 0.5% Coomassie blue and destained in water. Additionally, bands were quantified using Geldoc densitometer.
ELISA assay
MMP-2, MMP-9, TIMP-1, and TIMP-2 levels were assayed through ELISA kits according to manufacturer’s instructions. After conversion of MMP and TIMP concentrations from ng/mL to nmol/mL, MMP-9/TIMP-1 and MMP-2/TIMP-2 stoichiometric ratios were also calculated.
Statistical analysis
In accordance with,Citation26) descriptive data are expressed as means ± Standard Deviation, whereas inferential data are shown as means + Standard Error Mean or representative images of three independent experiments with similar results, with conditions performed in quadruplicate. All data were analyzed by one-way Analysis of Variance followed by Tukey’s post hoc test.
Results
Characterization of OLND preparations
Before use, all OLND preparations were characterized for: morphology and shell thickness, by optical and TEM; size, particle size distribution, polydispersity index, and zeta potential, by dynamic light scattering; refractive index by polarizing microscopy; viscosity and shell shear modulus by rheometry; and oxygen content (before and after UV-C sterilization) through a chemical assay. Results were always in line with literature data.Citation20) As shown in Fig. , OLNDs had spherical shapes and 596.35 ± 194.09 nm diameters. Furthermore, OLNDs displayed −25.68 ± 1.00 mV zeta potential, 0.13 polidispersity index, 1.33 refractive index, 1.59188e−3 Pa s viscosity, and 5.43e−2 mPa shear modulus, calculated at 150 s−1 shear rate. OLNDs showed a good oxygen-storing capacity (0.43 ± 0.01 mg/mL of oxygen) either before or after 20-min UV-C sterilization.
Fig. 1. OLND morphology and size distribution.
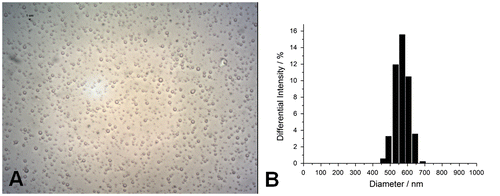
Oxygen tension and OLND effects on gelatinase secretion by human placental explants
Small portions of chorionic villi (35 mg) from physiological term pregnancies were incubated at 37 °C in serum-free Ham’s F12 medium with or without 10% v/v OLNDs for 8 h either in 3 or 20% O2. Thereafter, explant supernatants were collected and underwent analysis by gelatin zymography followed by subsequent densitometry. This technique allows to discern whether gelatinases have been secreted only as latent forms (proMMP-2 and proMMP-9) or they have undergone further proteolysis-mediated activation, thereby appearing as activated enzymes (MMP-2 and MMP-9). As shown in Fig. , placenta explants constitutively released only the latent protein forms of MMP-2 and MMP-9 (proMMP-2 and proMMP-9). Compared to the higher oxygen tension, in hypoxic conditions the release of both proMMP-2 and proMMP-9 appeared to be dramatically reduced. Intriguingly, this effect was effectively counteracted by OLNDs. Based on this evidence, the protein levels of gelatinases were further quantified by ELISA (Fig. ). As an average, the levels of proMMP-2 and proMMP-9 secreted by explants cultured in 20% O2 were ~470 pg/mL and ~4.6 ng/mL, respectively. Low oxygen tension (3% O2) reduced by almost 50% the levels of both molecules. OLNDs fully abrogated the effects of low oxygen tension.
Fig. 2. Oxygen tension and OLND effects on the secretion of latent forms of gelatinases (proMMP-2 and proMMP-9) by human placental explants.
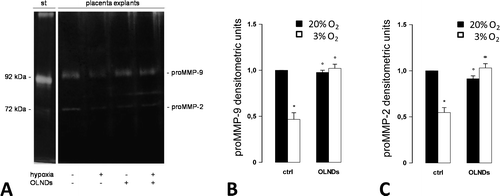
Fig. 3. Oxygen tension and OLND effects on the levels of secreted gelatinases (MMP-2 and -9) in human placental explants.
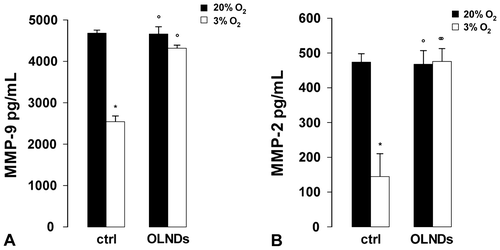
Oxygen tension and OLND effects on TIMP secretion by human placental explants
Small portions of chorionic villi (35 mg) from physiological term pregnancies were incubated at 37 °C in serum-free Ham’s F12 medium with or without 10% v/v OLNDs for 8 h either in 3 or 20% O2. Thereafter, supernatants were collected and TIMP-1 and TIMP-2 levels were quantified by ELISA (Fig. ). As an average, explants cultured in 20% O2 released ~22 ng/mL TIMP-1 and ~9 ng/mL TIMP-2. Low oxygen tension (3% O2) reduced by ~60% TIMP-1 release and almost doubled TIMP-2 levels. OLNDs effectively abrogated the effects of low oxygen tension.
Fig. 4. Oxygen tension and OLND effects on the levels of secreted MMP tissue inhibitors (TIMP-1 and -2) in human placental explants.
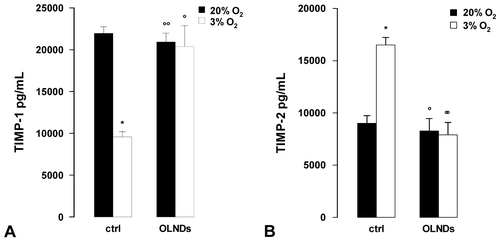
Oxygen tension and OLND effects on MMP/TIMP balances
Based on the values obtained by ELISA for the levels of MMP and TIMP secreted proteins, MMP/TIMP stoichiometric ratios were also calculated (Fig. ). As an average, low oxygen tension (3% O2) enhanced by ~20% the ratio between MMP-9 and TIMP-1, whereas it reduced by ~80% the ratio between MMP-2 and TIMP-2. OLNDs effectively abrogated the effects of low oxygen tension.
Fig. 5. Oxygen tension and OLND effects on secreted MMP/TIMP balances in human placental explants.
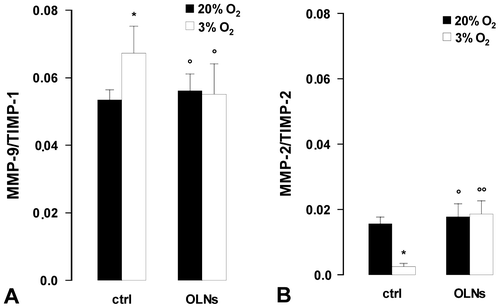
Discussion
The present in vitro study shows that changes in oxygen tension in term human placenta have significant effects on the secretion of gelatinases (MMP-2 and -9) and their inhibitors (TIMP-1 and -2). Furthermore, we show that innovative and nonconventional dextran-shelled/DFP-cored OLNDs counteract the dysregulating effects of low oxygen tension (3% O2) on human placental MMP/TIMP balances.
Throughout pregnancy, the human placenta is exposed to different oxygen tensions, with the first trimester facing lower oxygen levels compared to the third trimester.Citation27) In particular, early in the first trimester, the extravillous trophoblast invades and occludes the terminal portion of spiral arteries, inhibiting maternal blood from entering the intervillous space and resulting in a low oxygen environment (3% O2).Citation28) However, at the end of the first trimester, the endovascular extravillous trophoblast promotes physiological changes in placental spiral arteries, forming funnel-shaped arterioles with increased vascular compliance and circumference and leading to a dramatic increase in oxygen levels (8% O2) for the remainder of pregnancy.Citation28) In the second and third trimester, oxygen becomes more important for normal fetal organogenesis and growth.Citation29) Protection from hypoxia at this stage of development is established through upregulation of several genes, including those encoding for superoxide dismutase, insulin-like growth factor-2, heat shock protein-70, and P-glycoprotein. Citation30–33) Improper or inadequate conversion of spiral arteries in the first trimester can lead to chronic placental ischemia and hypoxia later in gestation.Citation27,28) According to a large number of papers, chronic hypoxia can cause deleterious outcomes such as intrauterine growth restriction, preeclampsia, unexplained miscarriage, and preterm labor,Citation27,28,34,35) although the correlation between hypoxia and preeclampsia has been recently argued by some authors.Citation36) Furthermore, in a recent large-scale study on Plasmodium falciparum- or vivax-infected placentas from Colombian women, hypoxia was also indicated as a marker of disease severity in placental malaria, an often fatal complication of Plasmodium infection.Citation37)
In the present work, villous explants from normal third trimester pregnancies constitutively released MMP-2 and MMP-9 proforms, as well as TIMP-1 and TIMP-2. Compared to our control group (20% O2), low oxygen tension (3% O2) strongly altered gelatinase secretion, reducing both MMP-2 and MMP-9 levels. Interestingly, in HTR-8/SVneo and BeWo trophoblast cells, MMP-2 mRNA expression and proMMP-2 protein levels are reportedly decreased after hypoxia-dependent A2B adenosine receptor activation.Citation38)
Beyond gelatinases, low oxygen tension also altered TIMP levels. TIMP-1 secretion by explants cultured in 3% O2 was significantly lower compared to the control group, whereas TIMP-2 was markedly increased. Consequently, MMP-9/TIMP-1 stoichiometric ratio was enhanced while MMP-2/TIMP-2 was reduced. These data are consistent with evidence showing lower TIMP-1 protein secretion in hypoxic HTR-8/SVneo trophoblast cellsCitation39) and TIMP-2 upregulation in pregnant catechol-o-methyltransferase-deficient mice with hypoxic placentas.Citation40)
As a next step, dextran-shelled/DFP-cored OLND were challenged for their ability to counteract low oxygen-dependent MMP/TIMP dysregulation in placental explants. The major novelty of these nonconventional gas nanocarriers, recently developed by our group, is the oxygen-storing core structure consisting in DFP, a fluorocarbon that makes OLNDs more stable than former perfluoropentane-based OLNBs without compromising their ability to release oxygen.Citation19,20) Extensive toxicity, absorption, distribution, and excretion studies have shown that fluorocarbons such as DFP are extremely stable, biologically inert, and rapidly excreted into the expired air in a nonmetabolized form after injection into the bloodstream.Citation41) On the other hand, dextran has been chosen as the main constituent of the polysaccharidic shell as for former OLNBs,Citation18 since dextran-based formulations have been extensively tested for biocompatibility,Citation42,43 and dextran-based hydrogels are currently used as matrices in tissue engineering, without showing signs of inflammation in vivo.Citation44) Recent toxicological studies on mechanically processed polysaccharides of different molecular weight have shown that dextran, along with the products of its mechano-chemical processing, can be classified as class 4 (low-toxicity) substance.Citation44)
Before use, OLND preparations employed in the present study were characterized for morphology, average diameters, shell thickness, size distribution, polydispersity index, zeta potential, refractive index, viscosity, shell shear modulus, and oxygen content (both before and after UV sterilization). For all the parameters, results were consistent with those previously obtained and recently published.Citation20) All OLND preparations displayed spherical shapes, nanometric sizes, negative charges, as well as similar viscosity and shell shear modulus. OLND negative charge is a likely consequence of the presence of dextran in the outer shell, since it is well known that this neutral polymer acquires negative polarity when immerged in a saline solution (such as PBS here).Citation45) Furthermore, the zeta potential measures charge repulsion or attraction between particles. Therefore, it is also a fundamental parameter to determine nanoparticle physical stability, with zeta potentials lower than −30 mV or larger than + 30 mV being generally required for physical stability of colloid systems.Citation23) Although OLNDs displayed zeta potentials slightly larger than −30 mV, our formulations proved to be physically stable over time for the steric repulsion of the polymer chains, as assessed by monitoring their sizes and zeta potential by dynamic light scattering up to six months after manufacturing. As expected, OLND solutions also displayed a good oxygen-storing capacity (0.4 mg O2/ml) either before or after UV-C sterilization. UV-C exposure was not accompanied by O3 and singlet oxygen generation.
Intriguingly, OLNDs effectively counteracted all the effects of low oxygen tension on gelatinase and TIMP secretion by placental explants, restoring physiological MMP/TIMP balances. These data, obtained upon culturing an organized and multicellular tissue such as the human placenta at different oxygen tensions in the absence or presence of OLNDs, expand and fit well with previous evidence from our group on OLND effects in single cell populations.Citation13–15) Indeed, in a series of recent works, we have shown that OLNDs can fully abrogate hypoxia-dependent dysregulation of MMP and TIMP production by human monocytic,Citation13) epithelial,Citation14) and endothelial cells,Citation15) no matter whether hypoxia previously up- or down-regulated the levels of these molecules, depending on the cell type. These results, combined with the intrinsic benefits of nanodroplets, including size, charge, stability, controlled release, and suitability for further drug functionalization, drug loading, or encapsulationCitation19,20)—not to mention nanodroplet antimicrobial properties when chitosan is employed as a shell polysaccharideCitation46)—strengthen the proposal that OLNDs may potentially serve as medical devices for preventive or adjuvant treatment in tissue disorders characterized by poor oxygenation and altered MMP/TIMP balances, thus encouraging further basic research as well as future preclinical and clinical studies aimed at eventually translating this technology to the clinical practice.
Ethical standards
This study, approved by the Institutional Review Board of O.I.R.M. S. Anna Hospital and “Ordine Mauriziano di Torino” (n.209; protocol 39226/C.27.1 04/08/09), was conducted in accordance with the Declaration of Helsinki; with the EU Directive 2010/63/EU for animal experiments; and with the Uniform Requirements for manuscripts submitted to Biomedical journals. All donors provided written informed consent.
Funding
This work was supported by the Compagnia di San Paolo [grant number ORTO11CE8R]; European Community [grant number CHIC600841]. The funding sources had no role in the project design and development.
Acknowledgments
Thanks are due to Adriano Troia for suggestions on nanodroplet manufacturing, to Giuliana Giribaldi for comments on the manuscript, and to Ghislain Opdenakker and Philippe Van den Steen for kindly giving recombinant human proMMP-9 and MMP-9.
Disclosure statement
Roberta Cavalli, Caterina Guiot, and Mauro Prato have been designed as inventors and the University of Torino as recipient of the international patent no. WO2015/028901 A1 (A nanostructure for the vehiculation of gas and/or active ingredients and/or contrast agents and use thereof) issued under the Patent Copyright Treaty (PCT). Other than that, no potential conflict of interest was reported by the authors.
References
- Nagase H, Visse R, Murphy G. Structure and function of matrix metalloproteinases and TIMPs. Cardiovasc. Res. 2006;69:562–573.10.1016/j.cardiores.2005.12.002
- Vandenbroucke RE, Libert C. Is there new hope for therapeutic matrix metalloproteinase inhibition? Nat. Rev. Drug Discov. 2014 13:904–927.10.1038/nrd4390
- Cauwe B, Van den Steen PE, Opdenakker G. The biochemical, biological, and pathological kaleidoscope of cell surface substrates processed by matrix metalloproteinases. Crit. Rev. Biochem. Mol. Biol. 2007;42:113–185.10.1080/10409230701340019
- Malemud CJ. Matrix metalloproteinases (MMPs) in health and disease: an overview. Front. Biosci. 2006;11:1696–1701.10.2741/1915
- Mandal M, Mandal A, Das S, et al. Clinical implications of matrix metalloproteinases. Mol. Cell. Biochem. 2003;252:305–329.10.1023/A:1025526424637
- Van den Steen PE, Dubois B, Nelissen I, et al. Biochemistry and molecular biology of gelatinase B or matrix metalloproteinase-9 (MMP-9). Crit. Rev. Biochem. Mol. Biol. 2002;37:375–536.10.1080/10409230290771546
- Willenbrock F, Crabbe T, Slocombe PM, et al. The activity of the tissue inhibitors of metalloproteinases is regulated by C-terminal domain interactions: a kinetic analysis of the inhibition of gelatinase A. Biochemistry. 1993;32:4330–4337.10.1021/bi00067a023
- O’Connell JP, Willenbrock F, Docherty AJ, et al. Analysis of the role of the COOH-terminal domain in the activation, proteolytic activity, and tissue inhibitor of metalloproteinase interactions of gelatinase B. J. Biol. Chem. 1994;269:14967–14973.
- Stetler-Stevenson WG, Krutzsch HC, Liotta LA. Tissue inhibitor of metalloproteinase (TIMP-2). A new member of the metalloproteinase inhibitor family. J. Biol. Chem. 1989;264:17374–17378.
- Olson MW, Gervasi DC, Mobashery S, et al. Kinetic analysis of the binding of human matrix metalloproteinase-2 and -9 to tissue inhibitor of metalloproteinase (TIMP)-1 and TIMP-2. J. Biol. Chem. 1997;272:29975–29983.10.1074/jbc.272.47.29975
- Steinke JW, Woodard CR, Borish L. Role of hypoxia in inflammatory upper airway disease. Curr. Opin. Allergy Clin. Immunol. 2008;8:16–20.10.1097/ACI.0b013e3282f3f488
- Kaur B, Khwaja FW, Severson EA, et al. Hypoxia and the hypoxia-inducible-factor pathway in glioma growth and angiogenesis. Neuro. Oncol. 2005;7:134–153.10.1215/S1152851704001115
- Gulino GR, Magnetto C, Khadjavi A, et al. Oxygen-loaded nanodroplets effectively abrogate hypoxia dysregulating effects on secretion of matrix metalloproteinase-9 and tissue inhibitor of metalloproteinase-1 by human monocytes. Mediators Inflamm. 2015;2015:964838.
- Khadjavi A, Magnetto C, Panariti A, et al. Chitosan-shelled oxygen-loaded nanodroplets abrogate hypoxia dysregulation of human keratinocyte gelatinases and inhibitors: new insights for chronic wound healing. Toxicol. Appl. Pharmacol. 2015;286:108–206.
- Basilico N, Magnetto C, D’Alessandro S, et al. Dextran-shelled oxygen-loaded nanodroplets reestablish a normoxia-like pro-angiogenic phenotype and behavior in hypoxic dermal microvascular endothelium. Toxicol. Appl. Pharmacol. 2015;288:330–338. pii: S0041-008X(15)30057-0. doi: 10.1016/j.taap.2015.08.005.
- Cabrales P, Intaglietta M. Blood Substitutes. ASAIO J. 2013;59:337–354.10.1097/MAT.0b013e318291fbaa
- Cavalli R, Bisazza A, Rolfo A, et al. Ultrasound-mediated oxygen delivery from chitosan nanobubbles. Int. J. Pharm. 2009;378:215–217.10.1016/j.ijpharm.2009.05.058
- Cavalli R, Bisazza A, Giustetto P, et al. Preparation and characterization of dextran nanobubbles for oxygen delivery. Int. J. Pharm. 2009;381:160–165.10.1016/j.ijpharm.2009.07.010
- Magnetto C, Prato M, Khadjavi A, et al. Ultrasound-activated decafluoropentane-cored and chitosan-shelled nanodroplets for oxygen delivery to hypoxic cutaneous tissues. RSC Adv. 2014;4:38433–38441.10.1039/C4RA03524K
- Prato M, Magnetto C, Jose J, et al. 2H,3H-decafluoropentane-based nanodroplets: new perspectives for oxygen delivery to hypoxic cutaneous tissues. PLOS One. 2015;10:e0119769.10.1371/journal.pone.0119769
- Kingdom J, Huppertz B, Seaward G, et al. Development of the placental villous tree and its consequences for fetal growth. Eur. J. Obstet. Gynecol. Reprod. Biol. 2000;92:35–43.10.1016/S0301-2115(00)00423-1
- Vu TH, Werb Z. Matrix metalloproteinases: effectors of development and normal physiology. Genes Dev. 2000;14:2123–2133.10.1101/gad.815400
- Sze A, Erickson D, Ren L, et al. Zeta-potential measurement using the Smoluchowski equation and the slope of the current-time relationship in electroosmotic flow. J. Colloid Interface Sci. 2003;261:402–410.10.1016/S0021-9797(03)00142-5
- Rolfo A, Garcia J, Todros T, et al. The double life of MULE in preeclamptic and IUGR placentae. Cell Death Dis. 2012;3:e305.10.1038/cddis.2012.44
- Khadjavi A, Valente E, Giribaldi G, et al. Involvement of p38 MAPK in haemozoin-dependent MMP-9 enhancement in human monocytes. Cell Biochem. Funct. 2014;32:5–15.10.1002/cbf.v32.1
- Cumming G, Fidler F, Vaux DL. Error bars in experimental biology. J. Cell Biol. 2007;177:7–11.10.1083/jcb.200611141
- Murray AJ. Oxygen delivery and fetal-placental growth: beyond a question of supply and demand? Placenta. 2012;33: e16e22.
- Patel J, Landers K, Mortimer RH, et al. Regulation of hypoxia inducible factors (HIF) in hypoxia and normoxia during placental development. Placenta. 2010;31: 951e7.
- Hutter D, Kingdom J, Jaeggi E. Causes and mechanisms of intrauterine hypoxia and its impact on the fetal cardiovascular system: a review. Int. J. Pediatr. 2010;2010:401323.
- Clifton VL, Vanderlelie J, Perkins AV. Increased anti-oxidant enzyme activity and biological oxidation in placentae of pregnancies complicated by maternal asthma. Placenta. 2005;26: 773e9.
- Trollmann R, Klingmuller K, Schild RL, et al. Differential gene expression of somatotrophic and growth factors in response to in vivo hypoxia in human placenta. Am. J. Obstet. Gynecol. 2007;197:601.e1-6.
- Richter HG, Camm EJ, Modi BN, et al. Ascorbate prevents placental oxidative stress and enhances birth weight in hypoxic pregnancy in rats. J. Physiol. 2012;590: 1377e87.
- Javam M, Audette MC, Iqbal M, et al. Effect of oxygen on multidrug resistance in term human placenta. Placenta. 2014;35:324–330.10.1016/j.placenta.2014.02.010
- Soleymanlou N, Jurisica I, Nevo O, et al. Molecular evidence of placental hypoxia in preeclampsia. J. Clin. Endocrinol. Metab. 2005;90:4299–4308.10.1210/jc.2005-0078
- McCarthy C, Cotter FE, McElwaine S, et al. Altered gene expression patterns in intrauterine growth restriction: potential role of hypoxia. Am. J. Obstet. Gynecol. 2007;196:70e1-e6.
- Huppertz B, Weiss G, Moser G. Trophoblast invasion and oxygenation of the placenta: measurements versus presumptions. J. Reprod. Immunol. 2014;101–102:74–79.10.1016/j.jri.2013.04.003
- Agudelo OM, Aristizabal BH, Yanow SK, et al. Submicroscopic infection of placenta by Plasmodium produces Th1/Th2 cytokine imbalance, inflammation and hypoxia in women from north-west Colombia. Malar. J. 2014;13:122.10.1186/1475-2875-13-122
- Darashchonak N, Sarisin A, Kleppa MJ, et al. Activation of adenosine A2B receptor impairs properties of trophoblast cells and involves mitogen-activated protein (MAP) kinase signaling. Placenta. 2014;35:763–771.10.1016/j.placenta.2014.06.369
- Canning MT, Postovit LM, Clarke SH, et al. Oxygen-mediated regulation of gelatinase and tissue inhibitor of metalloproteinases-1 expression by invasive cells. Exp. Cell Res. 2001;267:88–94.10.1006/excr.2001.5243
- Lee SB, Wong AP, Kanasaki K, et al. Preeclampsia. Am. J. Pathol. 2010;176:710–720.10.2353/ajpath.2010.090513
- Castro CI, Briceno JC. Perfluorocarbon-based oxygen carriers: review of products and trials. Artif. Organs. 2010;34:622–634.
- Bos GW, Hennink WE, Brouwer LA, et al. Tissue reactions of in situ formed dextran hydrogels crosslinked by stereocomplex formation after subcutaneous implantation in rats. Biomaterials. 2005;26:3901–3909.10.1016/j.biomaterials.2004.10.008
- De Groot CJ, Van Luyn MJA, Van DijK-Wolthuis WN, et al. In vitro biocompatibility of biodegradable dextran-based hydrogels tested with human fibroblasts. Biomaterials. 2001;22:1197–1203.10.1016/S0142-9612(00)00266-0
- Möller S, Weisser J, Bischoff S, et al. Dextran and hyaluronan methacrylate based hydrogels as matrices for soft tissue reconstruction. Biomol. Engin. 2007;24:496–504.10.1016/j.bioeng.2007.08.014
- Brooks DE, Seaman GVF. The effect of neutral polymers on the electrokinetic of cells and other charged particles. I. Models for the zeta potential increase. J. Colloid Interface Sci. 1972;43:670–686.
- Banche G, Prato M, Magnetto C, et al. Antimicrobial chitosan nanodroplets: new insights for ultrasound-mediated adjuvant treatment of skin infection. Future Microbiol. 2015;10:929–939.10.2217/fmb.15.27