Abstract
Obesity is documented to be a state of chronic mild inflammation associated with increased macrophage infiltration into adipose tissue and liver and skeletal muscle. As a pleiotropic inflammatory mediator, macrophage migration inhibitory factor (MIF) is associated with metabolic disease, so MIF may signal molecular links between adipocytes and myocytes. MIF expression was modified during myoblast differentiation, but the role of MIF during this process is unclear. C2C12 cells were transfected with MIF to investigate their role during differentiation. MIF expression attenuated C2C12 differentiation. It did not change proliferation, but downregulated cyclin D1 and CDK4, causing cell accumulation in the G1 phase. p21 protein was increased significantly and MyoD, MyoG, and p21 mRNA also increased significantly in the C2C12 cells treated with ISO-1, suggesting that inhibition of MIF promotes differentiation. MIF inhibits the myoblast differentiation by affecting the cell cycle progression, but does not affect proliferation.
Graphical abstract
Effects of MIF on C2C12 cell proliferation and the cell cycle showed that MIF inhibits myogenic differentiation via interference with cell cycle progression.
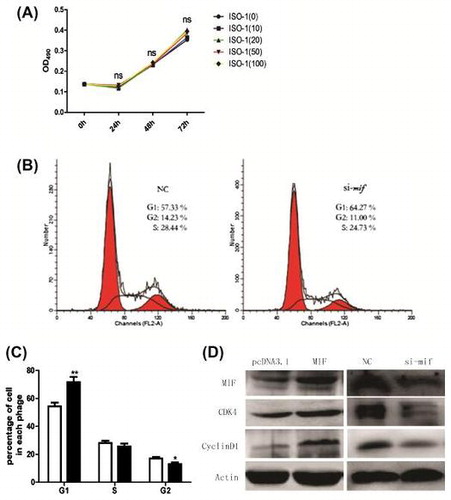
Obesity is a global health problem characterized by a state of chronic mild inflammation,Citation1) with elevated circulating of inflammatory markers such as TNFα, IL-6, CRP, PAI-1 MCP-1, and expression and release of inflammation-related adipokines: resistin, adiponectin, leptin, and visfatin.Citation2) Adiponectin which is thought to be anti-inflammatory,Citation3) is reduced in obesity.Citation4) Obesity is associated with increased macrophage infiltration into adipose tissueCitation5) and macrophages, and can comprise up to 40% of the cell’s population within an adipose tissue depot.Citation5,6) Accumulating evidence indicates that macrophages are a potential source of proinflammatory factorsCitation7) suggesting that macrophages can directly influence adipocyte biology and metabolic syndrome.Citation6)
Macrophage migration inhibitory factor (MIF) is one of the first proinflammatory cytokines described,Citation8,9) cloned, purified, and characterized at the molecular level.Citation10) As a pleiotropic inflammatory mediator, MIF is produced by various cell types such as monocytes/macrophages, B- and T-cells, and endothelial and epithelial cells.Citation11) MIF was first reported to inhibit macrophage migration in vitroCitation9,12) so was named for this activity. Increasing evidence suggests that MIF is involved in various acute and chronic inflammatory diseases,Citation11) such as obesity and diabetes.Citation13)
In obesity, proinflammatory macrophages are increased in skeletal muscle.Citation14–17) Mouse and human skeletal muscles have a distinct population of inflammatory macrophages with obesity, and these are linked to insulin resistance in these models.Citation18) However, the role of MIF during myogenic cell differentiation is uncertain, so we studied MIF’s effects on differentiation of C2C12 myogenic cells.
Materials and methods
Cell culture
C2C12 (ATCC) myoblasts were maintained in DMEM medium (HyClone, Thermo Scientific, Logan, Utah) supplemented with 10% fetal bovine serum (FBS) (Tianhang, Zhejiang, China), 100 U/mL penicillin, 100 μg/mL streptomycin, and cultured at 37 °C in a humidified chamber containing 5% CO2. For C2C12 cell differentiation experiments, the medium was replaced at 80% confluence (day 0) with differentiation medium (DM:DMEM supplemented with 2% heat-inactivated horse serum).
Plasmids and transfection
Expression vectors for MIF (pcDNA3.1-mif) were constructed by inserting the products of reverse transcription and PCR obtained from C2C12 RNA into pcDNA3.1. Inhibition of MIF was carried on by two methods, siRNA of MIF (IBS Bio, Shanghai, China) and ISO-1 (Sigma). Unless otherwise indicated, all experiments were performed in triplicate in three independent experiments. Vectors and siRNAs were transfected into cells in serum-free medium; media was changed to DMEM/DM 6 h later. Cells were harvested 24 h after transfection to isolate RNA or protein.
RNA isolation
Total RNA was extracted using Tiangen miRcute miRNA isolation kit following the manufacturer’s instructions. RNA quality was assessed using UV–Vis spectrophotometry (SMA4000). RNA was generally of high quality (average 260/280 ratio of 2.02, and an average 260/230 ratio of 1.79).
Real-time PCR (qRT-PCR)
Total RNA extracted from cells was reverse transcribed into cDNA using M-MLV reverse transcriptase (Promega) with oligo(dT)18 primers. The sequences of the primers used are listed in Table . Real-time PCR was performed using SYBR Green PCR Mix (ABI) with CFX96TM real timer PCR system (Bio-Rad). PCR cycles were as follows: initial denaturation at 95 °C for 5 min, followed by 35 cycles of 95 °C for 15 s, and 60 °C for 30 s. The average threshold (Ct) was determined for each gene, and normalized to GAPDH as internal normalization controls. Fold changes in relative expression were calculated using the 2-ΔΔCt method.
Table 1. The primers for real-time PCR.
Western blot
Cells were harvested and lysed in RIPA lysis buffer (Beyotime, P0013C) with or without phosSTOP phosphatase inhibitor cocktail tablets (Roche Applied Science city, state). Protein concentrations were determined using a BCA kit (Beyotime, Shanghai, China). Protein samples were separated using 10 or 12% SDS-PAGE, and then transferred to PVDF membranes (Bio-Rad). Membranes were blocked with 5% non-fat dry milk in TBS-Tween. Target proteins were measured with specific polyclonal antibodies; GAPDH or β-actin served as an internal control. Antibodies against MIF, cyclin D1 (Santa Cruz), MHC (Millipore), CDK4 (Bioworld), and p21 (ProteinTech) were used. Equivalent protein loads were verified and normalized using β-actin (Santa Cruz).
Immunofluorescence
C2C12 cells on glass coverslips were fixed in 4% paraformaldehyde for 30 min and washed three times with phosphate-buffered saline (PBS). Cells were treated with a blocking solution (PBS with 3% BSA and 0.2% Triton X-100) for 1 h before incubation with antibodies. Primary monoclonal anti-myosin heavy chain antibody (anti-MHC, 05-716, Millipore, city, state) and the secondary rabbit anti-mouse IgG antibody conjugated to fluorescein isothiocyanate (FITC, Sigma) were used. Nuclei were stained with 4’,6’diamidino-2-phenylindole (DAPI, Sigma), and images were obtained using fluorescent microscopy. Myoblast morphological differentiation images were acquired using phase contrast microscopy.
Cell proliferation analysis
Cells were grown in 96-well microtiter plates and incubated overnight in 100 μL of the culture medium. Cells were starved without FBS for 24 h at 70–80% confluence and then treated with siRNA of MIF(si-mif) at different concentrations (0, 10, 20, 50, and 100 μM) for 0, 24, 48, and 72 h, respectively. Cells without any treatment were used as controls. Twenty microliters of 5-mg/mL MTT (Sigma) labeling reagent were added to the designated well, and the cells were incubated at 37 °C for 4 h. Supernatant was removed, and then 150-μL dimethylsulfoxide was added to the designated well. After the plate was incubated at 37 °C for 15 min, absorbency was measured with a micro ELISA reader (Bio-Tek, Winooski, VT) at a wavelength of 570 nm.
Flow cytometry
Cells were harvested after treatment with si-mif (50 μM) for 24 h as described, and fixed with 75% cold alcohol at 4 °C overnight. After washing with PBS, propidium iodide was added and cells were incubated at 4 °C for 30 min. Cell cycle distribution was assessed with an FACSCalibur flow cytometer (BD Bioscience, Franklin Lakes, NJ) and analyzed with Mod-Fit software.
Statistical analysis
Data are presented as means ± standard deviation (SD). Statistical analyses were performed using unpaired two-tailed t-test (for two groups) and analysis of variance (ANOVA; for multiple groups). P-values < 0.05 were considered statistically significant.
Results
MIF is downregulated during myoblast differentiation
To explore the biological functions of MIF in myoblast differentiation, C2C12 myoblasts were differentiated with differentiation medium (DM) (Fig. (A), and then expression of muscle differentiation specific markers and MIF was measured by qPCR and Western blot. Myogenic marker gene myosin heavy chain (MHC), MyoG, p2, and MyoD mRNA expression was significantly upregulated, and myf5 was downregulated during C2C12 differentiation (Fig. (B). MIF expression was downregulated during early differentiation, but was upregulated at a later period during myogenesis (Fig. (B). MHC protein was elevated and MIF protein was consistent with mRNA (Fig. (C). It suggests that MIF may be critical for the function of differentiated myoblasts.
Fig. 1. MIF expression is changed during myoblast differentiation.
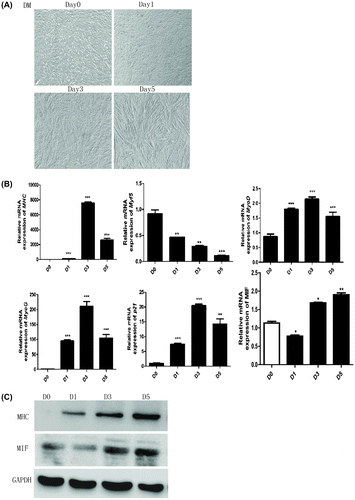
MIF overexpression inhibited myogenesis
Because expression was changed during myoblast differentiation, MIF plasmid was established to assess the role of MIF in skeletal myogenesis in C2C12 myoblasts, and myoblast differentiation was induced by DM. Typical morphological changes associated with myoblast differentiation, such as cell elongation, cell fusion, and myotube formation were observed in MIF-overexpressing and control myoblasts. However, myotube formation was observed in both cell lines after induction of MHC expression, but myotubes were fewer in MIF-overexpressing myoblasts (Fig. (A). MHC protein was consistent with the immunofluorescent results, lower in MIF-overexpressing than control myoblasts (Fig. (B). Thus, MIF played was important for myoblast differentiation and myotube formation.
Fig. 2. Overexpression of MIF inhibits C2C12 myogenesis.
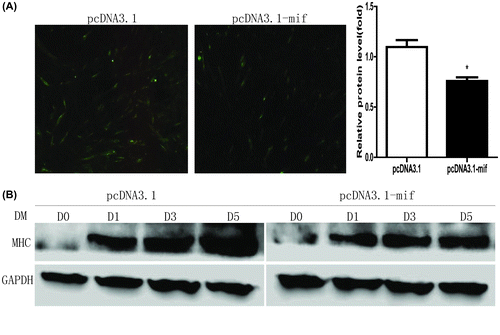
MIF is involved in cell cycle regulation
Recent studies show that MIF can modeulate cell proliferationCitation19) and is relevant to cell cycle factors.Citation20) Cell cycle arrest is critical for muscle differentiation and the two processes are closely coordinated. To clarify how MIF inhibits myogenesis, ISO-1 (an inhibitor of MIF) was applied to C2C12 cells (0, 10, 20, 50, and 100 μM) for 0, 24, 48, or 72 h, respectively. MTT assay revealed no significant differences between treated and control cells (Fig. (A), indicating that MIF inhibits C2C12 differentiation but not proliferation.
Fig. 3. Effects of ISO-1 and MIF siRNA on C2C12 cell proliferation and the cell cycle.
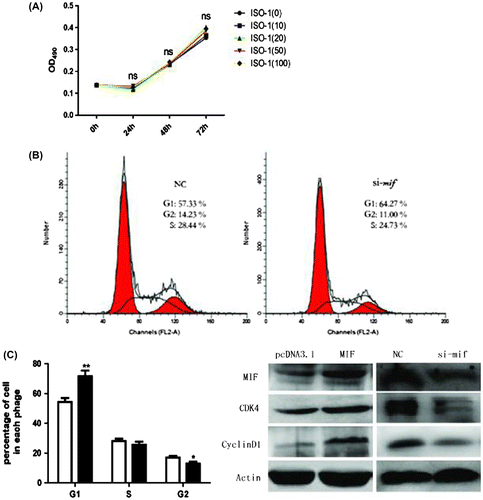
We measured the effect of MIF on C2C12 cell cycles by culturing and transfecting them with MIF siRNA (si-mif), and flow cytometry was used to analyze cell cycle arrest. Si-mif (50 μM) significantly inhibited C2C12 cell progression from G1 to S phase (Fig. (B), increasing cell accumulation in the G1 phase (Fig. (C).
Overexpression of cyclin D1, a cyclin protein which regulates cell cycle progression from the G1 to the S phaseCitation19,21) can lead to the loss of control of cell proliferation and differentiation. Cyclin-dependent kinase 4 (CDK4), also has an important role in the cell cycle regulation.Citation22,23) We measured the effect of MIF on cyclin D1 and CDK4 protein expression in C2C12 cells. After treatment with 50-μM siRNA for 24 h, cyclin D1 and CDK4 proteins decreased significantly (Fig. (D), indicating that inhibition of MIF downregulated cyclin D1 and CDK4, causing cell accumulation in the G1 phase or G1 arrest.
Inhibition of MIF promote myogenesis
In vitro, the skeletal muscle differentiation prerequisite for myogenic differentiation is irreversible withdrawal from the cell cycle.Citation24,25) MyoD is a crucial factor for myogenic differentiation that is key to myoblast proliferation. MyoD also acts as a master switch for skeletal muscle differentiation from myoblast proliferation into differentiation.Citation26) MyoD can promote cell cycle arrest by inducing p21,Citation27,28) which inhibits diverse cyclin-dependent kinases essential for cell cycle progression.Citation29) It is generally accepted that induction of p21 follows myogenin during myoblast differentiation and that abundant p21 is required for cells to maintain a postmitotic state.Citation30) After treatment with si-mif for 24 h, p21 protein was increased significantly (Fig. (A) and (B). MyoD, MyoG, and p21 mRNA also increased significantly (Fig. (A) in C2C12 cells treated with ISO-1, and cell elongation, cell fusion, and myotube formation was greater compared with controls (Fig. (C). This indicates that MIF inhibition promotes differentiation and that MIF inhibits myogenic differentiation via cell cycle progression.
Fig. 4. Inhibition of MIF promotes myogenesis.
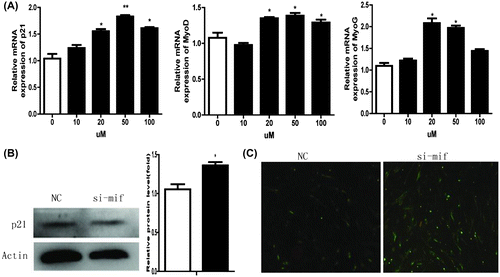
Discussion
Obesity is a worldwide problem characterized by chronic mild inflammationCitation1) and increased macrophages infiltration of adipose tissue.Citation5) MIF deficiency partially protects from high-fat-induced insulin resistance by attenuating macrophage infiltration, ameliorating adipose inflammation,Citation31) which suggests that MIF plays an important role in macrophages infiltration. Macrophages are also increased in skeletal muscle in high fat-fed mice or obese mouse models and humans,Citation18) while the precise mechanisms underpinning the initiation of macrophage recruitment and activation remain unclear. As a key regulator of inflammation, MIF may be a signaling link among macrophages, adipocytes, and myocytes. With a C2C12 cell line, we studied the role of MIF during myoblast proliferation and differentiation, and investigated the molecular mechanisms behind its effect during macrophage infiltration in skeletal muscle.
Crosstalk between myoblast proliferation and differentiation is important during myogenesis. Indeed, the formation of multi-core muscle tubes of C2C12 has two steps: myoblast proliferation and directional differentiation. Leaving the cell cycle irreversibly commits the cell to differentiation and factors affecting proliferation or this cell cycle exit can block differentiation. In this study, we found that MIF overexpression inhibited myoblast differentiation. However, it remains unclear why MIF is not effective at the proliferation. One possible explanation is that the MIF overexpression affects the cell condition at the same time, which is yet to be verified.
Myogenic differentiation is an orderly progression with strict regulation. First, cell cycle exit is essential for myogenic differentiation. Cyclin D1 is an important regulator of cell cycle progressionCitation21) and CDK4 is needed for the orderly progression of the cell cycle from the G1 to the S phase.Citation32) The process that coordinates myoblast proliferation and differentiation requires downregulation of cell cycle activators such as cyclins and CDKs.Citation33) In this study, to elucidate how MIF inhibits the differentiation of C2C12 cells, the cyclin D1 and CDK4 protein level, and also the cell cycle were investigated. MIF inhibition downregulated cyclin D1 and CDK4 protein significantly, and flow cytometry suggested that MIF increased the number of cells in the G1 phase, confirming that MIF inhibits C2C12 differentiation likely via cell cycle arrest. While the mechanism how MIF affects the cyclin D1 and CDK4 expression and how it subsequently affects the cell cycle remain unclear. Using posttranscriptional silencing with small interfering RNAs, we think maybe MIF acts as an autocrine factor involved in cell cycle progression, and we think that detailed future studies will focus on this protein to clarify the importance in MIF-mediated regulation of C2C12 cell differentiation.
Skeletal muscle development is regulated by a family of muscle-regulatory factors including MyoD, myf5, MyoG. MyoD was shown to induce termination of myoblast proliferation and expression of MyoG when myoblasts are induced to differentiate with DM.Citation34–36) Also, coordination of myoblast proliferation and differentiation requires upregulation of cell cycle inhibitors such as p21, p27, and p57.Citation37) We report that p21 protein increased significantly when MIF was inhibited by siRNA, and MyoD, MyoG, and p21 mRNA also increased significantly in C2C12 cells treated with ISO-1. MIF inhibition promoted C2C12 differentiation. Thus, MIF inhibits myogenic differentiation via interference with cell cycle progression. Whether MIF has a role in macrophage infiltration into skeletal muscle in obesity is a future research direction worthy of investigation. In 1966, MIF was initially described as an immune activity and was found to inhibit the random migration of macrophages.Citation12) Therefore, a possible potential regulatory feedback loop existed between the macrophages migration and secretion of MIF, that is, macrophages infiltration was increased in inflammatory status subsequently elevating the circular level of MIF, while elevated MIF in reverse inhibit the macrophages infiltration further, which maybe for a protective mechanism reason.
Funding
This work was supported by the Program of National Natural Science Foundation of China under [grant number 31172093, 31301940]; the National Science Foundation for Fostering Talents in Basic Research under [grant number J1103510]; the Fundamental Research Funds for the Central Universities under [grant number 2013PY005].
Authors contribution
Fengyun Wen and Jin Zheng contributed to research data, discussion, and preparation of the manuscript and is the guarantor of this work and, as such, had full access to all study data. Jing Yu, Mingju Gao, and Sumin Gao contributed to cell experiments. Yingying Zhou and Jianyu Liu contributed to the study design. Address all correspondence and requests for reprints to Zaiqing Yang, Ph.D., professor, College of Life Sciences & Technology, Huazhong Agricultural University, Wuhan, 430070, People’s Republic of China. Email: [email protected].
Disclosure statement
No potential conflict of interest was reported by the authors.
References
- Trayhurn P, Wood IS. Signalling role of adipose tissue: adipokines and inflammation in obesity. Biochem. Soc. Trans. 2005;33:1078–1081.10.1042/BST0331078
- Fantuzzi G. Adipose tissue, adipokines, and inflammation. J. Allergy Clin. Immunol. 2005;115:911–919; quiz 920.10.1016/j.jaci.2005.02.023
- Ouchi N, Kihara S, Arita Y, et al. Adiponectin, an adipocyte-derived plasma protein, inhibits endothelial NF-kappaB signaling through a cAMP-dependent pathway. Circulation. 2000;102:1296–1301.10.1161/01.CIR.102.11.1296
- Arita Y, Kihara S, Ouchi N, et al. Paradoxical decrease of an adipose-specific protein, adiponectin, in obesity. Biochem. Biophys. Res. Commun. 1999;257:79–83.10.1006/bbrc.1999.0255
- Weisberg SP, McCann D, Desai M, et al. Obesity is associated with macrophage accumulation in adipose tissue. J. Clin. Invest. 2003;112:1796–1808.10.1172/JCI200319246
- Xu H, Barnes GT, Yang Q, et al. Chronic inflammation in fat plays a crucial role in the development of obesity-related insulin resistance. J. Clin. Invest. 2003;112:1821–1830.10.1172/JCI200319451
- Hotamisligil GS, Shargill NS, Spiegelman BM. Adipose expression of tumor necrosis factor-alpha: direct role in obesity-linked insulin resistance. Science. 1993;259:87–91.10.1126/science.7678183
- George M, Vaughan JH. In vitro cell migration as a model for delayed hypersensitivity. Proc. Soc. Exp. Biol. Med. 1962;111:514–521.10.3181/00379727-111-27841
- David JR. Delayed hypersensitivity in vitro: its mediation by cell-free substances formed by lymphoid cell-antigen interaction. Proc. Natl. Acad. Sci. USA. 1966;56:72–77.10.1073/pnas.56.1.72
- Bernhagen J, Calandra T, Mitchell RA, et al. MIF is a pituitary-derived cytokine that potentiates lethal endotoxaemia. Nature. 1993;365:756–759.10.1038/365756a0
- Calandra T, Roger T. Macrophage migration inhibitory factor: a regulator of innate immunity. Nat. Rev. Immunol. 2003;3:791–800.10.1038/nri1200
- Bloom BR, Bennett B. Mechanism of a reaction in vitro associated with delayed-type hypersensitivity. Science. 1966;153:80–82.10.1126/science.153.3731.80
- Waeber G, Calandra T, Bonny C, et al. A role for the endocrine and pro-inflammatory mediator MIF in the control of insulin secretion during stress. Diabetes Metab. Res. Rev. 1999;15:47–54.10.1002/(ISSN)1520-7560
- Pincu Y, Linden MA, Zou K, et al. The effects of high fat diet and moderate exercise on TGFβ1 and collagen deposition in mouse skeletal muscle. Cytokine. 2015;73:23–29.10.1016/j.cyto.2015.01.013
- Zhang J, Xiao Z, Qu C, et al. CD8 T cells are involved in skeletal muscle regeneration through facilitating MCP-1 secretion and Gr1(high) macrophage infiltration. J. Immunol. 2014;193:5149–5160.10.4049/jimmunol.1303486
- Patsouris D, Cao JJ, Vial G, et al. Insulin resistance is associated with MCP1-mediated macrophage accumulation in skeletal muscle in mice and humans. PLoS One. 2014;9:e110653.10.1371/journal.pone.0110653
- Boon MR, Bakker LE, Haks MC, et al. Short-term high-fat diet increases macrophage markers in skeletal muscle accompanied by impaired insulin signalling in healthy male subjects. Clin. Sci. (Lond). 2015;128:143–151.10.1042/CS20140179
- Fink LN, Costford SR, Lee YS, et al. Pro-Inflammatory macrophages increase in skeletal muscle of high fat-Fed mice and correlate with metabolic risk markers in humans. Obesity (Silver Spring). 2014;22:747–757.10.1002/oby.v22.3
- Li GQ, Xie J, Lei XY, et al. Macrophage migration inhibitory factor regulates proliferation of gastric cancer cells via the PI3K/Akt pathway. World J. Gastroenterol. 2009;15:5541–5548.10.3748/wjg.15.5541
- Liu L, Ji C, Chen J, et al. A global genomic view of MIF knockdown-mediated cell cycle arrest. Cell Cycle. 2008;7:1678–1692.10.4161/cc.7.11.6011
- Tashiro E, Tsuchiya A, Imoto M. Functions of cyclin D1 as an oncogene and regulation of cyclin D1 expression. Cancer Sci. 2007;98:629–635.10.1111/cas.2007.98.issue-5
- Tam SW, Shay JW, Pagano M. Differential expression and cell cycle regulation of the cyclin-dependent kinase 4 inhibitor p16Ink4. Cancer Res. 1994;54:5816–5820.
- Pines J. The cell cycle kinases. Semin. Cancer Biol. 1994;5:305–313.
- Nadal-Ginard B. Commitment, fusion and biochemical differentiation of a myogenic cell line in the absence of DNA synthesis. Cell. 1978;15:855–864.10.1016/0092-8674(78)90270-2
- Stockdale FE, Holtzer H. DNA synthesis and myogenesis. Exp. Cell Res. 1961;24:508–520.10.1016/0014-4827(61)90450-5
- Puri PL, Balsano C, Burgio VL, et al. MyoD prevents cyclinA/cdk2 containing E2F complexes formation in terminally differentiated myocytes. Oncogene. 1997;14:1171–1184.10.1038/sj.onc.1200941
- Guo K, Wang J, Andres V, et al. MyoD-induced expression of p21 inhibits cyclin-dependent kinase activity upon myocyte terminal differentiation. Mol. Cell Biol. 1995;15:3823–3829.10.1128/MCB.15.7.3823
- Halevy O, Novitch BG, Spicer DB, et al. Correlation of terminal cell cycle arrest of skeletal muscle with induction of p21 by MyoD. Science. 1995;267:1018–1021.10.1126/science.7863327
- Sherr CJ, Roberts JM. CDK inhibitors: positive and negative regulators of G1-phase progression. Genes Dev. 1999;13:1501–1512.10.1101/gad.13.12.1501
- Shen X, Collier JM, Hlaing M, et al. Genome-wide examination of myoblast cell cycle withdrawal during differentiation. Dev. Dyn. 2003;226:128–138.10.1002/(ISSN)1097-0177
- Finucane OM, Reynolds CM, McGillicuddy FC, et al. Macrophage migration inhibitory factor deficiency ameliorates high-fat diet induced insulin resistance in mice with reduced adipose inflammation and hepatic steatosis. PLoS One. 2014;9:e113369.10.1371/journal.pone.0113369
- Santamaria D, Ortega S. Cyclins and CDKS in development and cancer: lessons from genetically modified mice. Front Biosci. 2006;11:1164–1188.10.2741/1871
- De Falco M, De Luca A. Involvement of cdks and cyclins in muscle differentiation. Eur. J. Histochem. 2006;50:19–23.
- Yokoyama S, Asahara H. The myogenic transcriptional network. Cell Mol. Life Sci. 2011;68:1843–1849.10.1007/s00018-011-0629-2
- Berkes CA, Tapscott SJ. MyoD and the transcriptional control of myogenesis. Semin. Cell Dev. Biol. 2005;16:585–595.10.1016/j.semcdb.2005.07.006
- Kitzmann M, Fernandez A. Crosstalk between cell cycle regulators and the myogenic factor MyoD in skeletal myoblasts. Cell Mol. Life Sci. 2001;58:571–579.10.1007/PL00000882
- Zhang P, Wong C, Liu D, et al. p21(CIP1) and p57(KIP2) control muscle differentiation at the myogenin step. Genes Dev. 1999;13:213–224.10.1101/gad.13.2.213