Abstract
Rice flour was stored at 15 °C/9 months, at 35 °C/14 days, or dry-heated at 120 °C/20 min. The breadmaking properties baked with this rice flour/fresh gluten flour deteriorated. In addition, the rice flour was mixed with oil in water vigorously, and oil-binding ability was measured. Every rice flour subjected to storage or dry-heated at 120 °C showed higher hydrophobicity, owing to changes in proteins. Then, proteins in the stored rice flour were excluded with NaOH solution, and bread baked with the deproteinized rice flour showed the same breadmaking properties as unstored rice flour/fresh gluten flour. The viscoelasticity of wheat glutenin fraction decreased after the addition of dry-heated rice flour in a mixograph profile. DDD staining increased Lab in color meter, which suggested an increase in SH groups in rice protein. The increase in SH groups caused a reduction in wheat gluten protein resulting in a deterioration of rice bread quality.
Graphical Abstract
Effect of 0.2% NaOH treatment of Akimasari rice flour on breadmaking properties of rice flour/flesh gluten flour.
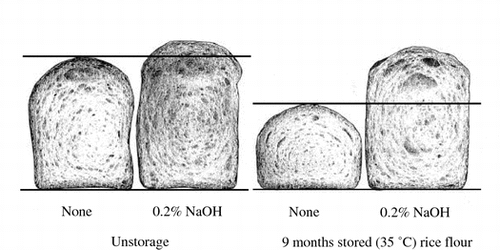
Rice bread has been produced in Japan since 2000. Since gluten protein is not present in rice flour, the volume of rice bread was poor. Wheat gluten is important for remarkable bread volume. Gluten is composed of glutenin and gliadin. It has been known that glutenin (105–several million) contributes elasticity and strength to wheat flour dough; when stretched, it resists and returns to its original shape. On the other hand, gliadin (3 × 104–12.5 × 104) stretches easily without resistance. Combined, these proteins provide a viscoelastic character to a dough, which allows for the entrapment of gas and prevents that gas from escaping. Bubbles in dough can expand and allow dough to become sufficiently porous to provide a desirable textured low-density product when baked.Citation1) It is known that rice proteins, mainly glutelin (75–90%), do not show such a viscoelasticity, indicating poor volume of rice bread. Rice bread requires the addition of gluten protein to have same viscoelasticity as wheat flour dough. Rice flour has been baked with gluten flour; however, it was known when the rice flour was stored at room temperature for several months the breadmaking properties (bread height (mm) and specific volume (cm3/g)) when baked with fresh gluten flour deteriorated. It is possible that when different cereal seed flours, such as rice flour and wheat flour, were blended during breadmaking, some interaction among them may occur, and interfere with breadmaking quality.
Tabara et al.Citation2) reported that rice flour showed a high affinity to oil (oil-binding ability) (hydrophobicity) when it was heat-treated at 120 °C for 120 min; that study showed that higher oil-binding ability of rice flour stored at room temperature (15 or 35 °C) could be obtained after a long storage period. The oil-binding ability of the rice flour was not affected by the treatments with diethyl ether or boiled chloroform/methanol (2:1) solution, which suggested no relationship to the oil in the rice flour, but was lost upon alkali (0.2% NaOH solution) or pepsin treatment, which suggested its relationship to rice proteins. However, it was not clear how the hydrophobicity of this stored or dry-heated rice flour reacted with fresh gluten flour, and whether the breadmaking properties deteriorated. It was also reported by SeguchiCitation3) that aged or dry-heated wheat starch granules showed a high oil-binding ability, which was due to change in starch granule surface proteins from hydrophilic to hydrophobic nature. Nakamura et al.Citation4,5) and Ozawa and SeguchiCitation6,7) reported that improvements of Kasutera cake volume and pancake textures by the hydrophobicity (lipophilization) of wheat starch granules caused by aging or dry-heating in wheat flour, respectively.
Wheat gliadin exists as single chains. Disulfide linkages exist, but they link cysteine R groups (a side groups) in the same chain. Glutenin makes up approximately 55–70% of the gluten complex. The disulfide bonding occurs toward the end of the chains, so, in effect, the glutenin molecule is linear.Citation8) When aged or dry-heated at 120 °C, rice flour showed a large hydrophobicity. In this study, stored rice flour (15 or 35 °C) or dry-heated rice flour at 120 °C were baked with fresh gluten flour, and breadmaking deterioration was evaluated. It was possibly thought that the buried hydrophobic sites such as sulfhydryl group in rice protein turned to naked state in rice flour, which could bind to oil. It is possible for redox reaction to occur between hydrophobic glutelin (naked sulfhydryl group) and glutenin, and make a smaller glutenin which could not entrap of gas from escaping. To establish the presence of naked SH groups in dry-heated rice proteins, DDD (6, 6′-dihydroxy-2, 2′-dinapthl disulfide) staining of SH groupsCitation9) was performed in this experiment.
The purpose in this experiment was to produce a remarkable rice bread even if baked with stored rice flour and fresh gluten flour.
Materials and methods
Materials
Japonica-type rice (Oryza sativa L. japonica, cv. Akimasari) and Indica–Japonica hybrid-type rice (Oryza sativa L. indica-japonica hybrid, cv. Mizuhochikara) were used in this experiment. Rice flour was prepared by Kumamoto Flour Milling Co. Ltd., Kumamoto, Japan. Mean diameters of the rice flour Akimasari 29.30 μm and Mizuhochikara 30.24 μm were determined using a laser diffraction particle size analyzer (Mastersizer 2000; Malvern Instruments Ltd, UK). Protein was determined by Kjeldahl method, and protein conversion was performed using the formula N × 5.7.Citation10) Ash was determined by the AACC International method (08-01, 2000)Citation10) at 14.0% moisture balance. Olive oil was purchased from Nissin Oillio Group, Ltd. (Tokyo, Japan), which was slightly yellowish in color, making it easier to identify. Gluten flour was purchased from commercial sources (Manildra Flour Mills Pty Ltd., NSW Australia).
Chemical reagents
DDD (6, 6′-dihydroxy-2, 2′-dinapthl disulfide, C20H14O2S2 = 350.45) was commercially purchased from Tokyo Chemical Industry Co., Ltd., Tokyo, Japan. Sodium 5,5-diethylbarbiturate (veranol acetate, C8H11N2SNaO3 = 206.17) and fast blue B salt (C14H12N4O2Cl2·ZnCl2 = 475.47) were purchased from Sigma-Aldrich Co., LLC., Tokyo, Japan.
Separation of gliadin and glutenin from gluten
Wheat flour was acetic acid fractionated by the method of Seguchi et al.Citation11) with slight modification, and gluten fraction was obtained. Gliadin and glutenin were obtained by the method of Osborn.Citation12) Mixture of the gluten (5.0 g) and 70% ethanol solution (200 mL) was stirred for 4 h at room temperature and homogenized for 30 s in LABO STIRRER L-35 (Yamato Scientific Co., Ltd., Tokyo, Japan). The suspension was stirred overnight in a cold room (4 °C) and centrifuged at 3000 rpm for 10 min at room temperature. Supernatant fraction (gliadin) and pallet fraction (glutenin) were obtained and freeze-dried.
Measurements of amylose contents in rice starch
Amylose contents in Akimasari 19.2% and Mizuhochikara 22.9% were determined in Bran + Luebbe GmbH-AutoAnalyzer (Bran + Luebbe GmbH, Germany) by KI/I2 method.
Stored treatments of rice flour
In case of commercial storage method (1), rice flour (1 kg) was packed in a nylon polyethylene bag with a sealed container of free-oxygen-absorbing agent Ageless (Mitsubishi Gas Chemical Company, INC. Tokyo, Japan), put in a corrugated cardboard and stored in 15 or 35 °C storehouse for 1–9 months. In other case of storage method (2), rice flour (100 g) was put into an iron plate (25 × 34 × 3 cm in height) at a depth of 0.5–1.0 cm for homogeneity in the heat-treating process at 35 °C for 1, 3, 7, and 14 days or at 120 °C for 10, 20, 40, 60, and 120 min in a drying oven (Sanyo Co., Ltd., Osaka, Japan). Samples were not sealed and opened to the atmosphere, and we measured the moisture contents in rice flour according to Tsutsumi and Nagahara.Citation13) The treated rice flour was left at room temperature for one hour, and then stirred and sieved through an iron sieve three times.
Determination of oil-binding ability of treated rice flour
The affinity of rice flour with oil (oil-binding ability) was evaluated by the oil-binding test of SeguchiCitation14) with slight modification. Stored or heat-treated rice flour (2.0 g), oil (4.0 mL) and water (20 mL) were shaken vigorously in a test tube (diameter 35 mm, height 20.5 cm) on a shaker (VORTEX GENIUS 3, IKA Japan K.K., Osaka, Japan) for 60 s. The oil saturated the rice flour in this experiment which showed that the amount of oil (4.0 mL) was enough to bind to the highest hydrophobic rice flour (2.0 g). The volume and height of the test tube were preliminarily measured using water. The oil bound to the rice flour sank in the water. The volume (mL) of the sunken oil/rice flour was measured.
Deproteinizing of rice flour with 0.2% NaOH solution
To extract proteins from the rice flour (250 g), 750 mL of 0.2% NaOH solution was added and stirred with a magnetic stirrer (Magnetic Stirrer IS-5G-I, Ikeda Scientific Co., Ltd., Tokyo, Japan) for 1 h at room temperature, and the suspension was then centrifuged at 1,600 rpm for 10 min. This process was repeated five times. The rice flour was further washed with water (750 mL) for 10 times until the red color due to phenolphthalein solution of the supernatant disappeared. The rice flour was dried at room temperature. Protein contents were determined as above.
Baking tests with rice flour/fresh gluten flour
Rice flour (moisture content 13.3%; 184.9 g), gluten (moisture contents 6.55%; 32.6 g), compressed yeast (6.5 g), sugar (10.9 g), salt (2.2 g), and water (163.1 mL) were mixed in a National automatic home bakery SD BT103 (Panasonic Corp., Osaka, Japan) for 25 min. The bread dough was then removed from the bread maker and divided into 120 g pieces, rounded, molded, and placed in baking pans. The bread dough was further proofed for 70 min at 38 °C. Baking was performed at 210 °C for 30 min in a DN-63 deck-style oven (Yamato Scientific Co., Tokyo, Japan), and the bread was removed from the pan and cooled for 1 h at a room temperature. The bread height (mm), weight (g), and volume (cm3) were measured, and crumbs were visually evaluated. The bread volume was measured by the rapeseed displacement method.
Mixograph tests of fresh rice flour/gluten flour
Mixograph test was performed as reported by Nakamura and Seguchi.Citation15) Rice flour (Mizuhochikara) 8.5 g (moisture content at 13.3%), gluten flour 1.5 g (moisture content at 6.55%), and water 8.0 mL were taken in a ten-gram mixograph (National MFG. Company Nebr. USA). In case of gliadin and glutenin, gliadin 4.5 g and glutenin flour 1.5 g were mixed with rice flour 5.5 and 8.5 g, respectively, and were taken with 8.0 mL of water in a Ten-gram Mixograph. The mixing speed of mixograph was 86 rpm read.
DDD (6,6′-dihydroxy-2,2′-dinapthl disulfide) staining of SH groups of proteins in treated rice flour
DDD staining of the sulfhydryl groups of proteins in dry-heated rice flour was performed by the method of MortonCitation9). DDD (40 mg) was dissolved in 24 mL of ethanol and mixed with 56 mL of veranol acetate buffer at pH 8.5Citation9). Rice flour (1.0 g) in 10 mL of the DDD solution was stirred at 50 °C for 60 min and centrifuged at 500 rpm for 3 min at room temperature. Precipitated rice flour was washed with water (20 mL) twice. To exclude free DDD, the rice was washed with 20 mL of acetic acid (pH 4.0–4.5) for 1 min at room temperature and centrifuged, and this step was performed in the same manner in 50, 60, 70, 80, and 99.5% (v/v) ethanol, ethanol/ether (1:1), and ether, in order. The rice flour was next washed with ethanol/ether (1:1), 99.5, 80, 70, 60, and 50% (v/v) ethanol, and water in this order. After washing, the rice flour was stirred with 10 mL of diazonium salt (fast blue B salt) solution, which was prepared as follows; diazonium salt (1 mg) was dissolved in 1 mL of 0.1 M potassium phosphate dibasic and monobasic buffer, pH 7.4Citation9) for 1 min at room temperature and centrifuged. The rice flour was further washed with 20 mL of water 9 times and dried at room temperature. This staining step was repeated three times. Next, the color of the staining rice flour was measured by chromaticity diagram for the L, a, b color specification system with a Hunter Lab colorimeter of the NE 2000 color meter (Nippon Denshoku Co., Tokyo, Japan). The L, a, and b values, respectively, indicate white–black, red–green, and yellow–blue. Presence of SH groups was indicated by a reddish color.
Statistical analysis
A statistical software package (Ver. 6.1, SPSS Inc., Chicago, IL) was used for the statistical analyses. Experiments were performed three times.
Results and discussion
Baking tests with rice flour/ fresh gluten flour
Rice flour (Akimasari) was subjected to 15 or 35 °C for 1–9 months. The storage method (1) was indicated in Materials and Methods section. Rice flour was subjected to baking tests and to determination of oil-binding ability. The excellent breadmaking results of unstored rice flour (bread height; 61.98 mm and specific volume; 2.68 cm3/g) at 15 °C gradually decreased throughout 9 months (final bread height was 51.37 mm and specific volume was 2.01 cm3/g) (Figs. and ). On the other hand, lower oil-binding ability in unstored rice flour (9.2 mL/2.0 g of rice flour) gradually increased to 11.3 mL/2.0 g, showing a higher hydrophobicity of stored rice flour (Fig. ). The results of breadmaking properties were related to oil-binding ability (r between bread height and oil-binding ability was −0.769, and between specific volume and oil-binding ability was −0.758, respectively) (Fig. ). At 35 °C, almost the same results were obtained; however, the levels of decrease in bread height (mm) and specific volume (cm3/g) and increase in oil-binding ability were higher than those of at 15 °C (Figs. and ). The results of breadmaking properties were related to oil-binding ability (r between bread height and oil-binding ability was −0.833, and between specific volume and oil-binding ability was −0.888, respectively) (Fig. ).
Fig. 2. Effects of storage (15 °C for 0–9 months) on the volume of oil/rice flour sank in water and on bread height and specific volume baked with rice flour/wheat gluten.
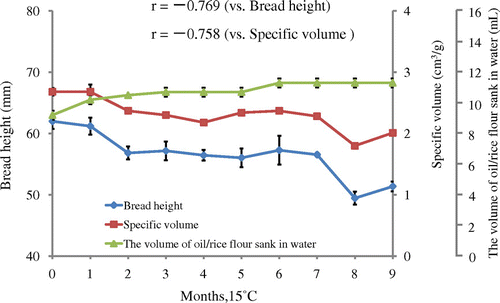
Fig. 4. Effects of storage (35 °C for 0–9 months) on the volume of oil / rice flour sank in water and on bread height and specific volume baked with rice flour/wheat gluten.
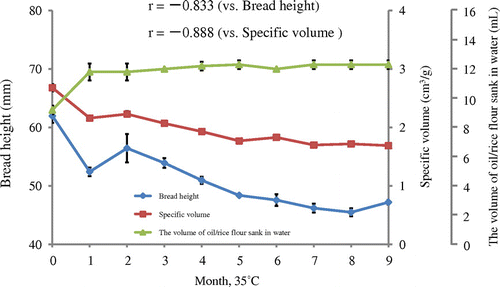
To clarify the differences in those results, the storage method 2 (storage in open conditions and depth of rice flour in 0.5–1.0 cm) for experiments in 35 or 120 °C was selected as in materials and methods. When the temperature was 35 °C, the experiments were performed for 1, 3, 7, and 14 days. The breadmaking test and oil-binding ability were tested simultaneously. In this experiment, we used two different samples (Akimasari rice (hard kernel) flour and Mizuhochikara rice (soft kernel) flour). The protein, ash, and moisture contents of the rice flour were: Akimasari, 6.4, 0.3, and 11.9%, and Mizuhochikara, 5.5, 0.2 and 12.5%, respectively (Table ). We could find more poor baking results and higher oil-binding ability in these conditions (Fig. ) than those in Fig. with Akimasari rice flour. The baking properties showed higher relationship between bread height and oil-binding ability in Akimasari (r = −0.965) in Mizuhochikara (r = −0.980), and between specific volume and oil-binding ability in Akimasari (r = −0.978), and in Mizuhochikara (r = −0.974), respectively (Fig. ). Mizuhochikara rice flour showed the same deterioration to Akimasari rice flour.
Table 1. General analysis of rice flour.
Fig. 5. Effects of storage (35 °C for 0–14 days) on the volume of oil / rice flour sank in water and on bread height and specific volume baked with rice flour/wheat gluten.
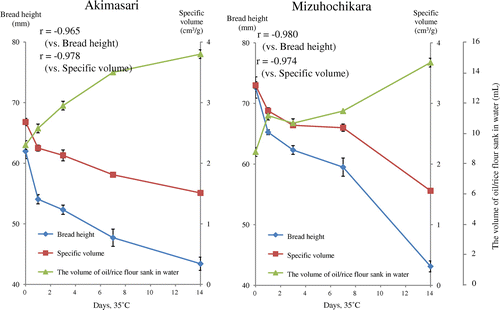
Rice flours was subjected to dry-heated at 120 °C for 10, 20, 40, 60, and 120 min and the breadmaking properties and oil-binding ability were measured in both rice flours (Figs. and ). Tabara et al.Citation16) observed that the hydrophobicity of wheat starch granule caused by dry-heat treatment was measured by sucrose fatty acid ester (SFAE) instead of oil. We measured the hydrophobicity of rice flour (35 °C, 14 days, in storage method 2) by SFAE (HLB = 13) instead of oil, and that was 13.5 mg SFAE/2 g rice flour. The relationship between breadmaking properties and oil-binding ability, r between bread height and oil-binding ability in Akimasari was −0.839 and in Mizuhochikara was −0.870, between specific volume and oil-binding ability in Akimasari was −0.905, and in Mizuhochikara was −0.880, respectively (Fig. ). It is generally known that chemical reaction increase 2–3 times higher or lower if the temperature increases or decreases by 10 °C. The temperature coefficient value (Q10) for oil-binding ability was determined by Tabara et al.Citation2).
Deproteinizing of rice flour with 02% NaOH solution
Tabara et al.Citation2) reported that the hydrophobicity of dry-heated rice flour was dependent on the rice proteins since the rice flour was deproteinized by 0.2% NaOH or treated with pepsin (pH 2.0) the oil-binding ability disappeared. It was reported by SeguchiCitation3) that the oil-binding ability of wheat starch granule was also lost by pepsin digestion of starch granule surface proteins. Akimasari rice flour was stored at 35 °C for 9 months (storage method 1), and was deproteinized with 0. 2% NaOH followed by washing with water until the red color due to phenolphthalein solution of the supernatant disappeared. Such stored and deproteinized sample was subjected to baking with fresh gluten flour. Table indicated that although protein contents in rice flour was 6.42%, it decreased to 0.17% after extraction with 0.2% NaOH of the stored rice flour, indicating that almost proteins in rice flour were removed with alkali. Unstored rice flour was also extracted with 0.2% NaOH and had 0.1% of protein content. Baking results are shown in Table and Fig. . Rice bread baked with unstored rice flour/fresh gluten flour showed bread height 61.98 mm (100%) and specific volume 2.68 cm3/g (100%), but after 9 months of storage the bread height was 47.22 mm (76%), and specific volume were 1.69 cm3/g (63%), and the same decreases were observed (Fig. ). It was known that the breadmaking properties when baked with stored and deproteinized rich flour/fresh gluten flour were similar to those of unstored rice flour (bread height 69.70 mm (112%), specific volume 3.15 cm3/g (118%)). The baking results of unstored and deproteinized rice flour/gluten flour indicated that bread height 72.44 mm (117%) and specific volume 3.41 cm3/g (127%), showing higher (10–30%) breadmaking properties. Hydrophobicity of naturally occurrence in rice proteins may contribute to the slight decrease in breadmaking properties of rice bread, and that was increased by storage at 15, 35 °C, or dry-heating at 120 °C.
Table 2. Effect of 0.2% NaOH treatment of Akimasari rice flour on breadmaking properties of rice flour/fresh gluten flour.
Fig. 8. Effect of 0.2% NaOH treatment of Akimasari rice flour on breadmaking properties of rice flour/fresh gluten flour.
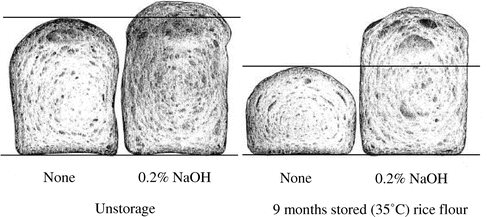
Effects of heat treatment (120 °C, 120 min) of rice flour on viscoelasticity of gluten, gliadin, and glutenin were examined in mixograph tests, respectively (Fig. ). Mixograph profiles indicated that the viscoelasticity of fresh rice flour/fresh gluten flour was decreased by heat treatment (120 °C, 120 min) (Fig. -(1) and -(2)) which was related to decrease in breadmaking properties of rice bread (Fig. ). Mixograph profile in fresh rice flour/fresh gliadin flour was not decreased by the heat treatment (Fig. -(3) and -(4)). However, mixograph profile in fresh rice flour/fresh glutenin flour was largely decreased by the heat treatment (Fig. -(5) and -(6)), which is indicating that heat-treated rice flour was not related to gliadin, but to glutenin. It is known that glutenin has a large number of disulfide-linking subunits, and exhibits strong elasticity (i.e., resistance when a mass of glutenin is stretched), which contributes to good breadmaking properties.
Fig. 9. Effect of heat treatment (120 °C, 2 h) of rice flour (Mizuhochikara) on gluten (9-1 and -2), gliadin (9-3 and -4), or glutenin (9-5 and -6) dough in mixograph profile. Gluten (1.5 g), gliadin (4.5 g), or glutenin (1.5 g) and rice flour (8.5, 5.5, and 8.5 g) were mixed with 8.0 mL water, respectively, and subjected to a Ten-gram Mixograph tests for 15 min.
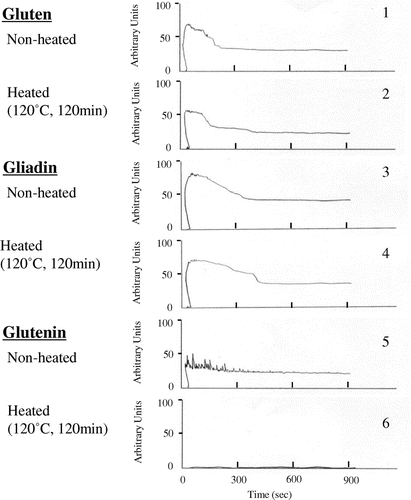
The structure of glutenin would be changed by mixing with rice flour which were stored for a long time at room temperature or heat treated at 120 °C within a short time. Such treatments of storage or heating proteins caused strong hydrophobicity in the rice flour.
Wen and LutheCitation17) reported that glutelin molecule consisted of α and β subunits with mol wt of 50.7 kD, and was determined that each molecule contained 1.4 free sulfhydryl residue and 1.1 disulfide residues per molecule. Wen and LutheCitation17) reported that the α and β subunits were linked by a disulfide bond and that either the α and β subunit contained a free sulfhydryl group. It is plausible thought that the glutelin changed from hydrophilic to hydrophobic by these treatments, in which buried hydrophobic sites would be exposed and show lipophilization, and simultaneously buried hydrophobic sulfhydryl groups would be exposed on the surface of the protein.
DDD (6,6′-dihydroxy-2, 2′-dinapthl disulfide) staining of SH groups in dry-heated rice protein meant changes of Lab values could be obtained (Fig. ); a (red) value in Lab increased from 10.5 to 11.2 indicating an increase in SH groups by dry-heating at 120 °C for 0 to 120 min.
Fig. 10. Effect of heat treatment on the sulfhydryl group of protein fractions in rice flour (Mizuhochikara) by DDD method.
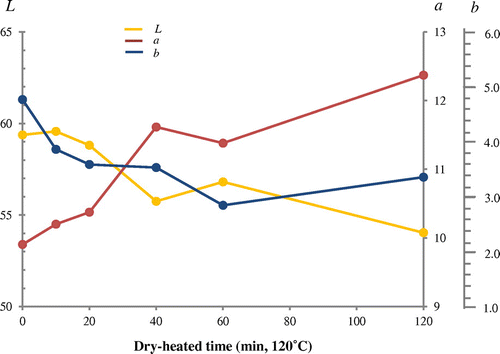
Large molecular glutenin was originally formed by disulfide bond of smaller subunits and contributes to a good volume of bread. The new sulfhydryl groups present in surface of stored or dry-heated rice protein (glutelin) could reduce the disulfide bond in glutenin. Reduced disulfide linkage changed to sulfhydryl groups, and molecular weight of glutenin changed to smaller one. Such reduced glutenin could not allow for the entrapment of gas and prevent that gas from escaping.
To produce excellent rice bread even if baked with stored rice flour and fresh gluten flour, we extracted rice proteins from stored rice flour with 0.2% NaOH and baked it with the deproteinized rice flour and fresh gluten protein. Resulting breadmaking properties such as bread height (mm) and specific volume (cm3/g) were similar to those of bread baked with unstored rice flour/fresh gluten flour.
Authors contributions
Masaharu Seguchi and Kotaro Matsunaga designed the study, and Mariko Nakagawa, Aya Tabara, and Masaharu Seguchi drafted the manuscript, and Yuki Ushijima reviewed the manuscript. Mariko Nakagawa, Aya Tabara, and Yuki Ushijima performed the experiment. All authors reviewed and approved the final manuscript.
Disclosure statement
No potential conflict of interest was reported by the authors.
References
- Casper JL, Atwell WA. Gluten-free baked products. Saint Paul (MN): AACC International; 2014. p. 2.
- Tabara A, Nakagawa M, Ushijima Y, Matsunaga K, Seguchi M. Effects of heat treatment on oil-binding ability of rice flour. Biosci. Biotechnol. Biochem. 2015;79:1629–1634.10.1080/09168451.2015.1039479
- Seguchi M. Oil-binding ability of heat-treated wheat starch. Cereal Chem. 1984;61:248–250.
- Nakamura C, Koshikawa Y, Seguchi M. Effect of flour changes due to storage on Kasutera cake volume. Food Sci. Technol. Res. 2007;13:351–355.10.3136/fstr.13.351
- Nakamura C, Koshikawa Y, Seguchi M. Increased volume of Kasutera cake (Japanese sponge cake) by dry heating of wheat flour. Food Sci. Technol. Res. 2008;14:431–436.10.3136/fstr.14.431
- Ozawa M, Seguchi M. Relationship between pancake springiness and interaction of wheat flour components caused by dry heating. Food Sci. Technol. Res. 2006;12:167–172.10.3136/fstr.12.167
- Ozawa M, Seguchi M. Effect of dry-heated prime starch and tailings fractions in wheat flour on pancake springiness. Cereal Chem. 2008;85:626–628.10.1094/CCHEM-85-5-0626
- Atwell WA. Wheat flour. Saint Paul (MN): American Association of Cereal Chemists; 2001. p. 29–31.10.1094/189112725X
- Morton WM. Effects of freezing and hardening on the sulfhydryl groups of protein fractions from cabbage leaves. Plant Physiol. 1969;44:168–172.10.1104/pp.44.2.168
- AACC International: approved methods of the American Association of Cereal Chemists. 10th ed. Methods 08-01 and 46-10. St. Paul, MN: The Association; 2000.
- Seguchi M, Hayashi M, Kanenaga K, Ishihara C, Noguchi S. Springiness of pancake and its relation to binding of prime starch to tailings in stored wheat flour. Cereal Chem. 1998;75:37–42.10.1094/CCHEM.1998.75.1.37
- Wrigley C, Békés F, Bushuk W. Gliadin and glutenin: the unique balance of wheat quality. Minnesota: AACC International. p. 11 2006.10.1094/9781891127519
- Tsutsumi C, Nagahara T. Effect of drying temperatures on the moisture determination of starches by oven drying method. Shokuryo kagaku Kenkyu Hokoku. 1961;15:118.
- Seguchi M. Oil-binding capacity of prime starch chlorinated wheat flour. Cereal Chem. 1984;61:241–243.
- Nakamura C, Seguchi M. Improving effects of stored wheat flour on pancake texture. Food Sci. Technol. Res. 2007;13:221–226.10.3136/fstr.13.221
- Tabara A, Oneda H, Murayama R, Matsui Y, Hirano A, Seguchi M. Determination of hydrophobicity of dry-heated wheat starch granules using sucrose fatty acid esters (SFAE). Biosci. Biotechnol. Biochem. 2014;78:1572–1576.10.1080/09168451.2014.923293
- Wen TN, Luthe DS. Biochemical characterization of rice glutelin. Plant Physiol. 1985;78:172–177.10.1104/pp.78.1.172