Abstract
Chattonella antiqua isolated in 2010 showed extremely more potent fish-killing activities against red sea bream, Japanese horse mackerel, and blue damselfish than those of Chattonella marina isolated in 1985. Chemiluminescence and electron spin resonance (ESR) analyses suggested greater reactive oxygen species (ROS)-producing activity of C. antiqua than that of C. marina. Sodium benzoate, a hydroxyl radical scavenger, significantly suppressed the fish-killing activity of C. antiqua on blue damselfish. The chlorophyll level in the gill tissue of blue damselfish exposed to flagellate cells increased along with the exposure time, and the cell count of gill-associated C. antiqua estimated with chlorophyll level was higher than that of C. marina. These results suggest that the ROS-producing activity and affinity of Chattonella cells to the gill surface may be important factors influencing the fish-killing activity of Chattonella species.
Graphical abstract
ROS-mediated gill tissue damage by C. antiqua may lead to fish death
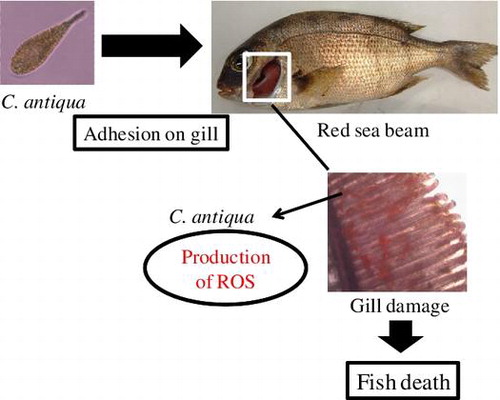
Harmful algal blooms (HABs) are a serious environmental issue. Concurrently with global warming, there have been a worldwide increase in the frequency, magnitude, and duration of HABs.Citation1,2) Hence, their threat to aquaculture, fisheries, and public health in coastal waters throughout the world has increased.Citation3) The raphidophycean flagellate Chattonella spp., such as Chattonella marina and Chattonella antiqua, are the most noxious HAB species, which cause serious damage to fish farms in Japan, especially to the aquaculture of yellowtail, Seriola quinqueradiata.Citation4) In addition to Japan, HABs due to Chattonella spp. often associated with fish-kill incidence have been reported in Australia, Netherlands, Brazil, and other area around the world.Citation5–8) Although the specific toxic mechanism of Chattonella spp. is still controversial, it has been suggested that suffocation is the direct cause of fish death by this flagellate species.Citation9–12) Previous studies have demonstrated that a decrease in partial pressure of oxygen in arterial blood is the earliest physiological change observed in fish exposed to C. marina.Citation13,14) In addition, several lines of evidence have suggested that excessive mucus on the gill surface, which is probably induced by C. marina, may interfere with O2 transfer, which in turn leads to asphyxia.Citation11,15)
In regard to the causative factor responsible for the fish-killing mechanism, it has been shown that Chattonella spp. generate reactive oxygen species (ROS), such as superoxide (O2−), hydrogen peroxide(H2O2), and hydroxyl radical (·OH).Citation16–20) Since ROS are toxic to living organisms,Citation21,22) the ROS generated by Chattonella spp. may be involved in gill tissue injury. This hypothesis may be supported by our previous finding that one strain of C. marina, which produces very low levels of O2-, showed lower toxicity to yellowtail than that by the other strain with higher O2- producing ability.Citation23) Since another raphidophycean flagellate Heterosigma akashiwo also showed ROS-mediated toxicity against rainbow trout, it seems likely that the production of ROS is a common characteristic of raphidophycean flagellates.Citation24)
In addition to ROS, previous studies have shown that Chattonella spp. produce several toxic substances, such as neurotoxins similar to the brevetoxins produced by Karenia brevis (formerly known as Gymnodinium breve and Ptychodiscus brevis), and hemagglutinating agents.Citation25–27) Matsusato and Kobayashi reported that neither the dead cells of C. antiqua nor cell-free filtrate of the culture of the flagellate cells was toxic to red sea bream.Citation28) Ishimatsu et al. also reported that ruptured C. marina showed no toxic effect on yellowtail, while there was a clear correlation between the cell’s ability to produce O2− and its fish-killing activity.Citation23) These findings suggest that live cells are essential for the toxicity of Chattonella spp. and that the toxic factors may be quite unstable in nature. However, the exact fish-killing mechanism of Chattonella is still open question, and plausible candidates for the toxic factors other than ROS have not been discovered yet. It is assumed that the toxic mechanism may not be straightforward, and multiple factors are involved in the complicated processes leading to fish death caused by Chattonella. Recent studies have suggested that ROS and certain lipids are synergistically involved in the ichthyotoxicity through chain reaction of lipid peroxidation.Citation29,30)
In 2010, we isolated C. antiqua from Shimabara Bay, Nagasaki, Japan, where the HAB caused by C. antiqua resulted in serious damage to local aquacultural industries. To gain insight into the fish-killing mechanism of Chattonella spp., in this study, comparative experiments were conducted to determine the fish-killing activities of the above-mentioned Chattonella spp., using three species of fish. The effects of the newly isolated C. antiqua on fish were compared to those of C. marina strain that was originally isolated in 1985 in Kagoshima, Japan, and has since been maintained in our laboratory, under artificial conditions.
Materials and methods
Cultures of Chattonella species
The raphidophytes C. marina and C. antiqua were isolated in Japan from Kagoshima in 1985, and Shimabara Bay in 2010, respectively. These flagellate cells were maintained in 100 mL flasks containing 40–50 mL of sterilized Erd–Schreiber-modified (ESM) medium under a 12:12-h photoperiod using a cool-white fluorescent lamp (200 ± 5 μmol/m2/s) at 26 °C.Citation19) The ESM medium was autoclaved for 15 min at 121 °C before use. Cell numbers were counted microscopically using a hemocytometer (Erma Inc., Tokyo, Japan). Cells from each culture were used from late exponential growth phase throughout the experiments, unless otherwise specified. Mass cultures of the flagellate cells were conducted with 5 L flasks containing 500 mL of ESM medium. To the flask, 40–50 mL of subcultured flagellate cells from late exponential growth phase was inoculated and then cultured for 10–15 days until it reached late exponential growth phase, under the conditions described above.
Preparation of ruptured C. antiqua
The ruptured cell suspension of C. antiqua at 10,000 cells/mL was prepared by ultrasonic treatment of the cell suspension in a bath-type sonicator (XL2020; Wakenyaku Co., Ltd., Kyoto, Japan) for 60 s at 20 °C. Microscopic observation confirmed that all cells were ruptured by this treatment. The ruptured cell suspension was immediately used for the red sea bream exposure experiment and chemiluminescence analysis as described below.
Determination of chlorophyll levels
The chlorophyll content per cell was measured spectrophotometrically following acetone extraction. Each culture was harvested by centrifugation (1,000 g, 10 min) and then 100% acetone was added to the cell pellet. After vigorous agitation by vortexing, the supernatant was obtained by centrifugation (10,000 g, 10 min). The chlorophyll content of the supernatant was spectrophotometrically determined at 664 and 630 nm.
Determination of protein content
The protein content of the flagellate cells was measured with the RC-DC Protein assay kit (BIO-RAD, Hercules, CA, USA) according to the manufacturer’s instruction. Bovine serum albumin (BSA) was used as a standard.
Exposure experiments
Juvenile Japanese horse mackerel (Trachurus japonicus, n = 35~50, mean body length = 10.1 ± 0.7 cm, body weight = 8.6 ± 1.8 g) and red sea bream (Pagrus major, n = 50~60, mean body length = 3.9 ± 0.3 cm, body weight = 0.9 ± 0.1 g) were supplied by Nagasaki Prefectural Institute of Fisheries, Japan, in spring from April to June. Adult blue damselfish (Chrysiptera cyanea, n = 40~50, mean body length = 5.9 ± 0.3 cm, and body weight = 3.8 ± 0.5 g) were purchased from a local fish retailor in Nagasaki, Japan. This fish species was available throughout a year. Red sea breams were exposed to either ruptured or live Chattonella cells, in a 10-L acrylic tank. To the acrylic tank containing 2.5 L of ESM medium and 10 red sea breams, either 2.5 L of C. marina or C. antiqua cell suspension in late exponential growth phase (20,000 cells/mL) was added. As a control, 10 red sea breams were kept in 5.0 L of ESM medium. The salinity and pH of each exposure medium was 25‰ and 8.0, respectively. The experiments were carried out at 26 ± 1 °C and dissolved oxygen (DO) was kept at 6.1 ± 0.6 mg/L by aeration during the exposure experiments. For Japanese horse mackerel (n = 10) and blue damselfish (n = 5), the exposure experiments were conducted in 15 and 10 L acrylic tanks with a total volume of 10.0 and 5.0 L, respectively, under the same conditions described above. To investigate the possible involvement of hydroxyl radicals in the fish-killing activity of C. antiqua, a blue damselfish exposure experiment was conducted in the absence or presence of sodium benzoate (final 10 and 50 mM), a hydroxyl radical scavenger.
Measurement of the superoxide anion (O2−)
To measure the superoxide levels of C. marina and C. antiqua, chemiluminescence analysis using L-012 (Wako Pure Chemical Industry, Co., Ltd., Osaka, Japan) as a superoxide-specific chemiluminescence probe was undertaken, as described previously.Citation31) Chemiluminescence responses of C. marina (40,000 cells/mL) and C. antiqua (20,000 cells/mL) were recorded for 30 s using a Bio-Orbit Luminometer (1254–001; Bio-Orbit Oy, Turku, Finland) after the addition of L-012 to each ruptured and live cell suspensions. Reaction mixtures consisted of 145 μL of each flagellate cell suspension, 50 μL of L-012 (10 μM), and either 5 μL of superoxide dismutase (SOD: Cu, Zn-SOD) solution (final concentration of 100 U/mL) or 5 μL of ESM medium. Chemiluminescence response measured in ESM medium alone was considered as a baseline for other measurements. All chemiluminescence measurements were conducted in triplicate at 26 °C using 1.5 mL microtube cuvettes.
Measurement of hydroxyl radicals
The hydroxyl radical levels in the flagellate cell suspensions (40,000 cells/mL) were measured by the electron spin resonance (ESR)-spin trapping assay as described previously.Citation20) In brief, 10 μL of DMPO solution (89 mM) was added to 90 μL of flagellate cell suspension. After 1 h of incubation at 27 °C, the reaction mixture was subjected to ESR (The EMX Plus, Bruker, Billerica, Massachusetts, USA). The measurement conditions of the ESR experiments were as follows: field sweep: 3327–3412 G, field modulation frequency: 100 kHz, modulation amplitude: 3 G, sweep time: 122.880 s, time constant: 163.840 ms, microwave frequency: 9.458 GHz, microwave power: 4 mW.
Estimation of the cell count of C. marina and C. antiqua associated with gill tissues of blue damselfish exposed to flagellate cells
For the estimation of the chlorophyll levels in the gill tissue of blue damselfish, the whole gill tissue of the blue damselfish in the experiment was immediately removed from the fish after exposure to flagellate cells at 20,000 cells/mL for 1, 2, and 3 h and immersed into acetone. After 10 min of extraction, the solution was transferred to a tube and the supernatant was obtained by centrifugation (10,000 g, 10 min). The level of chlorophyll in the supernatant was estimated spectrophotometrically as described above. Based on the calibration curve between flagellate cell count and chlorophyll content, the number of flagellate cells associated with the gill was estimated. The chlorophyll level in the extract prepared from normal gill tissue using same procedure was essentially at a negligible level.
Results
Characterizations of C. marina and C. antiqua
The average cell lengths of C. marina and C. antiqua were 30–50 and 50–130 μm, and the widths were 20–30 and 30–50 μm, respectively. The average protein and chlorophyll contents of C. marina and C. antiqua were 4.3 and 8.0 ng per cell, and 40 and 50 pg per cell, respectively.
Fish-killing activities of C. marina and C. antiqua
As shown in Fig. , red sea bream exposed to the culture of C. antiqua at a cell density of 10,000 cells/mL exhibited mortality. The first fish died after 40 min of exposure to the flagellate cells, and all fish were dead within 3.5 h. Japanese horse mackerel and blue damselfish were also highly sensitive to C. antiqua, and 80% and 100% of these fish species died within a few hours of exposure, respectively. Compared to the red sea bream, these fish species were slightly more resistant to C. antiqua. On the other hand, C. marina showed no significant toxic effect on these three fish species, and no dead fish were observed even after 24 h from the time of initial exposure. The fish-killing activity of C. antiqua against red sea bream was dependent on the cell density, with C. antiqua at 5,000 cells/mL showing lower toxicity than at 10,000 cells/mL, even if 100% of the fish died within 7 h (Fig. ).
Fig. 1. Fish mortality after exposure to Chattonella marina and Chattonella antiqua.
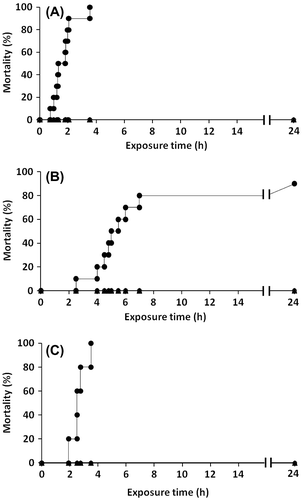
Fig. 2. Cell density-dependent toxicities of C. antiqua against red sea bream in comparison with C. marina.
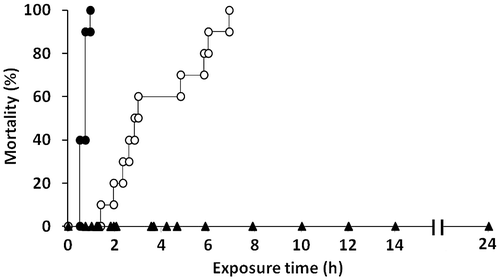
Superoxide generating activities of C. marina and C. antiqua
Superoxide generating activities of C. marina and C. antiqua were measured by analysis of chemiluminescence. After the addition of L-012 to each cell suspension, chemiluminescence response was recorded during 30 s. As shown in Fig. , a strong chemiluminescence response was observed in C. antiqua at 20,000 cells/mL, and the response almost completely disappeared in the presence of SOD. A chemiluminescence response was also observed in C. marina (40,000 cells/mL); however, it was at a much lower level than that observed for C. antiqua, even when measured at a two-fold higher cell density.
Fig. 3. L-012-mediated chemiluminescence responses of C. marina (△, ▲) and C. antiqua (○, ●).
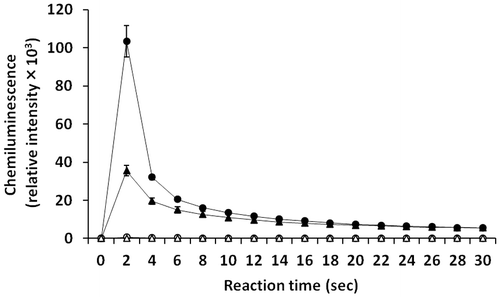
Hydroxyl radical levels of C. marina and C. antiqua
As shown in Fig. , a noticeable signal of DMPO-OH, which reflects the presence of hydroxyl radicals, was observed in the C. antiqua cell suspension (40,000 cells/mL) and was much stronger than that of C. marina (40,000 cells/mL).
Effects of sodium benzoate on the fish-killing activity of C. antiqua
As shown in Fig. , sodium benzoate at 10 mM demonstrated partial protection against the fish-kill induced by C. antiqua at 10,000 cells/mL, and complete protection at 50 mM, at which concentration no dead fish were observed even after 24 h of the initial exposure.
Fig. 5. Effects of sodium benzoate on the mortality of blue damselfish exposed to C. antiqua.
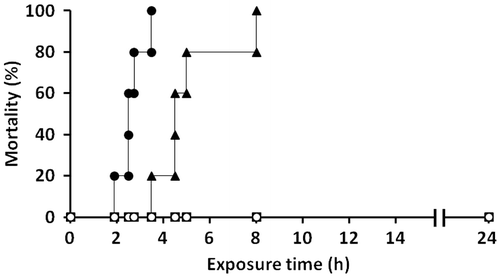
Fish-killing activity and superoxide production in ruptured C. antiqua cells
As shown in Fig. , ruptured C. antiqua cells did not demonstrate significant toxicity against red sea bream, and no dead fish were observed after 24 h of the initial exposure to ruptured cells prepared from C. antiqua (10,000 cells/mL). No significant chemiluminescence response was observed in the ruptured cell suspension.
Fig. 6. Fish-killing activity and ROS-producing activity of ruptured C. antiqua cells.
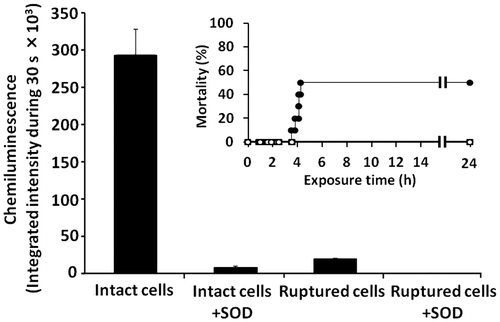
Estimation of flagellate cell count associated with the gills of blue damselfish exposed to C. marina and C. antiqua
As shown in Fig. , the estimated cell numbers of C. antiqua gradually increased along with the exposure time, with all the values were higher than those observed during exposure to C. marina.
Fig. 7. Estimation of cell numbers of C. marina and C. antiqua associated with the gills of blue damselfish exposed to the flagellates at 20,000 cells/mL.
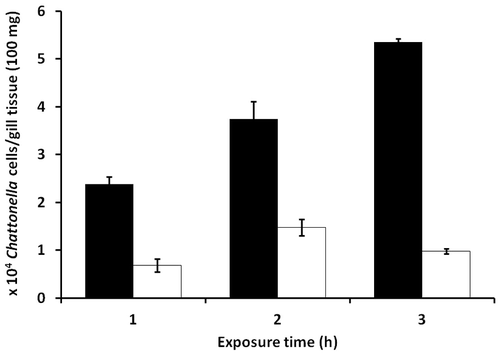
Discussion
In vitro cell culture-based bioassays have previously been developed to analyze toxic factors produced by HAB species. The developed assays have been suitable for the detection and characterization of toxic substances derived from HAB species.Citation30,32) In the case of Chattonella known as potent fish killer, however, exposure experiments using appropriate fish species is essential for the evaluation of the exact fish-killing activity. In our preliminary experiments, it was found that red sea bream and Japanese horse mackerel at their juvenile stage are relatively easy to handle and suitable for laboratory level small-scale exposure experiment. Blue damselfish is also useful, since this species is available throughout a year, and the body size of even fully growth one is small enough for laboratory experiments. Therefore, in this study, comparative studies on the fish-killing activities of C. marina and C. antiqua were conducted using these fish species. Exposure experiments revealed that C. antiqua had extremely potent and higher fish-killing activity than that by C. marina at least against the three fish species (Figs. and ). Differences in fish-killing activities between C. marina and C. antiqua had been observed in red sea bream exposure experiments conducted in previous years (data not shown). Although C. antiqua showed slightly different toxicity levels depending on the fish species, 80–100% of the fish exposed to this flagellate died within a few hours. However, C. marina was dramatically less toxic to these fish species, and no dead fish were observed even after 24 h exposure. The difference in toxicity to fish between C. marina and C. antiqua is too great to be simply explained by the differences in cell size or protein and chlorophyll content. In relation to Chattonella strains with different levels of toxicity, a previous study conducted in 1996 showed that a C. marina strain isolated in 1978 (strain 78) caused no mortality in yellowtails, whereas another C. marina stain isolated in 1985 (strain 85) was highly toxic to yellowtail under the same experimental conditions.Citation23) Strain 78 had been cultured for more than 10 years after the initial isolation, under laboratory conditions, without intervening cyst stage.Citation33) Since strain 78 was shown to be toxic to yellowtail in 1989, it was speculated that the loss of toxicity might have occurred during the long artificial cultivation period.Citation10) Although the mechanism of fish killing of Chattonella is still controversial, and the involvement of ROS in the toxicity of Chattonella has not yet been confirmed, it seems likely that the cell’s ability to produce ROS reflects the vital potential of the flagellate cell and might be correlated with the fish-killing activity. In fact, the ability of C. antiqua to produce ROS was much greater than that of C. marina. These results, together with previous findings, suggest that the ability of Chattonella cells to produce ROS is an important factor linked with the fish-killing activity. To further investigate the involvement of ROS in the toxicity of Chattonella, experiments of C. antiqua exposure to blue damselfish were carried out in the presence or absence of sodium benzoate, a hydroxyl radical scavenger. Among ROS, the hydroxyl radical is known to be the most toxic oxygen radical. This radical scavenger is also inexpensive relative to other ROS-scavengers. Sodium benzoate at 50 mM showed no toxic effect on fish or C. antiqua (data not shown). For these reasons, this reagent was selected for our experiments. Sodium benzoate demonstrated a potent protective effect in blue damselfish exposed to C. antiqua in a concentration-dependent manner. In the presence of 50 mM of sodium benzoate, all of the fish exposed to C. antiqua were still alive after 24 h from the initial time of exposure, while toxicity was confirmed in the absence of sodium benzoate under the same conditions. These results may support the theory that ROS, especially hydroxyl radicals, are involved in the toxicity of Chattonella to fish. Furthermore, consistent with previous studies, ruptured C. antiqua cells showed no significant toxicity on red sea bream, or ability to generate ROS, suggesting that the cellular integrity with active metabolism including ROS-producing activity is essential for the ichthyotoxicity. Since the ROS level detected in our strain of C. marina was not negligible level, there is a possibility that other toxic factors responsible for the fish-killing activity might become lower than that of C. antiqua concomitant with the decrease in ROS-generating activity. Further studies are necessary to identify such factors.
During the Chattonella-exposure experiments, the presence of Chattonella cells on the surface of the gill was observed. To estimate the number of Chattonella cells associated with the gill of the fish exposed to Chattonella, in this study, the amount of Chattonella-derived chlorophyll in the gill was extracted with acetone. The results indicated that the number of C. antiqua cells associated with gill tissue gradually increased with the exposure time, and the estimated cell number was greater for C. antiqua than for C. marina. These results suggest that C. antiqua may have a stronger affinity to gill surface in blue damselfish than that by C. marina. It has been reported that Chattonella cells have glycocalyx on their surface, and our previous study suggested that discharged glycocalyx from Chattonella cells was attached to the gill surface of the fish exposed to Chattonella, in addition to the Chattonella cells themselves.Citation34,35) Glycocalyx reportedly consists of complex carbohydrates and a carbohydrate–protein complex and was suggested to be bioactive.Citation34) Our previous study suggested that a superoxide-producing enzyme system might exist in the glycocalyx of C. marina.Citation36) Hori et al. reported that C. antiqua has lectin-like compounds and lectin receptors on the cell surface.Citation37) In addition, it was found that the ROS-producing activity of C. marina increased with the addition of fish mucus, suggesting that the interaction between fish mucus and the Chattonella cell surface may lead to the activation of the ROS-generation enzyme system located on the Chattonella cell surface.Citation38) The subsequent increase in ROS generation may be involved in the gill tissue damage during Chattonella exposure. Based on these findings, together with the results obtained in this study, it seems that the glycocalyx may play an important role in the interaction between Chattonella cells and gill surface, which appears to be responsible for the gill tissue damage. Further detailed analysis on the composition and structure of the glycocalyx, as well as its bioactivities, may provide an insight into the fish-killing mechanism of Chattonella. In this regard, our Chattonella strains with very different levels of fish toxicity will be useful for the elucidation of the mechanism of toxicity of Chattonella spp. Proteomic analysis especially focused on the glycocalyx of C. antiqua and C. marina is now planning to conduct, which may provide tips for the certain proteins linked with the differences in the fish-killing and ROS-generation activities between two strains.
Since C. marina has intracellular hemolytic agents even detectable in the ruptured cells, hemolytic activities of the ruptured cell suspensions of C. marina and C. antiqua were measured.Citation39) Although both ruptured cell suspensions showed slightly increased hemolytic activities as compared to intact cell suspensions, no significant difference between two strains was observed (data not shown). Hence, it seems unlikely that hemolytic activity is a factor involved in fish-killing activity of Chattonella spp.
In conclusion, C. antiqua isolated in 2010 showed extremely potent and higher fish-killing activity against three fish species than that by C. marina isolated in 1985. The potent toxicity may be partly explained by greater ROS-producing activity and stronger affinity to gill surface of C. antiqua than of C. marina. Our Chattonella strains with quite different ichthyotoxicity are useful for shedding light on the toxic mechanism of Chattonella.
Author contributions
KC, YY, MY, MU, and TO conceived the experiments. JS, TN, TN, and MU performed the experiments and analyzed the data together with KC, YY, and MY. YY, MY, DK, and TO provided valuable advice. DK and TO equally contributed to the manuscript writing. All authors discussed the results and commented on the manuscript.
Funding
This work was financially supported in part by a Grant-in-Aid for Scientific Research from the Ministry of Education, Culture, Sports, Science and Technology of Japan and Nagasaki University Major Research Project (Research Initiative for Adaptation to Future Ocean Change).
Disclosure statement
No potential conflict of interest was reported by the authors.
References
- Moore SK, Trainer VL, Mantua NJ, et al. Impacts of climate variability and future climate change on harmful algal blooms and human health. Environ. Health. 2008;7:S4.10.1186/1476-069X-7-S2-S4
- Paerl HW, Huisman J. Climate change: a catalyst for global expansion of harmful cyanobacterial blooms. Environ. Microbiol. Rep. 2009;1:27–37.10.1111/emi4.2009.1.issue-1
- Anderson DM. Turning back the harmful red tide. Nature. 1997;388:513–514.10.1038/41415
- Okaichi T. Red tides problem in Seto Inland Sea, Japan. In: Okaichi T, Anderson DM, Nemoto T, editors. Red tides: biology, environmental science, and toxicology. New York, NY: Elsevier; 1989. p. 137–142.
- Munday BL, Hallegraeff GM. Mass mortality of captive southern bluefin tuna (Thumus maccoyii) in April/May 1996 in Boston Bay, South Australia: a complex diagnostic problem. Fish Pathol. 1998;33:43–350.
- Vrieling EG, Koeman RPT, Nagasaki K, et al. Chattonella and Fibrocapsa (Raphidophyceae): first observation of, potentially harmful, red tide organisms in Dutch coastal waters. Neth. J. Sea Res. 1995;33:183–191.10.1016/0077-7579(95)90005-5
- Odebrecht C, Abreu PC. Raphidophycean in southern Brazil. Harmful Algal News. 1995;13:4.
- Imai I, Yamaguchi M. Life cycle, physiology, ecology and red tide occurrences of the fish-killing raphidophyte Chattonella. Harmful Algae. 2012;14:46–70.10.1016/j.hal.2011.10.014
- Endo M, Foscarini R, Kuroki A. Electrocardiogram of a marine fish, Pagrus major, exposed to red tide plankton, Chattonella marina. Mar. Biol. 1988;97:477–481.10.1007/BF00391043
- Ishimatsu A, Maruta H, Tsuchiyama T, et al. Respiratory, ionoregulatory and cardiovascular responses of the yellowtail Seriola quinqueradiata to exposure to the red tide plankton Chattonella. Nippon. Suisan. Gakk. 1990;56:189–199.10.2331/suisan.56.189
- Ishimatsu A, Sameshima M, Tamura A, et al. Histological analysis of the mechanisms of Chattonella-induced hypoxemia in yellowtail. Fish. Sci. 1996;62:50–58.
- Ishimatsu A, Maruta H, Oda T, et al. A comparison of physiological responses in yellowtail to fatal environmental hypoxia and exposure to Chattonella marina. Fish. Sci. 1997;63:557–562.
- Ishimatsu A, Tsuchiyama Takashi, Yoshida M, et al. Effect of Chattonella Exposure on Acid-Base Status of the Yellowtail. Nippon. Suisan. Gakk. 1991;57:2115–2120.10.2331/suisan.57.2115
- Tsuchiyama T, Ishimatsu A, Oda T, et al. Effect of Chattonella Exposoure on Plasma Catecholamine Levels in the Yellowtail. Nippon. Suisan. Gakk. 1992;58:207–211.10.2331/suisan.58.207
- Hishida Y, Ishimatsu A, Oda T. Mucus blockade of lamellar water channels in yellowtail exposed to Chattonella marina. Fish. Sci. 1997;63:315–316.
- Oda T, Moritomi j, Kawano I, et al. Catalase- and superoxide dismutase-induced morphological changes and growth inhibition in the red tide phytoplankton Chattonella marina. Biosci. Biotechnol. Biochem. 1995;59:2044–2048.10.1271/bbb.59.2044
- Oda T, Nakamura A, Shikayama M, et al. Generation of reactive oxygen species by raphidophycean phytoplankton. Biosci. Biotechnol. Biochem. 1997;61:1658–1662.10.1271/bbb.61.1658
- Kawano I, Oda T, Ishimatsu A, et al. Inhibitory effect of the iron chelator desferrioxamine (Desferal) on the generation of activated oxygen species by Chattonella marina. Mar. Biol. 1996;126:765–771.10.1007/BF00351343
- Oda T, Ishimatsu A, Shimada M, et al. Oxygen-radical-mediated toxic effects of the red tide flagellate Chattonella marina on Vibrio alginolyticus. Mar. Biol. 1992;112:505–509.10.1007/BF00356297
- Oda T, Akaike T, Sato K, et al. Hydroxyl radical generation by red tide algae. Archs. Biochem. Biophys. 1992;294:38–43.10.1016/0003-9861(92)90133-H
- Halliwell B, Gutteridge JMC. Oxygen toxicity, oxygen radicals, transition metals and disease. Biochem. J. 1984;219:1–14.10.1042/bj2190001
- Oda T, Akaike T, Hamamoto T, et al. Oxygen radicals in influenza-induced pathogenesis and treatment with pyran polymer conjugated SOD. Science. 1989;244:974–976.10.1126/science.2543070
- Ishimatsu A, Oda T, Yoshida M, et al. Oxygen radicals are probably involved in the mortality of yellowtail by Chattonella marina. Fish. Sci. 1996;62:836–837.
- Yang CZ, Albright LJ, Yousif AN. Oxygen-radical-mediated effects of the toxic phytoplankter Heterosigma carterae on juvenile rainbow trout Oncorhynchus mykiss. Dis. Aquat. Org. 1995;23:101–108.10.3354/dao023101
- Khan D, Ahmed MS, Arakawa O, et al. Properties of neurotoxins separated from a harmful red tide organism Chattonella marina. Israel. J. Aquacult. 1995;47:137–141.
- Khan S., Arakawa O., Onoue Y., et al. A toxicological study of the marine phytoflagellate, Chattonella antiqua (Raphidophyceae). Phycologia. 1996;35:239–244.10.2216/i0031-8884-35-3-239.1
- Ahmed MS, Khan S, Arakawa O, et al. Properties of hemagglutinins newly separated from toxic phytoplankton. Biochim. Biophys. Acta. 1995;1243:509–512.10.1016/0304-4165(94)00184-Y
- Matsusato T, Kobayashi H. Studies on death of fish caused by red tide. Bull. Nansei Reg. Fish. Res. Lab. 1974;7:43–67. (in Japanese with English abstract).
- Marshall JA, Nichols PD, Hamilton B, et al. Ichthyotoxicity of Chattonella marina (Raphidophyceae) to damselfish (Acanthochromis polycanthus): the synergistic role of reactive oxygen species and free fatty acids. Harmful Algae. 2003;2:273–281.10.1016/S1568-9883(03)00046-5
- Dorantes-Aranda JJ, Seger A, Mardones JI, et al. Progress in understanding algal bloom-mediated fish kills: the role of superoxide radicals, phycotoxins and fatty acids. PLOS ONE. 2015;10:e0133549.10.1371/journal.pone.0133549
- Kim D, Yamasaki Y, Yamatogi T, et al. The possibility of reactive oxygen species (ROS)-independent toxic effects of cochlodinium polykrikoides on damselfish (Chromis caerulea). Biosci. Biotechnol. Biochem. 2009;73:613–618.10.1271/bbb.80693
- Katsuo D, Kim D, Yamaguchi K, et al. A new simple screening method for the detection of cytotoxic substances produced by harmful red tide phytoplankton. Harmful Algae. 2007;6:790–798.10.1016/j.hal.2007.04.002
- Imai I. Cyst formation of the noxious red tide flagellate Chattonella marina (Raphidophyceae) in culture. Mar. Biol. 1989;103:235–239.10.1007/BF00543353
- Yokote M, Honjo T. Morphological and histochemical demonstration of a glycocalyx on the cell surface of Chattonella antiqua, a ‘naked flagellate’. Experientia. 1985;41:1143–1145.10.1007/BF01951699
- Kim D, Okamoto T, Oda T, et al. Possible involvement of the glycocalyx in the ichthyotoxicity of Chattonella marina (Raphidophyceae): immunological approach using antiserum against cell surface structures of the flagellate. Mar. Biol. 2001;139:625–632.
- Kim D, Nakamura A, Okamoto T, et al. Mechanism of superoxide anion generation in the toxic red tide phytoplankton Chattonella marina: possible involvement of NAD(P)H oxidase. Biochim. Biophys. Acta. 2000;1524:220–227.10.1016/S0304-4165(00)00161-6
- Hori K, Ogata Takehiko, Kamiya H, et al. Lectin-like compounds and lectin receptors in marine microalgae: hemagglutination and reactivity with purified lectins. J. Phycol. 1996;32:783–790.10.1111/j.0022-3646.1996.00783.x
- Nakamura A, Okamoto T, Komatsu N, et al. Fish mucus stimulates the generation of superoxide anion by Chattonella marina and Heterosigma akashiwo. Fish. Sci. 1998;64:866–869.
- Kuroda A, Nakashima T, Yamaguchi K, et al. Isolation and characterization of light-dependent hemolytic cytotoxin from harmful red tide phytoplankton Chattonella marina. Comp. Biochem. Physiol. C-Toxicol. Pharmacol. 2005;141:297–305.10.1016/j.cca.2005.07.009