Abstract
We herein report the immunostimulatory effect of spinach aqueous extract (SAE) on mouse macrophage-like J774.1 cells and mouse primary peritoneal macrophages. SAE significantly enhanced the production of interleukin (IL)-6 and tumor necrosis factor-α by both J774.1 cells and peritoneal macrophages by enhancing the expression levels of these cytokine genes. In addition, the phagocytosis activity of J774.1 cells was facilitated by SAE. Immunoblot analysis revealed that SAE activates mitogen-activated protein kinase and nuclear factor-κB cascades. It was found that SAE activates macrophages through not only TLR4, but also other receptors. The production of IL-6 was significantly enhanced by peritoneal macrophages from SAE-administered BALB/c mice, suggesting that SAE has a potential to stimulate macrophage activity in vivo. Taken together, these data indicate that SAE would be a beneficial functional food with immunostimulatory effects on macrophages.
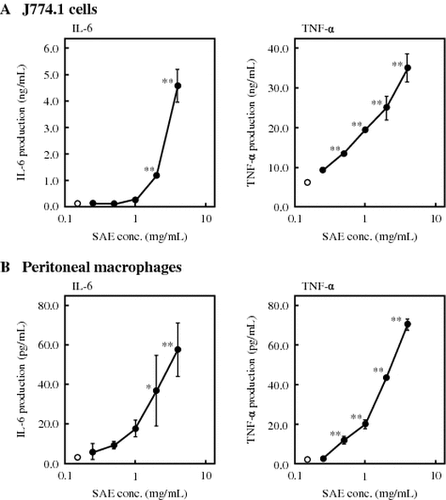
The innate immune system is the first line of host defense mechanism and mainly performed by neutrophils and macrophages. Macrophages are phagocytic cells differentiated from monocytes and play two roles in the innate immune system. The first is to recognize and phagocytize microbial pathogens. Macrophages recognize the molecular structures that are characteristic of microbial pathogens called pathogen-associated molecular patterns through the transmembrane proteins of pattern recognition receptors (PRRs) such as Toll-like receptors (TLRs).Citation1,2). Microbial phagocytosis triggers production of proinflammatory cytokines such as interleukin (IL)-6 and tumor necrosis factor (TNF)-α. These cytokines regulate the cell death in inflammatory tissues, modify vascular endothelial permeability, recruit blood cells to inflamed tissues, and induce the production of acute-phase proteins.Citation1,3) The second one is to present fragmented microbial pathogens to T cells as an antigen through macrophage-mediated phagocytosis. A fragmented peptide from microbial pathogens is bound to the major histocompatibility complex (MHC) class II molecules.Citation3) The MHC class II-antigen peptide complex is presented to the T cell receptor of CD4+ T lymphocytes, resulting in the activation of the adaptive immune response.Citation4) Thus, macrophages play an important role in the innate and adaptive immunity, and the macrophage activation leads to enhancements of the immune system.
Spinach, Spinacia oleracea Linn, is a vegetable rich in various nutrients such as carotenes, vitamins, folic acid, and iron. Various biological functions based on these nutrients have been reported; lutein, a non-provitamin A carotenoid, has been strongly implicated as a protective agent against age-related macular degeneration and cataracts.Citation5,6) Lutein also enhances matrix metalloproteinase-9 production and phagocytosis in murine macrophage-like RAW264.7 cells.Citation7) A glycolipid fraction from spinach has an inhibitory effect on the mammalian DNA polymerase activity and human cancer cell proliferation.Citation8,9) In addition, an aqueous spinach extract has been reported to effectively ameliorate asthmatic symptoms in an ovalbumin-challenged model mice, inducing a decrease in the CD4+ cell number and the levels of IL-4 and IL-13 in the lung.Citation10) An aqueous spinach extract also has an anti-allergic activity that controls degranulation of rat basophilic leukemia RBL-2H3 cells, resulting from the suppressed Ca2+ mobilization through the downregulated phosphorylation of Syk kinase and phosphatidylinositol 3-kinase (PI3K) in the signaling pathways involved in degranulation caused by the antigen-antibody interaction.Citation11) However, the immunostimulatory effect of water-soluble components in spinach has not yet to be reported. In this study, we investigated the immunostimulatory effect of spinach aqueous extract (SAE) on mouse macrophage-like J774.1 cells and mouse primary peritoneal macrophages.
Materials and methods
Reagents
Roswell Park Memorial Institute Medium (RPMI)-1640, penicillin, streptomycin, bovine serum albumin (BSA), fetal bovine serum (FBS), lipopolysaccharides (LPS) from Escherichia coli 026/B6 were products of Sigma-Aldrich (St. Louis, MO, USA). Aprotinin, pefabloc SC, and a protease inhibitor cocktail were obtained from Roche Applied Science (Basel, Switzerland). A phosphatase inhibitor cocktail was purchased from Nacalai Tesque (Kyoto, Japan). Goat anti-actin antibody (sc-1616) and horseradish peroxidase (HRP)-labeled anti-goat IgG antibody (sc-2020) were purchased from Santa Cruz Biotechnology (Santa Cruz, CA, USA). Rabbit anti-histone H3 antibody (#4499), rabbit anti-nuclear factor-κB (NF-κB) p65 antibody (#8242), mouse anti-IκBα antibody (#4814), rabbit anti-p44/42 mitogen-activated protein kinase (MAPK) antibody (#4695), rabbit anti-phosphorylated p44/42 MAPK antibody (#4370), rabbit anti-stress-activated protein kinase (SAPK)/c-Jun N-terminal kinase (JNK) antibody (#9258), rabbit anti-phosphorylated SAPK/JNK antibody (#4668), rabbit anti-p38 MAPK antibody (#9212), rabbit anti-phosphorylated p38 MAPK antibody (#4511), HRP-labeled anti-rabbit IgG antibody (#7074), and HRP-labeled anti-mouse IgG antibody (#7076) were from Cell Signaling Technology (Danvers, MA, USA). Ethyl (6R)-6-[N-(2-chloro-4-fluorophenyl)sulfamoyl]cyclohex-1-ene-1-carboxylate (TAK-242) was purchased from Chemscene (Monmouth Junction, NJ, USA).
Sample preparation
Freeze-dried spinach powder was suspended in 10 mM sodium phosphate buffer (NaPB; pH 7.4) at 0.1 g/mL at 4 °C for 24 h. The suspension was centrifuged at 10,000 × g at 4 °C for 20 min, and the supernatant was collected and dialyzed using a dialysis membrane with molecular weight cut off (MWCO) of 14,000 (Wako Pure Chemical Industries, Osaka, Japan) against 10 mM NaPB at 4 °C overnight. Then, the dialyzed supernatant was filtrated through a 0.22 μm membrane and used as SAE. Dry weight of SAE was measured by weighing a portion of freeze-dried samples. To evaluate an effect of heat treatment, 4 mg/mL of SAE was heated at each temperature (40, 60, 80, and 100 °C) for 10 min. To evaluate an effect of proteolytic enzyme, 4 mg/mL of SAE was treated with 500 μg/mL of trypsin (MP Biomedicals, Santa Ana, CA, USA) or proteinase K (Wako Pure Chemical Industries) at 37 °C for 15 h. The treated SAE was subsequently heated at 100 °C for 10 min in order to inactivate the enzyme.
Mice
BALB/c mice (Japan SLC, Shizuoka, Japan) were housed with a pelleted basal diet and water ad libitum, in an animal room under 12 h light/dark cycle at a temperature of 24 ± 1 °C and a humidity of 55 ± 5%. All animal experiments described herein were carried out in accordance with the protocol approved by the Laboratory Animal Care Committee of Ehime University. Mice were maintained in accordance with the Guidelines for the Care and Use of Laboratory Animals of Ehime University.
J774.1 cells
J774.1 cells, the mouse macrophage-like cell line, were obtained from Japanese Collection of Research Bioresources Cell Bank (Osaka, Japan). J774.1 cells were cultured in RPMI-1640 medium supplemented with 100 U/mL of penicillin, 100 μg/mL of streptomycin, and 10% FBS at 37 °C under humidified 5% CO2–95% air. Before evaluating the immunostimulatory effect, the adherent cells were gently detached with 0.02% ethylenediamine-N,N,N′,N′-tetraacetic acid (EDTA; Dojindo Laboratories, Kumamoto, Japan)-phosphate-buffered saline (PBS).
Mouse peritoneal macrophages
Mouse peritoneal macrophages were prepared as previously describedCitation12) with some modifications. Briefly, 8-week-old female BALB/c mice were injected with 3.0% thioglycollate medium (2 mL/mouse) in the peritoneum following 1 week acclimation. Four days after injection, the mice were sacrificed and injected with 3 mL of 0.05% EDTA-PBS in the peritoneum to collect thioglycollate-elicited peritoneal macrophages. The collected cells were centrifuged at 160 × g, 4 °C for 5 min, and the cell pellet was washed with PBS and centrifuged again. The cell pellet was suspended in 10% FBS-RPMI-1640 medium and cultured in a culture dish. After incubation for 3 h, the cells were washed with PBS three times to remove unattached cells such as neutrophils. Purity of peritoneal macrophages prepared by this method was estimated as more than 85% according to previous reports.Citation13–16) Before evaluating the immunostimulatory effect, PBS was added to the dish and the adherent cells were detached by pipetting on ice.
Cytokine production assay
Cells suspended in 10% FBS-RPMI-1640 medium were seeded into a 48-well cell culture plate (BD Falcon, Franklin Lakes, NJ, USA) at 2.5 × 105 cells/well and cultured at 37 °C overnight under humidified 5% CO2–95% air. After washing with PBS, the cells were treated with 500 μL of 10% FBS-RPMI-1640 medium containing various concentrations of SAE or 10 mM NaPB as control. In the case of J774.1 cells, the cells were incubated at 37 °C for 6 h, whereas peritoneal macrophages were for 24 h. After incubation, the concentrations of IL-6 and TNF-α in the culture media were measured by Mouse IL-6 enzyme-linked immunosorbent assay (ELISA) MAX Standard (BioLegend, San Diego, CA, USA) and Mouse TNF-alpha DuoSet ELISA (R&D Systems, Minneapolis, MN, USA), respectively, according to manufacturer’s instructions.
Real-time RT-PCR
Cells suspended in 10% FBS-RPMI-1640 medium were seeded into a 48-well cell culture plate at 2.5 × 105 cells/well and cultured at 37 °C overnight under humidified 5% CO2–95% air. After washing with PBS, the cells were treated with 500 μL 10% FBS-RPMI-1640 medium containing various concentrations of SAE or 10 mM NaPB as control and incubated at 37 °C for 6 h. Total RNA was isolated from the cells using Sepasol-RNA I Super G (Nacalai Tesque) according to the manufacturer’s instructions. Total RNA was used as a template for cDNA synthesis performed using MMLV-reverse transcriptase (Promega, Madison, WI, USA) and an oligo-(dT)20 primer (Toyobo, Osaka, Japan). The real-time PCR mixture, with a final volume of 20 μL, consisted of THUNDERBIRD SYBR qPCR Mix (Toyobo), 10 pmol of a forward primer, 10 pmol of a reverse primer, and 0.1 μg of a cDNA sample. The thermal cycling conditions were: 20 s at 95 °C, and 40 cycles of 3 s at 95 °C and 30 s at 60 °C. PCR products were measured on a StepOnePlus Real-time PCR System (Applied Biosystems, Foster City, CA, USA), and the relative gene expression was calculated based on the comparative CT method using a StepOne Software v2.1 (Applied Biosystems). The expression of β-actin was used as an endogenous control. Specific oligonucleotide sequences for each gene are as follows. Mouse β-actin: sense, 5′-CATCCGTAAAGACCTCTATGCCAAC-3′ and antisense, 5′-ATGGAGCCACCGATCCACA-3′; mouse IL-6: sense, 5′-A AGCCAGAGTCCTTCAGAGAGAT-3′ and antisense, 5′-TTGGATG GTCTTGGTCCTTAGC-3′; mouse TNF-α: sense, 5′-CTACTCCCAGGTTCTCTTCAA-3′ and antisense, 5′-GCAGAGAGGAGGTTGACTTTC-3′.
Phagocytosis activity
J774.1 cells suspended in 10% FBS-RPMI-1640 medium were seeded into a 48-well cell culture plate at 2.5 × 105 cells/well and cultured at 37 °C overnight under humidified 5% CO2–95% air. After washing with PBS, the cells were treated with 500 μL of 10% FBS-RPMI-1640 medium containing 4 mg/mL of SAE or 10 mM NaPB as control and incubated at 37 °C for 6 h. After washing with PBS, 500 μL of 10% FBS-RPMI-1640 medium containing 20 μg of zymosan A (Saccharomyces cerevisiae) BioParticles, Texas Red conjugate (Texas Red-labeled zymosan A; Invitrogen, Carlsbad, CA, USA) were added to the cells and incubated for 1 h under dark condition. After removing the medium, the cells were collected with PBS and centrifuged at 160 × g, 4 °C for 5 min. The cell pellet was suspended in 1 mL of 2% FBS-PBS, and phagocytosis activity was measured on a flow cytometer (FACSCalibur, BD Biosciences, San Jose, CA, USA).
Immunoblot analysis
J774.1 cells suspended in 10% FBS-RPMI-1640 medium were seeded into a 35 mm culture dish (BD Falcon) at 1.0 × 106 cells/dish and cultured at 37 °C overnight under humidified 5% CO2–95% air. After washing with PBS, the cells were treated with 2 mL of 10% FBS-RPMI-1640 medium containing 4 mg/mL of SAE, 10 mM NaPB as control, or 100 ng/mL of LPS as positive control and incubated at 37 °C for 15 min. Cytosolic and nuclear proteins were prepared using a CelLytic NuCLEAR Extraction Kit (Sigma) according to manufacturer’s instructions. Protein concentration was measured using a DC protein assay kit and equalized to the same protein concentration. The denatured proteins were then separated by SDS-PAGE and transferred onto a PVDF membrane (Hybond-P, GE Healthcare, Buckinghamshire, UK). The membrane was blocked with 5% skim milk dissolved in Tris-buffered saline containing 0.1% Tween 20 (TBS-T) for 1 h at room temperature. After washing with TBS-T, the membrane was incubated with a primary antibody in 5% BSA-TBS-T at 4 °C overnight. After washing with TBS-T, the membrane was incubated with a HRP-labeled anti-goat IgG antibody, HRP-labeled anti-mouse IgG antibody, or a HRP-labeled anti-rabbit IgG antibody in 5% skim milk-TBS-T at room temperature for 1 h. After washing with TBS-T, blots were developed by an ImmunoStar LD (Wako Pure Chemical Industries). Bands were visualized using a ChemiDoc XRS Plus apparatus (Bio-Rad Laboratories), and the chemiluminescent intensity was quantified using the Quantity One software (Bio-Rad Laboratories).
Treatment of TLR4 inhibitor
J774.1 cells suspended in 10% FBS-RPMI-1640 medium were seeded into a 48-well cell culture plate at 2.5 × 105 cells/well and cultured at 37 °C overnight under humidified 5% CO2–95% air. After washing with PBS, the cells were treated with 500 μL of 10% FBS-RPMI-1640 medium containing 0.2 μM of TAK-242 (TLR4 inhibitor) for 1 h. After washing with PBS, the cells were treated with 500 μL of 10% FBS-RPMI-1640 medium containing 4 mg/mL of SAE, 10 mM NaPB as control, or 2 ng/mL of LPS as positive control and incubated at 37 °C for 6 h. The concentration of IL-6 and TNF-α in the culture media were measured by ELISA as described above. The phagocytosis activity was measured on a flow cytometer as described above.
Immunostimularoty effect of SAE on peritoneal macrophages ex vivo
Ex vivo experiment was performed as previously describedCitation12,17) with some modifications. Briefly, 6-week-old female BALB/c mice were divided into 3 groups (6 mice per group) following 1 week acclimation. They were orally administered with 20 μL of 10 mM NaPB for the control group, SAE at 25 mg dry weight/kg body weight/day for high dose group, or 5 mg dry weight/kg body weight/day for low dose group for consecutive 14 days from day 0 to day 13. On day 10, the mice were injected with 3.0% thioglycollate medium (2 mL/mouse) in the peritoneum. On day 14, the mice were sacrificed and injected with 0.05% EDTA-PBS in the peritoneum, and peritoneal macrophages were collected and cultured as described above. To evaluate the effect of SAE on cytokine production, peritoneal macrophages were seeded into a 48-well cell culture plate at 2.5 × 105 cells/well and cultured for 24 h. The concentration of IL-6 and TNF-α in the culture media were measured by ELISA as described above.
Statistical analysis
Data obtained were expressed as mean ± standard deviation (SD). One-way ANOVA followed by Tukey’s test was used to assess the statistical significance of the difference against control. Values with *p < 0.05 or **p < 0.01 were considered to be statistically significant.
Results
Effects of SAE on cytokine production by J774.1 cells and peritoneal macrophages
The effect of SAE on cytokine production by J774.1 cells was first examined. SAE was added to culture medium of J774.1 cells at various concentrations, and the cytokine concentration in the medium was measured by ELISA after 6 h incubation. As shown in Fig. (A), SAE significantly enhanced the production of IL-6 and TNF-α by J774.1 cells in dose-dependent manners. When J774.1 cells were treated with 4 mg/mL of SAE, the production of IL-6 and TNF-α was increased 4500-fold and 4-fold compared to control, respectively. Next, the effect of SAE on cytokine production by peritoneal macrophages was examined. As shown in Fig. (B), SAE also significantly enhanced the production of IL-6 and TNF-α by peritoneal macrophages in dose-dependent manners. The production of IL-6 and TNF-α by peritoneal macrophages was increased 20-fold and 26-fold compared to control, respectively, by the treatment with 4 mg/mL of SAE. In addition, SAE showed no toxicity to either cells within the tested concentrations (data not shown). From these results, further experiments were performed with 4 mg/mL of SAE, which showed the highest effect on cytokine production without cytotoxicity.
Fig. 1. Effects of SAE on cytokine production by J774.1 cells and peritoneal macrophages.
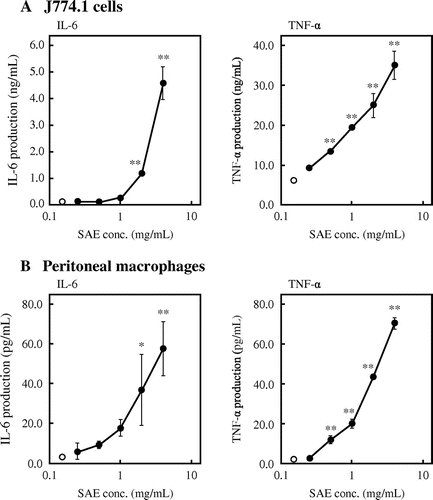
Effects of SAE on mRNA expression of cytokines in J774.1 cells and peritoneal macrophages
As described above, SAE enhanced the cytokine production by J774.1 cells and peritoneal macrophages. Therefore, the effect of SAE on mRNA expression of cytokines was examined. SAE was added to the culture medium of J774.1 cells and peritoneal macrophages at various concentrations, and the mRNA expression level of cytokines was evaluated by real-time RT-PCR. As shown in Fig. (A), SAE significantly facilitated the mRNA expression levels of IL-6 and TNF-α in J774.1 cells in dose-dependent manners. When J774.1 cells were treated with 4 mg/mL of SAE, the mRNA expression levels of IL-6 and TNF-α was increased 126-fold and 2-fold compared to control, respectively. In addition, SAE also facilitated the mRNA expression levels of IL-6 and TNF-α in peritoneal macrophages (Fig. (B)). The mRNA expression levels of IL-6 and TNF-α in peritoneal macrophages was increased 18-fold and 16-fold compared to control, respectively, by the treatment with 4 mg/mL of SAE. These results indicated that SAE stimulates the cytokine production by enhancing cytokine gene expression.
Fig. 2. Effects of SAE on mRNA expression of cytokines in J774.1 cells and peritoneal macrophages.
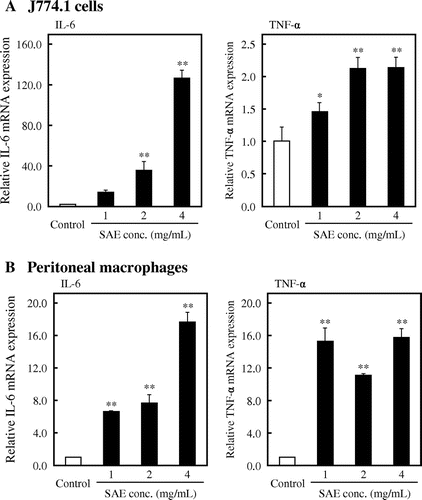
Effect of SAE on phagocytosis activity of J774.1 cells
Macrophages are phagocytic cells differentiated from monocytes in tissues and known as the effector cell in the innate immune response. Thus, we examined the effect of SAE on phagocytosis activity of J774.1 cells using Texas Red-labeled zymosan A. J774.1 cells were treated with SAE for 6 h, and Texas Red-labeled zymosan A was added to the culture medium after washing the cells with PBS. As shown in Fig. (A), the zymosan A-mediated phagocytosis activity was significantly enhanced by SAE. Fig. (B) showed that the phagocytosis rate of SAE-treated cells was increased to 53.3% (p < 0.01 vs. control), whereas the phagocytosis rate of control cells was 37.4%. These results suggested that SAE stimulates phagocytosis activity of macrophages.
Fig. 3. Effect of SAE on phagocytosis activity of J774.1 cells.
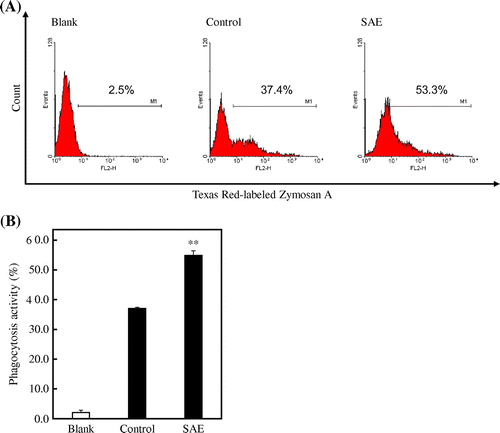
Effect of SAE on the signaling pathways involved in macrophage activation
We examined how SAE affects the signaling pathways involved in macrophage activation. It is known that macrophages are activated by a stimulus such as microbial pathogen and show the responses such as cytokine production and phagocytosis activity through MAPK and NF-κB cascades. J774.1 cells were treated with 4 mg/mL of SAE for 15 min, and the protein level of signal molecules was evaluated by immunoblot analysis. LPS, a major outer membrane component of gram-negative bacteria, was used as a positive control that binds to TLR4 on the cell surface of macrophages and stimulates the cytokine production through MAPK and NF-κB cascades.Citation18,19) As shown in Fig. (A), the phosphorylation levels of extracellular signal-regulated protein kinases (ERK), JNK, and p38 in J774.1 cells were increased by SAE. Especially, the phosphorylation levels of JNK and p38 were significantly increased. In addition, the protein level of IκBα decreased by LPS or SAE (Fig. (B)), suggesting that SAE enhances the degradation of IκBα. As shown in Fig. (C), the translocation of NF-κB into the nucleus was facilitated by the treatment of SAE. These results revealed that SAE stimulates the macrophage activation through the upregulation of MAPK and NF-κB cascades.
Fig. 4. Effect of SAE on the signaling pathways involved in macrophage activation.
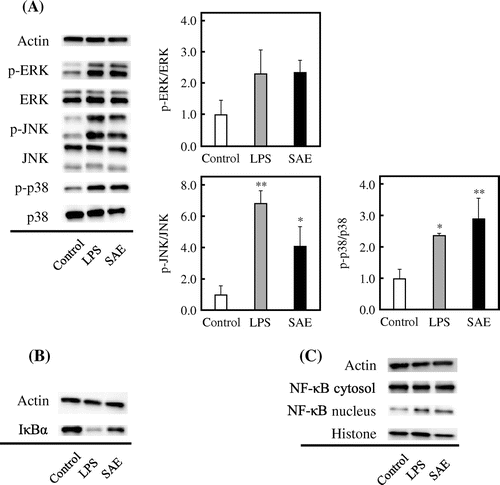
Effect of SAE on TLR4
It is known that macrophages recognize the molecular structures through the PRRs such as TLRs on the cell surface, thereby activating MAPK and NF-κB cascades. Thus, we examined the effect of SAE on TLR4 using TAK-242, which is a small molecule inhibitor that selectively inhibits TLR4 signaling.Citation20,21) J774.1 cells were treated with 0.2 μM TAK-242 for 1 h. The cells were subsequently treated with SAE for 6 h after washing with PBS, and then the cytokine concentration in culture medium was measured by ELISA. As shown in Fig. (A), the IL-6 production induced by LPS or SAE was completely inhibited by the treatment of TAK-242. On the other hand, the inhibition rate of the TNF-α production-inducing activity of SAE was around 40%, whereas that of LPS was 70% (p < 0.01 vs. LPS with treatment of TAK-242). These results suggested that SAE stimulates the cytokine production by acting not only on TLR4, but also on other receptors. Furthermore, we examined the effect of TAK-242 on phagocytosis activity of J774.1 cells. As shown in Fig. (B), the TLR4 inhibition by TAK-242 did not affect the SAE-enhanced phagocytosis activity of J774.1 cells. This result suggested that SAE stimulates the phagocytosis activity by acting on factors other than TLR4.
Fig. 5. Effect of SAE on TLR4 in J774.1 cells.
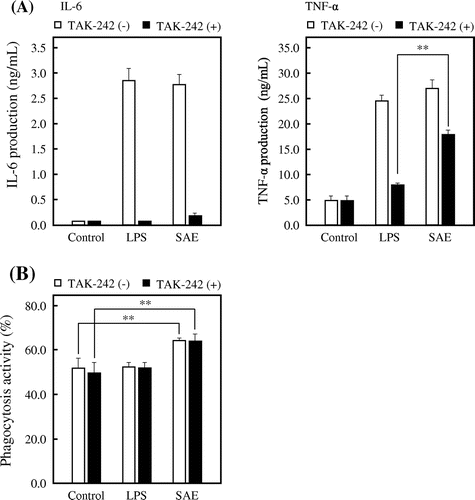
Properties of active substances in SAE
To investigate the properties of the active substance, SAE was heated at each temperature (40, 60, 80, and 100 °C) for 15 min. The heat-treated SAE was then added to the culture medium of J774.1 cells, and the cytokine concentration was measured by ELISA. As shown in Fig. (A), the cytokine production-inducing activity was significantly decreased with the SAE heated at 100 °C, although there was no difference in the cytokine production between the heated SAE at or lower than 80 °C and non-heated SAE. These results suggested that the active substance in SAE is unstable to heat. Next, SAE was treated with 500 μg/mL of trypsin or proteinase K at 37 °C for 15 h. As shown in Fig. (B), the activity on cytokine production was significantly increased by the proteinase K-treated SAE, whereas the trypsin treatment did not affect the cytokine production-inducing activity of SAE. In particular, there was a twofold difference between the non-treated SAE and the proteinase K-treated SAE in the production of IL-6, indicating that the active substance in SAE is a proteinaceous substance.
Fig. 6. Effects of heat- and proteolytic enzyme-treated SAE on cytokine production by J774.1 cells.
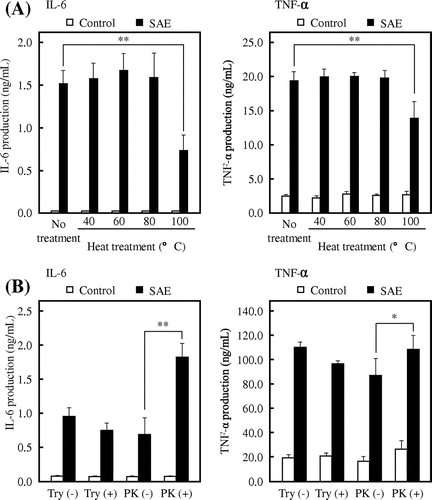
Effect of oral administration of SAE on peritoneal macrophages
Finally, we investigated the effect of oral administration of SAE. Mice were orally administered with 10 mM NaPB for the control group, SAE at 25 mg dry weight/kg body weight/day for high dose group, or 5 mg dry weight/kg body weight/day for low dose group for consecutive 14 days from day 0 to day 13. On day 10, the mice were injected with 3.0% thioglycollate medium in the peritoneum. On day 14, the mice were sacrificed and peritoneal macrophages were collected and cultured for 24 h to evaluate the cytokine production. As shown in Fig. , the oral administration of SAE significantly enhanced the production of IL-6 in the low dose group (p < 0.05 vs. control), whereas SAE did not affect the production of TNF-α. These results suggest that SAE has a potential to stimulate macrophage activity in vivo.
Fig. 7. Effect of oral administration with SAE on peritoneal macrophages.
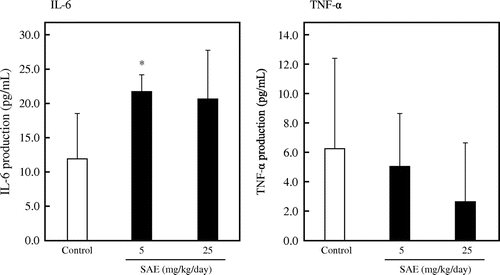
Discussion
In this study, we found that SAE enhances the cytokine production by mouse macrophage-like J774.1 cells and mouse peritoneal macrophages (Fig. ). In addition, the phagocytosis activity of J774.1 cells was enhanced by SAE (Fig. ). Since it has been suggested that the macrophages are activated by SAE, we examined the mechanism of action underlying the macrophage activation by SAE. The MAPK and NF-κB cascades play essential roles in macrophage activation. As seen in the results of immunoblotting (Fig. (A)), SAE increased the phosphorylation levels of ERK, JNK, and p38 in J774.1 cells. In various stimuli, MAPKs are phosphorylated by specific MAPK kinases (MAPKKs), which are activated by specific MAPKK kinases (MAPKKKs), and the phosphorylated MAPKs translocate into the nucleus and activate the specific transcription factor.Citation22,23) There are mainly three families of MAPKs in mammals; ERK, JNK, and p38 kinases.Citation24) The ERK1/2 is activated by MEK1/2 of MAPKK, and the activated ERK1/2 phosphorylates the transcription factor Elk-1 bound to the c-fos promoter and causes the synthesis of c-Fos protein.Citation22,25,26) The JNK activated by MKK4 and MKK7 of MAPKK binds to and phosphorylates the c-Jun and activating transcription factor 2 (ATF2) bound to the c-jun promoter, thereby leading to synthesis of c-Jun protein.Citation23,26–28) The synthesized c-Fos and c-Jun proteins (or ATF2) form the activator protein (AP)-1 dimers, which bind to AP-1-binding site on DNA and promote the transcriptional activity of target genes.Citation22,29,30) The p38 is activated by many of the same extracellular stimuli that activate the JNKs. Although p38 does not phosphorylate or activate c-Jun, it can also contribute to AP-1 activity.Citation26) The p38 is phosphorylated by MKK3 and MKK6 of MAPKK, and the phosphorylated p38 causes the activation of Elk-1, ATF2, or cyclic AMP responsive element-binding protein (CREB), contributing to c-fos and c-jun expression.Citation25,26) For that, it is thought that SAE enhances the expression levels of cytokine genes by activating AP-1 activity through the upregulated phosphorylation of MAPKs.
In addition, SAE enhanced the degradation of IκBα and the translocation of NF-κB into the nucleus in J774.1 cells (Fig. (B) and (C)). NF-κB is a transcription factor that mediates signal transduction between the cytoplasm and nucleus in many cell types, and the NF-κB family consists of five members in mammals: NF-κB1 (p50 and its precursor p105), NF-κB2 (p52 and its precursor p100), RelA (p65), RelB, and Rel (c-Rel).Citation31) It is known that the NF-κB dimers consisting of p50-RelA are associated with increased transcription of genes encoding chemokines, cytokines, and adhesion molecules that are important components of the innate and adaptive immune responses.Citation32,33) The NF-κB dimers are initially located in the cytoplasm in an inactive form bound to IκBα, which acts as an inhibitor of NF-κB. In the classical NF-κB signaling pathway, the stimuli such as LPS cause the activation of IκB kinase (IKK) complex, which consists of the IKKα and IKKβ catalytic subunits and the IKKγ regulatory subunit (called NF-κB essential modulator; NEMO).Citation1) The activated IKK complex phosphorylates IκBα, and the phosphorylated IκBα rapidly undergoes degradation by the ubiquitin-proteasome system. The released NF-κB dimers translocate into the nucleus, bind to the κB motif sequences found in promoter and enhancer regions of genes, and promote the expression of proinflammatory cytokine genes.Citation34) For that, it is suggested that SAE promotes the cytokine gene expression by inducing the translocation of NF-κB into the nucleus through the upregulated phosphorylation and degradation of IκBα. Furthermore, it has been reported that specific p38 and NF-κB inhibitors impair the TLR-induced phagocytosis activity of macrophages.Citation35,36) These reports suggest that the activation of MAPK and NF-κB cascades is involved in TLR-induced phagocytosis activity of macrophages. Therefore, it is suggested that the activation of MAPK and NF-κB cascades by SAE leads to the enhanced phagocytosis activity of macrophages.
The results obtained in this study suggest that the active substance in SAE is estimated to be water-soluble substance and unable to pass through cell membrane to exhibit its activity inside the cells. Therefore, it is likely that SAE acts on the cell surface receptors such as TLRs and involves the macrophage activation. There are various PRRs in macrophages, which initially sense the presence of pathogens.Citation1,2) The PRR families include transmembrane proteins such as TLRs and C-type lectin receptors (CLRs), as well as cytoplasmic proteins such as the retinoic acid-inducible gene (RIG)-like receptors (RLRs) and NOD-like receptors (NLRs).Citation1,2) Especially, the TLR family is a typical PRR in macrophages that recognizes bacteria, viruses, and fungi, and plays a crucial role in the innate and adaptive immune responses; TLR2 recognizes the lipoproteins of bacteria and fungi such as zymosan by forming a heterodimer with either TLR1 or TLR6, whereas TLR4 recognizes LPS together with myeloid differentiation factor 2 (MD2) on the cell surface.Citation2,37,38) The TLR signal transduction is initiated by the recruitment of one or more adaptor proteins such as myeloid differentiation primary response protein 88 (MyD88), Toll/IL-1R homology (TIR) domain-containing adaptor protein (TIRAP), TIR domain-containing adaptor protein inducing interferon β (TRIF), and TRIF-related adaptor molecule (TRIM).Citation1,39) The MyD88 functions as a downstream signaling of most TLRs, excepting TLR3, and leads to the activation of MAPK and NF-κB cascades, resulting in the macrophage activation.Citation1,2) In this study, we used TAK-242, a TLR4 inhibitor, in order to examine an effect of SAE on TLR4. As shown in Fig. (A), the IL-6 production induced by SAE was completely inhibited by the treatment of TAK-242, whereas the inhibition on the TNF-α production-inducing activity of SAE was weaker than that of LPS. In addition, the treatment of TAK-242 showed no effect on the SAE-enhanced phagocytosis activity of J774.1 cells (Fig. (B)). These results suggested that SAE stimulates macrophage activation by acting not only on TLR4, but also on other receptors that have not yet been elucidated. Meanwhile, SAE treated with the proteolytic enzyme showed a different response to the productions of IL-6 and TNF-α (Fig. (B)). It is supposed from this result that SAE includes one or more active substances or activates different signaling pathway except for TLR4 to activate the productions of IL-6 and TNF-α.
The properties of active substance in SAE were investigated. Because SAE was extracted with 10 mM NaPB, the active substance in SAE was considered to be water-soluble. In addition, the cytokine production by J774.1 cells was increased by dialysis of SAE using a dialysis membrane with MWCO of 14,000 compared to the non-dialyzed spinach extract (data not shown). Phytochemicals, which are natural chemicals such as carotenoids and chlorophylls contained in plants, are abundant in spinach. Lutein is a non-provitamin A carotenoid primarily found in dark-green leafy vegetables such as spinach and its immunostimulatory and anti-inflammatory effects on macrophages have been reported.Citation7,40) However, many of the phytochemicals are lipid-soluble substances with low molecular weight. Thus, it is suggested that the molecular weight of the active substance in SAE is equal to or higher than 14,000 and the substances with low molecular weight, such as phytochemicals including lutein, would not be the active substances in SAE. In recent years, the immunostimulatory effect of various polysaccharides from plants on macrophages has been reported.Citation41–44) However, as shown in Fig. (A), the active substance in SAE is unstable to heating at 100 °C. It is well known that LPS stimulates the cytokine production via TLR4 on the cell surface of macrophages.Citation18,19) The cytokine production-inducing activity of LPS was not inactivated by heating at 100 °C (data not shown). In addition, the activity of SAE significantly remained compared to LPS under TAK-242 treatment condition, although the TNF-α production induced by LPS almost disappeared by the treatment of TAK-242 (Fig. ). From these results, the cytokine production-inducing activity of SAE is estimated not to be due to contamination of LPS. As shown in Fig. (B), the cytokine production-inducing activity of SAE increased by fragmenting SAE with proteolytic enzymes. This result suggests that the active substance is a protein which shows strong activity by fragmenting them into peptides with proteolytic enzymes.
Finally, we investigated the effect of oral administration of SAE. As shown in Fig. , the oral administration of SAE significantly enhanced the production of IL-6 in the low dose group. However, there was no difference in the production of TNF-α among each group. The result shown in Fig. suggests that SAE is more effective to the production of IL-6 than that of TNF-α by J774.1 cells. In addition, from Fig. (B), it has been suggested that the production of IL-6 by J774.1 cells is increased by digesting SAE with proteolytic enzymes. For these reasons, it is thought that the remarkable effect on the production of IL-6 in ex vivo experiment might be due to the fragmentation of SAE by protease digestion. This result suggested that the ingestion of SAE or spinach may be effective for enhancing the immune system in humans.
In conclusion, SAE significantly enhanced the production of IL-6 and TNF-α by both J774.1 cells and peritoneal macrophages by enhancing the expression levels of these cytokine genes. In addition, the phagocytosis activity of J774.1 cells was facilitated by SAE. Immunoblot analysis revealed that SAE has the immunostimulatory effect on macrophages through the activation of MAPK and NF-κB cascades. Furthermore, it was found that SAE activates macrophages through not only TLR4, but other receptors. The active substance in SAE was expected to be a proteinaceous substance with molecular weight equal to or higher than 14,000. Finally, the production of IL-6 was significantly enhanced by peritoneal macrophages from SAE-administered BALB/c mice, suggesting that SAE has a potential to stimulate macrophage activity in vivo. Taken together, these data indicated that SAE would be a beneficial functional food with immunostimulatory effects on macrophages.
Author contributions
M. Ishida, S. Ose, K. Nishi, and T. Sugahara conceived and designed the experiments. M. Ishida and S. Ose performed the experiments and analyzed the data. M. Ishida, S. Ose, K. Nishi, and T. Sugahara wrote the manuscript.
Notes
Abbreviations: AP-1, activator protein-1; BSA, bovine serum albumin; ELISA, enzyme-linked immunosorbent assay; ERK, extracellular signal-regulated protein kinase; FBS, fetal bovine serum; IL-6, interleukin-6; JNK, c-Jun N-terminal kinase; LPS, lipopolysaccharide; MAPK, mitogen-activated protein kinase; MWCO, molecular weight cut off; NAPB, sodium phosphate buffer; NF-κB, nuclear factor-κB; PBS, phosphate-buffered saline; RPMI-1640, Roswell Park Memorial Institute Medium-1640; SAE, spinach aqueous extract; TLR, Toll-like receptor; TNF-α, tumor necrosis factor-α.
References
- Takeuchi O, Akira S. Pattern recognition receptors and inflammation. Cell. 2010;140:805–820.10.1016/j.cell.2010.01.022
- Aderem A, Ulevitch RJ. Toll-like receptors in the induction of the innate immune response. Nature. 2000;406:782–787.10.1038/35021228
- Ma J, Chen T, Mandelin J, et al. Regulation of macrophage activation. Cell. Mol. Life Sci. 2003;60:2334–2346.10.1007/s00018-003-3020-0
- Pu Z, Carrero JA, Unanue ER. Distinct recognition by two subsets of T cells of an MHC class II-peptide complex. Proc. Natl. Acad. Sci. USA. 2002;99:8844–8849.10.1073/pnas.092260499
- Riso P, Brusamolino A, Scalfi L, et al. Bioavailability of carotenoids from spinach and tomatoes. Nutr. Metab. Cardiovasc. Dis. 2004;14:150–156.10.1016/S0939-4753(04)80035-8
- Johnson EJ. The role of lutein in disease prevention. Nutr. Clin. Care. 2000;3:289–296.10.1046/j.1523-5408.2000.00075.x
- Lo H-M, Chen C-L, Yang C-M, et al. The carotenoid lutein enhances matrix metalloproteinase-9 production and phagocytosis through intracellular ROS generation and ERK1/2, p38 MAPK, and RARβ activation in murine macrophages. J. Leukoc. Biol. 2013;93:723–735.10.1189/jlb.0512238
- Maeda N, Hada T, Murakami-Nakai C, et al. Effects of DNA polymerase inhibitory and antitumor activities of lipase-hydrolyzed glycolipid fractions from spinach. J. Nutr. Biochem. 2005;16:121–128.10.1016/j.jnutbio.2004.08.005
- Kuriyama I, Musumi K, Yonezawa Y, et al. Inhibitory effects of glycolipids fraction from spinach on mammalian DNA polymerase activity and human cancer cell proliferation. J. Nutr. Biochem. 2005;16:594–601.10.1016/j.jnutbio.2005.02.007
- Heo J-C, Park C-H, Lee H-J, et al. Amelioration of asthmatic inflammation by an aqueous extract of Spinacia oleracea Linn. Int. J. Mol. Med. 2010;25:409–414.
- Ishida M, Nishi K, Watanabe H, et al. Inhibitory effect of aqueous spinach extract on degranulation of RBL-2H3 cells. Food Chem. 2013;136:322–327.10.1016/j.foodchem.2012.08.079
- Putra ABN, Morishige H, Nishimoto S, et al. Effect of collagens from jellyfish and bovine Achilles tendon on the activity of J774.1 and mouse peritoneal macrophage cells. J. Funct. Foods. 2012;4:504–512.10.1016/j.jff.2012.02.011
- Ding AH, Nathan CF, Stuehr DJ. Release of reactive nitrogen intermediates and reactive oxygen intermediates from mouse peritoneal macrophages. Comparison of activating cytokines and evidence for independent production. J. Immunol. 1988;141:2407–2412.
- Vodovotz Y, Bogdan C, Paik J, et al. Mechanisms of suppression of macrophage nitric oxide release by transforming growth factor beta. J. Exp. Med. 1993;178:605–613.10.1084/jem.178.2.605
- Schindler H, Lutz MB, Röllinghoff M, et al. The production of IFN-γ by IL-12/IL-18-activated macrophages requires STAT4 signaling and is inhibited by IL-4. J. Immunol. 2001;166:3075–3082.10.4049/jimmunol.166.5.3075
- Misharin AV, Saber R, Perlman H. Eosinophil contamination of thioglycollate-elicited peritoneal macrophage cultures skews the functional readouts of in vitro assays. J. Leukoc. Biol. 2012;92:325–331.10.1189/jlb.1111560
- Kumalasari ID, Nishi K, Harmayani E, et al. Effect of bengkoang (Pachyrhizus erosus) fiber extract on murine macrophage-like J774.1 cells and mouse peritoneal macrophages. J. Funct. Foods. 2013;5:582–589.10.1016/j.jff.2012.12.005
- Sanghera JS, Weinstein SL, Aluwalia M, et al. Activation of multiple proline-directed kinases by bacterial lipopolysaccharide in murine macrophages. J. Immunol. 1996;156:4457–4465.
- Hambleton J, Weinstein SL, Lem L, et al. Activation of c-Jun N-terminal kinase in bacterial lipopolysaccharide-stimulated macrophages. Proc. Natl. Acad. Sci. USA. 1996;93:2774–2778.10.1073/pnas.93.7.2774
- Ii M, Matsunaga N, Hazeki K, et al. A novel cyclohexene derivative, ethyl (6R)-6-[N-(2-chloro-4-fluorophenyl)sulfamoyl]cyclohex-1-ene-1-carboxylate (TAK-242), selectively inhibits toll-like receptor 4-mediated cytokine production through suppression of intracellular signaling. Mol. Pharmacol. 2006;69:1288–1295.10.1124/mol.105.019695
- Matsunaga N, Tsuchimori N, Matsumoto T, et al. TAK-242 (resatorvid), a small-molecule inhibitor of toll-like receptor (TLR) 4 signaling, binds selectively to TLR4 and interferes with interactions between TLR4 and its adaptor molecules. Mol. Pharmacol. 2011;79:34–41.10.1124/mol.110.068064
- Su B, Karin M. Mitogen-activated protein kinase cascades and regulation of gene expression. Curr. Opin. Immunol. 1996;8:402–411.10.1016/S0952-7915(96)80131-2
- Johnson GL, Lapadat R. Mitogen-activated protein kinase pathways mediated by ERK, JNK, and p38 protein kinases. Science. 2002;298:1911–1912.10.1126/science.1072682
- Chang L, Karin M. Mammalian MAP kinase signalling cascades. Nature. 2001;410:37–40.10.1038/35065000
- Zhang Y, Dong C. MAP kinases in immune responses. Cell. Mol. Immunol. 2005;2:20–27.
- Minden A, Karin M. Regulation and function of the JNK subgroup of MAP kinases. Biochim. Biophys. Acta. 1997;1333:F85–F104.
- Minden A, Lin A, Smeal T, et al. c-Jun N-terminal phosphorylation correlates with activation of the JNK subgroup but not the ERK subgroup of mitogen-activated protein kinases. Mol. Cell. Biol. 1994;14:6683–6688.10.1128/MCB.14.10.6683
- Weston CR, Davis RJ. The JNK signal transduction pathway. Curr. Opin. Genet. Dev. 2002;12:14–21.10.1016/S0959-437X(01)00258-1
- Karin M. The regulation of AP-1 activity by mitogen-activated protein kinases. J. Biol. Chem. 1995;270:16483–16486.10.1074/jbc.270.28.16483
- Karin M, Liu Z-G, Zandi E. AP-1 function and regulation. Curr. Opin. Cell Biol. 1997;9:240–246.10.1016/S0955-0674(97)80068-3
- Ghosh S, Karin M. Missing pieces in the NF-κB puzzle. Cell. 2002;109:S81–S96.10.1016/S0092-8674(02)00703-1
- Collart MA, Baeuerle P, Vassalli P. Regulation of tumor necrosis factor alpha transcription in macrophages: involvement of four kappa B-like motifs and of constitutive and inducible forms of NF-kappa B. Mol. Cell. Biol. 1990;10:1498–1506.10.1128/MCB.10.4.1498
- Bonizzi G, Karin M. The two NF-κB activation pathways and their role in innate and adaptive immunity. Trends Immunol. 2004;25:280–288.10.1016/j.it.2004.03.008
- Matthews JR, Hay RT. Regulation of the DNA binding activity of NF-κB. Int. J. Biochem. Cell Biol. 1995;27:865–879.10.1016/1357-2725(95)00071-V
- Blander JM, Medzhitov R. Regulation of phagosome maturation by signals from toll-like receptors. Science. 2004;304:1014–1018.10.1126/science.1096158
- Suzuki E, Umezawa K. Inhibition of macrophage activation and phagocytosis by a novel NF-κB inhibitor, dehydroxymethylepoxyquinomicin. Biomed. Pharmacother. 2006;60:578–586.10.1016/j.biopha.2006.07.089
- Beutler BA. TLRs and innate immunity. Blood. 2009;113:1399–1407.
- Pasare C, Medzhitov R. Toll-like receptors: linking innate and adaptive immunity. Microbes Infect. 2004;6:1382–1387.10.1016/j.micinf.2004.08.018
- Barton GM, Kagan JC. A cell biological view of Toll-like receptor function: regulation through compartmentalization. Nat. Rev. Immunol. 2009;9:535–542.10.1038/nri2587
- Rafi MM, Shafaie Y. Dietary lutein modulates inducible nitric oxide synthase (iNOS) gene and protein expression in mouse macrophage cells (RAW 264.7). Mol. Nutr. Food Res. 2007;51:333–340.10.1002/(ISSN)1613-4133
- Kim A-J, Kim Y-O, Shim J-S, et al. Immunostimulating activity of crude polysaccharide extract isolated from Curcuma xanthorrhiza Roxb. Biosci. Biotechnol. Biochem. 2007;71:1428–1438.10.1271/bbb.60241
- Lei W, Browning JD Jr, Eichen PA, et al. Immuno-stimulatory activity of a polysaccharide-enriched fraction of Sutherlandia frutescens occurs by the toll-like receptor-4 signaling pathway. J. Ethnopharmacol. 2015;172:247–253.10.1016/j.jep.2015.06.013
- Xie X, Zou G, Li C. Antitumor and immunomodulatory activities of a water-soluble polysaccharide from Chaenomeles speciosa. Carbohydr. Polym. 2015;132:323–329.10.1016/j.carbpol.2015.06.046
- Lee S-G, Jung J-Y, Shin J-S, et al. Immunostimulatory polysaccharide isolated from the leaves of Diospyros kaki Thumb modulate macrophage via TLR2. Int. J. Biol. Macromol. 2015;79:971–982.10.1016/j.ijbiomac.2015.06.023