Abstract
A new compound in cucumber, Cucumis sativus, nutrient solution that appears under iron-deficient conditions, but not under ordinary culture conditions, has been revealed by HPLC analysis. The chemical structure of this compound was identified using LC-MS and NMR techniques as that of 4′-ketoriboflavin. This is the first report to show that 4′-ketoriboflavin can be found in metabolites from organisms.
Iron is an essential metal in biological processes such as intracellular respiration, photosynthesis, nitrogen fixation, and DNA synthesis. Under aerobic conditions, however, iron generally exists in an insoluble ferric form. There are two types of iron uptake mechanisms in plants: Strategy I and Strategy II.Citation1−3) Strategy I plants use a Fe(III) reduction-based mechanism,Citation4) whereas Strategy II plants use a Fe(III) chelation-based mechanism.Citation5) In some dicots that use the Strategy I mechanism, flavins are known to be secreted under iron-deficient conditions.Citation6−12) Shinmachi et al. reported that cucumber, Cucumis sativus (C. sativus), produced the highest amounts of flavins under iron-deficient conditions among several tested dicots.Citation13) In an HPLC analysis of C. sativus in an iron-deficient nutrient solution, we found a new peak in its chromatogram that is not seen in ordinary culture. We determined the chemical structure of the peak using LC-MS and NMR techniques.
C. sativus specimens were grown in a growth chamber with a photosynthetic photon flux density of 30 μmol m−2 s−2 with a photoperiod of 16 h light at 26 °C during the day and 8 h dark at 20 °C during the night. Seeds were germinated and grown in vermiculite for five days. Seedlings were then grown for one additional month in HI (hairy root and intact plant) nutrient solution (pH 6.0) as described by Shinmachi et al. Citation14) Plants were transplanted into 100 or 500 ml shading glass bottles. HI nutrient solution strength was increased once each week (natural HI nutrient solution with 1 ppm iron). Control (Fe-sufficient) plants were grown with 5 ppm iron (Fe(III)-EDTA), while Fe-deficient plants were grown with no added iron for 12 days; in both cases the nutrient solution was replaced every 3 days. The Fe-sufficient and -deficient nutrient solutions were pooled and compared. Yellow pigment was only observed in the Fe-deficient nutrient solution. To concentrate this yellow pigment, pooled Fe-deficient nutrient solution (100 ml) was applied to Sep-Pak C18 cartridges (Waters, Milford, MA, USA) that had been pre-equilibrated with methanol and distilled water. The pigments retained in these cartridges were eluted with pure methanol and dried under N2. The prepared pigments were suspended in 5 mM ammonium acetate solution, and the suspension was then placed onto a Pegasil ODS SP100 (Senshu Scientific, Tokyo, Japan) C-18 column (4.6 × 250 mm) equilibrated with 5 mM ammonium acetate: methanol (70: 30) and subjected to HPLC using a chromatograph (L-7000 series, HITACHI, Tokyo, Japan) that was equipped with an online degasser and fluorescent detector (S-3370, Soma, Tokyo, Japan; excitation 450 nm, emission 530 nm). An HPLC chromatogram of the suspension showed two peaks, one corresponding to riboflavin and the other as yet unidentified (compound X). The unidentified compound eluted at a longer retention time compared with authentic flavins (Fig. (A)–(C)). A diode array detector (L-2450, HITACHI) was used to assess the UV–vis spectrum of the unidentified peak at three absorption maxima (approximately 266, 371 and 445 nm); this revealed a spectrum similar to that of authentic flavin (Fig. (D) and (E)). These results suggested that the unidentified peak was a flavin derivative. This flavin derivative was estimated to account for 64% of the secreted flavin compounds based on the fluorescence intensities of riboflavin and the flavin derivative in the chromatogram.
Fig. 1. Flavin compounds present in (A) standard solution [FAD, FMN, and riboflavin (Rbfl)], and in C. sativus nutrient solution cultivated under (B) iron-deficient and (C) iron-sufficient conditions.
![Fig. 1. Flavin compounds present in (A) standard solution [FAD, FMN, and riboflavin (Rbfl)], and in C. sativus nutrient solution cultivated under (B) iron-deficient and (C) iron-sufficient conditions.](/cms/asset/468bcfb3-5e86-4e38-a972-50404505c626/tbbb_a_1095070_f0001_b.gif)
For qualitative analysis of the flavin derivative, two liters of C. sativus nutrient solution were concentrated using a rotary evaporator. The resultant flavin derivative solution was placed on Sep-Pak C18 cartridges (Waters), and the flavin derivative fractionation solution was prepared using the methods described above. The prepared solution was fractionated by means of HPLC using a Pegasil ODS SP100 (Senshu Scientific) C-18 column (10 × 250 mm) equilibrated with 5 mM ammonium acetate: methanol (70: 30). The flow rate was 3.0 min/ml. The flavin derivative appeared at a retention time of 15.97 min. The fractionated flavin derivative was concentrated using a rotary evaporator, and the resultant solution was desalted using Sep-Pak C18 cartridges (Waters). The flavin derivative retained in the cartridges was eluted with pure methanol and dried under N2. Approximately 1 mg of flavin derivative was prepared as a yellow solid. The solids were suspended in 5 mM ammonium acetate solution. The flavin derivative suspended in the solution was analyzed by LC-MS/MS using a system consisting of a quadruple/time-of-flight tandem mass spectrometer (Triple TOD 5600 system, AB SCIEX, Framingham, MA, USA) and an ultra high performance liquid chromatograph (Nexera, Shimadzu, Kyoto, Japan) equipped with a Pegasil ODS SP100 (Senshu Scientific) C-18 column (4.6 × 250 mm). The mobile phase and flow rate were the same as for HPLC analysis. LC-MS/MS data of the flavin derivative are shown in Fig. : m/z 375.1280 [M+H]+ ( requires 375.1299). A comparison of the MS/MS data with those of riboflavin, and specifically our observation of a fragment ion at m/z 243, suggested the presence of a 7,8-dimethylisoalloxazine unit (Fig. ). Based on the measured accurate mass data, the molecular formula was speculated to be C17H18N4O6. These data suggested that this compound was a riboflavin derivative with a dehydrogenated ribityl chain, indicating that the structure of the flavin derivative was a ketoriboflavin. Previously, two ketoriboflavins, 2-ketoriboflavin, and 4-ketoriboflavin have been reported as photodegradation products of riboflavin.Citation15) The structures of these ketoriboflavins were estimated by LC-MS and also by a degradation study using isotope-labeled materials.Citation15,16) However, NMR data of these compounds had not previously been reported. For further structural elucidation of the unidentified ketoriboflavin from C. sativus, NMR spectral analyses including 1H and 13C NMR, heteronuclear single-quantum correlation, heteronuclear multiple bond correlation (HMBC) and nuclear Overhauser effect spectroscopy (NOESY) were performed (Fig. ). Spectroscopic data for the ketoriboflavin were as follows: 1H NMR (600 MHz, CD3OD): δ 2.46 (3H, s, 7-Me), 2.58 (3H, s, 8-Me), 4.44 (1H, d, J = 5.2 Hz, 3′-H), 4.46 (1H, m, 2′-H), 4.55 (1H, d, J = 19.5 Hz, 5′-H), 4.60 (1H, d, J = 19.5 Hz, 5′-H), 4.89 (2H, m, 1′-H), 7.93 (1H, s, 9-H), 7.95 (1H, s, 6-H); and 13C NMR (150 MHz, CD3OD): δ 19.3 (7-Me), 21.3 (8-Me), 49.4 (C-1′), 68.2 (C-5′), 71.7 (C-2′), 78.4 (C-3′), 118.6 (C-9), 132.3 (C-6), 134.1 (C-9a), 136.5 (C-5a), 137.4, (C-4a), 138.6 (C-7), 149.3 (C-8), 152.2 (C-10a), 158.9 (C-2), 162.4 (C-4), 212.7 (C-4′). 1H and 13C NMR spectroscopic data indicated that this compound consisted of a dimethylated isoalloxazine ring and a pentose that included one carbonyl group as expected. The 13C NMR data for dimethylated isoalloxazine ring of the unidentified ketoriboflavin were assigned by HMBC analysis and comparison to the reported data for riboflavin.Citation17) 1H NMR data for the 4′-ketoriboflavin showed that the vicinal coupling constant value between H-2′ and H-3′ of the pentose unit was J = 5.2 Hz. Relative configuration of C-2′ and C-3′ positions was estimated to be eryrthro by comparing the J values to those of d-erythro-2-pentulose (J = 5.4 Hz) and d-threo-2-pentulose (J = 2.4 Hz).Citation18) The observed NOESY correlations of 6-H/7-Me, 9-H/8-Me, and 9-H/1′-H together with the HMBC spectrum revealed the pattern of substitution on the isoalloxazine ring as shown in Fig. . The HMBC spectrum also showed that the specific 5′-H terminal methylene group in the pentosyl side chain was connected with a C-4′ carbonyl carbon (δC 212.7). The presumable precursor for this compound was riboflavin, which had already been identified among C. sativus secretions, so that the stereochemistry of the ribityl sidechain would be retained. Consequently, the structure of this compound was determined to be 4-ketoriboflavin, as shown in Fig. .
Fig. 2. MS/MS spectrum of riboflavin (A) and an unidentified flavin compound (B) in the positive ionization mode.
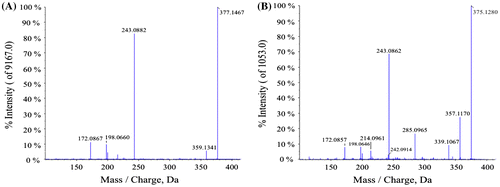
Fig. 3. Structure of riboflavin (A) and 4′-ketoriboflavin (B) as determined using 1H NMR (C), 13C NMR (D), NOESY (E), and HMBC (F).
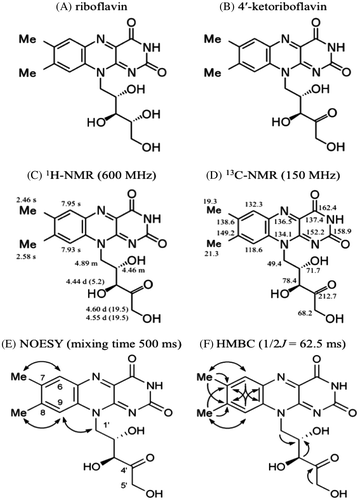
The flavins secreted under iron-deficient conditions were analyzed using the lumi-flavin method.Citation6,19) HPLC analysis of iron-deficient nutrient solution showed that both Beta vulgaris and Spinach secreted Riboflavin 3′- and 5′-sulfate, while Medicago truncatula secreted 7-carboxy-riboflavin, 7-hydroxy-riboflavin and 7α-hydroxy-riboflavin.Citation7,8,12) This is the first report to show that 4′-ketoriboflavin, which has an oxidized ribityl chain, was secreted not only from animals and bacteria, but also from plants.
Only two flavin compounds, riboflavin and 4′-ketoriboflavin, were secreted from C. sativus under iron-deficient conditions. The 4′-ketoriboflavin appears not to be a photodegradation product but rather to be secreted by C. sativus, given that the amounts of secreted 4′-ketoriboflavin were greater than those of riboflavin. We are interested in how the interaction between iron compounds and 4′-ketoriboflavin may differ from that between iron compounds and other flavins, as 4′-ketoriboflavin was only secreted under iron-deficient conditions.
Authors contribution
J. Satoh, E. Yoshimura, S. Kawasaki and Y. Niimura designed the study. J. Satoh, H. Koshino, R. Katsuta and Y. Niimura wrote the manuscript. K. Sekino, K. Takeda, and F. Shinmachi cultivated the plant and performed HPLC. S. Ito performed LC-MS and analyzed the data. H. Koshino performed NMR. H. Koshino, R. Katsuta, and T. Nukada analyzed the NMR data. All authors reviewed and approved the final manuscript.
Acknowledgments
We are grateful to prof. Kyoko HIGUCHI (Tokyo University of Agriculture) and prof. Shunsuke YAJIMA (Tokyo University of Agriculture) for helpful advice in this study. We thank C. Takaishi, S. Yokotani, Y. Anahara, M. Yamanaka, and K. Wada for their technical assistance in preparing 4′-ketoriboflavin for NMR analysis.
Disclosure statement
No potential conflict of interest was reported by the authors.
References
- Römheld V, Marschner H. Mobilization of iron in the rhizosphere of different plant species. Adv. Plant Nutr. 1986;2:155–204.
- Bienfait HF. Mechanisms in Fe-efficiency reactions of higher plants. J. Plant Nutr. 1988;11:605–629.
- Kobayashi T, Nishizawa NK. Iron uptake, translocation, and regulation in higher plants. Annu. Rev. Plant Biol. 2012;63:131–152.
- Brumbarova T, Bauer P, Ivanov R. Molecular mechanisms governing Arabidopsis iron uptake. Trends Plant Sci. 2015;20:124–133.
- Kobayashi T, Nishizawa N, Mori S. Molecular analysis of iron-deficient graminaceous plants. In: Barton L, Abadia J, editors. Iron nutrition in plants and rhizospheric microorganisms. Springer; 2006. Chapter 20; p. 395–435.
- Welkie GW, Miller GW. Iron Nutrition of Nicotiana Tabacum L. in relation to riboflavin, riboflavin-5-phosphate, and flavin adenine dinucleotide content. Plant Physiol. 1960;35:516–520.
- Susin S, Abian J, Sanchez-Baeza F, et al. Riboflavin 3′- and 5′-sulfate, two novel flavins accumulating in the roots of iron-deficient sugar beet (Beta vulgaris). J. Biol. Chem. 1993;268:20958–20965.
- Susín S, Abián J, Peleato ML, et al. Flavin excretion from roots of iron-deficient sugar beet (Beta vulgaris L.). Planta. 1994;193:514–519.
- Welkie GW. Taxonomic distribution of dicotyledonous species capable of root excretion of riboflavin under iron deficiency. J. Plant Nutr. 2000;23:1819–1831.
- Higa A, Mori Y, Kitamura Y. Iron deficiency induces changes in riboflavin secretion and the mitochondrial electron transport chain in hairy roots of Hyoscyamus albus. J. Plant Physiol. 2010;167:870–878.
- Rodríguez-Celma J, Lattanzio G, Grusak MA, et al. Root responses of Medicago truncatula plants grown in two different iron deficiency conditions: changes in root protein profile and riboflavin biosynthesis. J. Proteome Res. 2011;10:2590–2601.
- Rodriguez-Celma J, Vazquez-Reina S, Orduna J, et al. Characterization of flavins in roots of Fe-deficient strategy I plants, with a focus on Medicago truncatula. Plant Cell Physiol. 2011;52:2173–2189.
- Shinmachi F, Hasegawa I, Yazaki J. Difference in riboflavin secretion phenomenon as a result of iron-deficiency among plant species. Jpn J. Soil Sci. Plant Nutr. [in Japanese with English abstract]. 1995;66:337–341.
- Shinmachi F, Hasegawa I, Yazaki J. Analysis of iron-deficiency response systems on the plant root, using the hairy root. Jpn. J. Soil Sci. Plant Nutr. [in Japanese with English abstract]. 1992;63:202–209.
- Cairns WL, Metzler DE. Photochemical degradation of flavins. VI. A new photoproduct and its use in studying the photolytic mechanism. J. Am. Chem. Soc. 1971;93:2772–2777.
- Hardwick CC, Herivel TR, Hernandez SC, et al. Separation, identification and quantification of riboflavin and its photoproducts in blood products using high-performance liquid chromatography with fluorescence detection: a method to support pathogen reduction technology. Photochem. Photobiol. 2004;80:609–615.
- Keller PJ, Le Van Q, Bacher A, et al. Biosynthesis of riboflavin. Tetrahedron. 1983;39:3471–3481.
- Vuorinen T, Serianni AS. Synthesis of d-erythro-2-pentulose and d-threo-2-pentulose and analysis of the 13C- and 1H-n.m.r. spectra of the 1-13C- and 2-13C-substituted sugars. Carbohydr. Res. 1991;209:13–31.
- Welkie GW, Miller GW. Riboflavin excretion from roots of iron-stressed and reciprocally grafted tobacco and tomato plants. J. Plant Nutr. 1988;11:691–700.