Abstract
The high-affinity IgE Fc receptor (FcεRI) β chain acts as a signal amplifier through the immunoreceptor tyrosine-based activation motif in its C-terminal intracellular region. Polymorphisms in FcεRI β have been linked to atopy, asthma, and allergies. We investigated the secondary structure, conformation, and thermal stability of FcεRI β polymorphic (β-L172I, β-L174V, and β-E228G) proteins. Polymorphisms did not affect the secondary structure and conformation of FcεRI β. However, we calculated Gibbs free energy of unfolding (ΔGunf) and significant differences were observed in ΔGunf values between the wild-type FcεRI β (β-WT) and β-E228G. These results suggested that β-E228G affected the thermal stability of FcεRI β. The role of β-E228G in biological functions and its involvement in allergic reactions have not yet been elucidated in detail; therefore, differences in the thermal stability of β-E228G may affect the function of FcεRI β.
Graphical abstract
Significant differences were observed in ΔGunf values between FcεRI β-WT and β-E228G.
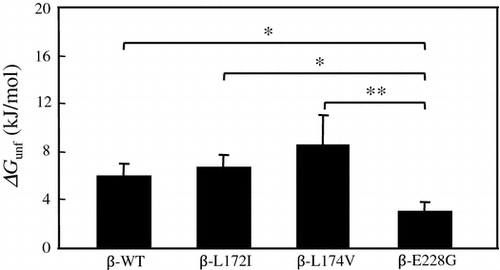
Mast cells express a high-affinity receptor for IgE (FcεRI). FcεRI has a tetrameric structure consisting of three distinct polypeptides including the IgE-binding α chain, fourfold membrane-spanning β chain, and disulfide-linked γ-γ homodimer.Citation1–4) The aggregation of FcεRI by the interaction of bound IgE with multivalent antigens has been shown to induce the release of histamine, leukotrienes, and inflammatory cytokines, and plays an important role in allergic inflammation.Citation5,6) Of these polypeptides, the FcεRI β chain acts as a signal amplifier in mast cell activation.Citation7–9) Furthermore, the β chain was previously reported to amplify early activation signals five–sevenfold through FcεRI in humans.Citation8) The β chain has also been suggested to function as a stabilizer of the FcεRI complex.Citation10) It contains an immunoreceptor tyrosine-based activation motif (ITAM), a conserved feature of many antigen receptors that imparts signaling competence. The ITAM consensus sequence is (D/E)XXYXXLX7–11-YXXL(L/I), in which tyrosine residues are phosphoacceptor sites for the actions of receptor-associated protein tyrosine kinases.Citation11) On the other hand, the β chain ITAM shows a notable departure from the consensus ITAM sequence with the presence of a third tyrosine (the middle tyrosine) between the two canonical tyrosine residues and a shorter spacer region between the canonical tyrosines. A previous study showed that the phosphorylation of these three tyrosines played a very important role in mast cell activation via FcεRI.Citation12)
Several studies on the genetic background of atopy by genome-wide screening for polymorphisms and linkage analyses identified a number of candidate genes likely to contribute to the pathogenesis of allergies.Citation13–15) Of these, a significant role for polymorphisms in the FcεRI β chain in the manifestation of the phenotype has been suggested. Genetic linkage studies demonstrated that a locus in chromosome 11q12-13 encompassing the β chain gene was linked to various allergic disorders and high levels of serum IgE.Citation16–19) Three polymorphisms in the β chain gene, which result in the amino acid changes of Leu181→Ile and Leu183→Val in the fourth transmembrane domain and of Glu237→Gly in the C-terminal intracellular domain, have so far been identified.Citation20–22) A strong relationship was found between these polymorphisms and atopy in various populations.Citation21,23) The human variant (Leu181→Ile, Leu183→Val, Glu237→Gly) is known to correspond to the mouse β chain (Leu172→Ile: β-L172I, Leu174→Val: β-L174V, Glu228→Gly: β-E228G).Citation7) A previous study reported that no significant differences existed in skin prick tests and in histamine release from peripheral basophils among atopic patients with or without the L181I polymorphism.Citation24) An evaluation of the mouse β-E228G or human β-E237G variant has provided conflicting findings as to whether this variant is involved in allergic reactions. In humans, β-E237G may be associated with measures of atopy and asthma.Citation21,25) Kim et al. showed that E237G was associated with a positive rate of airway hyperresponsiveness, but not with either a positive skin prick test or total serum IgE levels.Citation26) On the other hand, Furumoto et al. demonstrated that β-E228G in the FcεRI β chain in mice did not affect FcεRI-mediated mast cell activation, such as β-hexosaminidase release, cytokine production, and leukotriene secretion in vitro.Citation27) Therefore, the role of β-E228G in biological functions and its involvement in allergic reactions have not yet been clarified.
From a structural aspect, previous studies reported that the C-terminal intracellular region of FcεRI β was dominated by an α-helical structure.Citation28,29) FcεRI β of cytoplasmic and extracellular regions without the C-terminal region also has an α-helical structure.Citation30) However, its conformation and thermal stability remain unknown even for the wild type (β-WT) protein. Therefore, we herein examined the secondary structure, conformation, and thermal stability of FcεRI β-WT, β-L172I, β-L174V, and β-E228G proteins.
Materials and methods
Reagents
Taq DNA polymerase, restriction enzymes (EcoRI and BamHI), and isopropyl-β-D-thiogalactopyranoside (IPTG) were purchased from Takara Bio Inc. (Kyoto, Japan). The pGEX-6P-1 vector was from GE Healthcare (Tokyo, Japan). E. coli BL21 (DE3) was from Stratagene (La Jolla, CA). Protein Extraction Reagent, Benzonase nuclease, and rLysozyme solution were from Novagen (Madison, WI). The chemicals used in the present study were of analytical grade.
Construct, bacterial expression, and purification of fusion-proteins
The mouse β chains of the WT, L172I, L174V, and E228G coding sequences in the pMX-puro vectorCitation27) were used as templates. PCR amplification was performed using the plasmid DNAs described above as templates with the following primers and cycling conditions: forward, 5,-CCGGATCCAATCTGACCAATAACTTCGC-3, reverse, 5,-GGGAATTCTTATGAATCAACTGGAGAAG-3, 94 °C for 1 min, 55 °C for 1 min, and 72 °C for 1 min with 35 cycles. The amplified DNAs including the β chain C-terminal intracellular domain, transmembrane domain, and extracellular domain (amino acids 143-235) were digested by BamHI and EcoRI, and were cloned into the pGEX-6P-1 vector downstream of the glutathione S-transferase (GST) gene. The integrities of β-WT, β-L172I, β-L174V, and β-E228G were confirmed by a sequence analysis using a capillary sequencer. E. coli BL21 (DE3) competent cells were transformed with recombinant plasmids and grown in LB medium containing 100 μg/mL ampicillin. After cell density reached 0.4–0.6 OD600 nm, IPTG at a final concentration of 2 mM was added to induce GST-fused β-WT, β-L172I, β-L174V, and β-E228G protein fragments. After a 4-6-h induction at 30 °C cells were collected by centrifugation at 6000 rpm for 10 min, and lysed using Protein Extraction Reagent with Benzonase nuclease and rLysozyme solution. They were subjected to centrifugation at 15,000 rpm for 20 min at 4 °C. The supernatant was rotated for 2 h at 4 °C with glutathione-sepharose beads. Cleavage solution (50 mM Tris-HCl, 150 mM NaCl, 1 mM EDTA, 1 mM DTT, pH 7.5) with Prescission Protease was added and incubated end-over-end overnight at 4 °C, and the supernatant was then collected. They were dialyzed in phosphate-buffered saline solution. The amino acid sequences encoded by the β chain C-terminal intracellular domain, transmembrane domain, and extracellular domain (amino acids 143-235) of β-WT β-L172I, β-L174V, and β-E228G are shown in Fig. . These proteins were used for CD spectroscopy. This study was approved by the Experimental Committee of Gifu University (approval number: 23-2-56).
Fig. 1. Amino acid sequences of β-WT, β-L172I, β-L174V, and β-E228G (amino acids 143-235).
Notes: The ITAM motif is shown as a double-headed arrow. Tyrosines in the ITAM are underlined. The amino acid residue 172 replacement of Leu to Ile, 174 replacement of Leu to Val, and 228 replacement of Glu to Gly are in bold type.
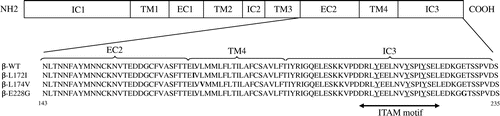
CD measurements
CD spectra were recorded on a Jasco J-820 spectropolarimeter (Jasco Corp. Tokyo, Japan) with a Jasco PTC-423L Peltier-type thermostatic cell holder with an error of approximately 0.2 °C. Regarding protein measurements, far-UV and near-UV CD spectra were recorded at 200–250 and 250–350 nm, respectively, by collecting data at 0.1-nm intervals. Quartz cuvettes with an optical path length of 1 and 10 mm were used for far-UV and near-UV CD measurements, respectively. Each spectrum was the average of eight consecutively acquired spectra. The final concentration of the protein was 0.25 mg/mL for far-UV CD measurements and 0.5 mg/mL for near-UV CD. The buffer spectrum was measured and subtracted from sample spectra.
CD spectra obtained were subjected to 15 point Savitzky-Golay smoothing on Spectra Manager for Windows 95/NT ver 1.5301 software (Jasco Corp. Tokyo, Japan). Ellipticity was reported as the mean residue molar ellipticity ([θ], deg cm2 dmol−1) calculated from
(1)
where [θ]obs is ellipticity measured in degrees, mrw is the mean residue molecular weight, c is the protein concentration (in grams per milliliter), and L is the optical path length of the cell (in centimeters).
In thermal unfolding studies, CD spectra were measured as the temperature was elevated from 20 to 90 °C at intervals of 2 °C. A CD spectrum at each temperature was obtained by equilibrating the sample for 5 min prior to data collection. Buffer spectra were measured in the same temperature region, and were subtracted from sample spectra at the corresponding temperature. Transition curves for the thermal denaturation of β-WT, β-L172I, β-L174V, and β-E228G proteins were obtained from ellipticity at 222 nm.
Analysis of Gibbs free energy of unfolding (ΔGunf)
In thermal denaturation experiments, the thermal stabilities of β-WT, β-L172I, β-L174V, and β-E228G proteins were calculated assuming a two-state denaturation process.Citation31,32) The fraction of the unfolded protein, Fu, was calculated using the equation below(2)
where [θ]obs, [θ]u, and [θ]f represent the CD signals at a particular temperature, completely unfolded state, and completely folded state, respectively.
The apparent equilibrium constant of unfolding (K) and corresponding ΔGunf were calculated using Equations (Equation3(3) ) and (Equation4
(4) )
(3)
(4)
where R is the gas constant and T is the absolute temperature. We here calculated ΔGunf at 25 °C (T = 298 K).
Statistical analyses
Statistical analyses were performed using Stat View 5.0 (Hulinks, Tokyo, Japan). Results are presented as the mean and SD, and differences were considered significant at p < 0.05. A one-way factorial ANOVA was performed to determine overall differences among ΔGunf of β-WT, β-L172I, β-L174V, and β-E228G proteins and, if significant differences existed, post hoc analysis tests (Fisher’s PLSD) were performed.
Results
β-L172I, β-L174V, and β-E228G proteins were indicative of α-helical structure and did not change the secondary structure of FcεRI β
In order to investigate the effects of β-L172I, β-L174V, and β-E228G on the secondary structure of FcεRI β, we measured the far-UV CD spectra of β-WT, β-L172I, β-L174V, and β-E228G proteins at an ambient temperature. Fig. shows the far-UV CD spectra of β-WT, β-L172I, β-L174V, and β-E228G proteins, respectively. The CD spectra of β-WT, β-L172I, β-L174V, and β-E228G proteins had negative maxima at 208 and 222 nm. No significant differences were observed between β-WT and β-L172I, β-L174V, and β-E228G proteins. The far-UV CD spectra of β-WT and β-L172I, β-L174V, and β-E228G proteins were indicative of an α-helical structure and β-L172I, β-L174V, and β-E228G proteins did not show any loss or collapse in the α-helical content, suggesting that β-L172I, β-L174V, and β-E228G did not change the secondary structure of FcεRI β.
β-E228G affected the thermal stability of FcεRI β
The thermal stability of the secondary structure was investigated using a CD intensity at 222 nm in a temperature range between 20 and 90 °C. Fig. shows the transition curve for the thermal denaturation of β-WT, β-L172I, β-L174V, and β-E228G proteins, respectively. The characteristics of their transition curves for thermal denaturation were considered to be different. These results suggest that β-L172I, β-L174V, and β-E228G may affect temperature-induced structural changes in FcεRI β.
Fig. 3. Transition curve for the thermal denaturation of β-WT, β-L172I, β-L174V, and β-E228G proteins obtained from ellipticity at 222 nm.
Notes: The black, blue, green, and red lines indicate β-WT, β-L172I, β-L174V, and β-E228G, respectively. One representative of three individual experiments is shown.
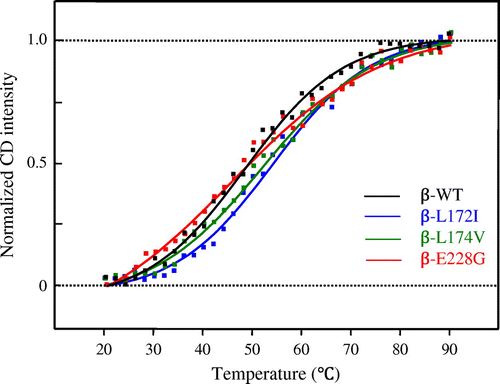
Significant differences were observed in ΔGunf values between β-E228G and other β chain proteins
ΔGunf was calculated to investigate the thermal stability of β-WT, β-L172I, β-L174V, and β-E228G proteins in more detail. The ΔGunf values of β-WT, β-L172I, β-L174V, and β-E228G proteins were obtained from a two-state denaturation model.Citation31,32) The ΔGunf values of β-WT, β-L172I, β-L174V, and β-E228G proteins were shown in Fig. . Significant differences were observed in ΔGunf values between β-WT and β-E228G (p < 0.05), between β-L172I and β-E228G (p < 0.05), and between β-L174 V and β-E228G (p < 0.01). These results suggested that β-E228G affected the thermal stability of FcεRI β.
Fig. 4. Mean (±SD) value of ΔGunf (kJ/mol) for β-WT, β-L172I, β-L174V, and β-E228G proteins obtained from transition curves in the thermal denaturation experiment (three individual experiments were performed).
Notes: Each column and bar represents the mean value and SD, respectively. Data points with an “ asterisk” indicate a significant p value (*p < 0.05, **p < 0.01). The ΔGunf values of β-WT, β-L172I, β-L174V, and β-E228G proteins were 6.0 ± 1.0, 6.8 ± 1.0, 8.6 ± 2.5, 3.1 ± 0.8 kJ/mol, respectively.
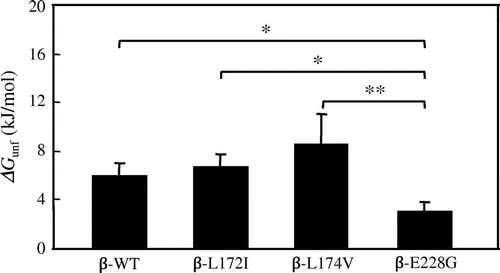
β-L172I, β-L174V, and β-E228G did not affect the conformation in the local environment around ITAM tyrosines
In order to observe the changes affecting the environment of aromatic amino acids in β-L172I, β-L174V, and β-E228G proteins, CD spectra were measured in the near-UV region. Fig. shows the near-UV CD spectra of β-WT, β-L172I, β-L174V, and β-E228G proteins, respectively. The near-UV CD bands of proteins mainly arose from the conformation asymmetrically formed around aromatics, such as Phe, Tyr, and Trp. The features of the near-UV CD spectra of β-L172I, β-L174V, and β-E228G proteins were similar to that of the β-WT protein. Conformational changes were not observed with decreases in the band intensity in β-L172I, β-L174V, and β-E228G. These results suggested that β-L172I, β-L174V, and β-E228G did not affect the conformation in the local environment around ITAM tyrosines.
Discussion
FcεRI β acts as a signal amplifier through the ITAM in its C-terminal region and also functions as a stabilizer of the FcεRI complex.Citation7–10) In humans, a polymorphism (E237G) corresponding to mouse E228G has been linked to atopy, asthma, and allergies.Citation20,22) On the other hand, E237G has not been associated with either a positive skin prick test or total serum IgE levels.Citation26) However, previous studies on the role of E237G in the expression and function of FcεRI have not yielded a clearer understanding of its effects on mast cell physiology. Furthermore, the structural and conformational aspects and thermal stability of FcεRI β remain largely unknown. In the present study, we revealed that β-L172I, β-L174V, and β-E228G did not affect the secondary structure or conformation of FcεRI β; however, β-E228G affected its thermal stability.
The following reasons were suggested for why β-E228G affected the thermal stability of FcεRI β. The conformation of the whole main chain of the protein is determined by two conformational angles, phi (φ) and psi (ψ), for each amino acid, for which the plot is called a Ramachandran plot.Citation33,34) Gly, with only a hydrogen atom as a side chain, has the ability to adopt a markedly wider range of conformations than the other residues. However, Glu is restricted in its range of conformations. Gly is known to play a very important role in protein structure, and the properties of Gly may affect the thermal stability of FcεRI β.
The cytoplasmic tail of FcεRI β in the vicinity of amino acid residue 228 is rich in acidic amino acid residues. The hydropathy index of Glu and Gly were previously reported to be −3.5 and −0.4, respectively.Citation35) These findings indicate that β-E228G introduces a hydrophobic change in the C-terminal region of FcεRI β. β-E228G may also change hydrogen bonding between amino acid residues in the secondary structure and its conformation. In β-E228G, these factors may affect the thermal stability of FcεRI β.
The Src family protein tyrosine kinase Lyn has been shown to weakly bind to the β chain ITAM.Citation36–38) Changes in the thermal stability of β-E228G may affect the protein–protein interactions of tyrosine kinases, for example, Lyn with FcεRI β. Furthermore, we previously reported that the mutagenesis of a single C-terminal Asp to Ala (β-D234A) significantly impaired the production of IL-6, but not degranulation in mast cells.Citation39) These findings strongly suggest a novel signaling pathway mediated by the cytoplasmic tail downstream of the FcεRI β ITAM. β-D234A is known to have no effect on the secondary structure of FcεRI β. Minami et al. also showed that no significant differences were observed in the secondary structure and conformation of commercial plasma-derived (pHSA) and recombinant human serum albumin (rHSA) products; however, significant differences were noted in their ΔGunf values.Citation40) They also showed that pHSA and rHSA products had different cell proliferation activities for some cell types. These findings demonstrated that β-E228G affected the thermal stability of FcεRI β, and based on the difference in thermal stability, may also affect the function of FcεRI β. However, we did not use differential scanning calorimetry in the present study because we could not purify FcεRI β proteins at high concentrations. And we did not investigate the structure and thermal stability using β chain proteins with full length. In addition, β chain proteins with a large amount of hydrophobic residues were investigated in an aqueous solution. Furthermore, destabilization of β chain might affect the phosphorylation of ITAM tyrosine of β chain, however, the mechanism such as ubiquitination or other post translational modifications in mast cell by which the thermal stability of the β-E228G protein may affect its biological function requires future investigations.
Authors contributions
Study concept and design: Terada T. Acquisition of data: Terada T, Takahashi T, Arikawa H. Analysis and interpretation of data: Terada T, Takahashi T, Arikawa H, Era S. Drafting of the manuscript: Terada T and Era S. All authors reviewed and approved the final manuscript.
Disclosure statement
No potential conflict of interest was reported by the authors.
Funding
This work was supported in part by a Grant-in-Aid for Scientific Research from the Ministry of Education, Science, Sports, and Culture of Japan [grant number 26461577] and by a grant from Gifu University.
Acknowledgments
We thank the Division of Instrumental Analysis, Life Science Research Center, Gifu University for its technical support.
Notes
* Abbreviations: FcεRI, high-affinity IgE Fc receptor; ITAM, immunoreceptor tyrosine-based activation motif; ΔGunf, Gibbs free energy of unfolding; CD, circular dichroism
References
- Metcalfe DD, Baram D, Mekori YA. Mast. cells. Physiol. Rev. 1997;77:1033–1079.
- Kinet JP, Blank U, Ra C, et al. Isolation and characterization of cDNAs coding for the beta subunit of the high-affinity receptor for immunoglobulin E. Proc. Natl. Acad. Sci. USA. 1988;85:6483–6487.10.1073/pnas.85.17.6483
- Blank U, Ra C, Miller L, et al. Complete structure and expression in transfected cells of high-affinity IgE receptor. Nature. 1989;337:187–189.10.1038/337187a0
- Rivera J, and Gilfillan AM. Molecular regulation of mast cell activation. J. Allergy Clin. Immunol. 2006;117:1214–1225 quiz 1226. 10.1016/j.jaci.2006.04.015
- Ra C, Jouvin MH, Kinet JP. Complete structure of the mouse mast cell receptor for IgE (Fc epsilon RI) and surface expression of chimeric receptors (rat-mouse-human) on transfected cells. J. Biol. Chem. 1989;264:15323–15327.
- Galli SJ, Tsai M. Mast cells in allergy and infection: versatile effector and regulatory cells in innate and adaptive immunity. Eur. J. Immunol. 2010;40:1843–1851.10.1002/eji.201040559
- Dombrowicz D, Lin S, Flamand V, et al. Allergy-associated FcRβ is a molecular amplifier of IgE- and IgG-mediated in vivo responses. Immunity. 1998;8:517–529.10.1016/S1074-7613(00)80556-7
- Lin S, Cicala C, Scharenberg AM, et al. The FcεRIβ subunit functions as an Amplifier of FcεRIγ-mediated cell activation signals. Cell. 1996;85:985–995.10.1016/S0092-8674(00)81300-8
- Hiraoka S, Furumoto Y, Koseki H, et al. Fc receptor beta subunit is required for full activation of mast cells through Fc receptor engagement. Int. Immunol. 1999;11:199–207.10.1093/intimm/11.2.199
- Turner H, Kinet JP. Signalling through the high-affinity IgE receptor FcεRI. Nature. 1999;402:24–30.10.1038/35037021
- Nadler MJ, Matthews SA, Turner H, et al. Signal transduction by the high-affinity immunoglobulin E receptor Fc epsilon RI: coupling form to function. Adv. Immunol. 2000;76:325–355.
- Furumoto Y, Nunomura S, Terada T, et al. The FcepsilonRIbeta immunoreceptor tyrosine-based activation motif exerts inhibitory control on MAPK and IkappaB kinase phosphorylation and mast cell cytokine production. J. Biol. Chem. 2004;279:49177–49187.10.1074/jbc.M404730200
- Holgate ST. Genetic and environmental interaction in allergy and asthma. J. Allergy Clin. Immunol. 1999;104:1139–1146.10.1016/S0091-6749(99)70005-9
- Barnes KC. Gene–environment and gene–gene interaction studies in the molecular genetic analysis of asthma and atopy. Clin. Exp. Allergy. 1999;Suppl 4:47–51.
- Bleecker ER. Mapping susceptibility genes for asthma and allergy. Clin. Exp. Allergy. 1998;Suppl 5:6–12.10.1046/j.1365-2222.1998.028s5006.x
- Cookson WO, Sharp PA, Faux JA, et al. Linkage between immunoglobulin E responses underlying asthma and rhinitis and chromosome 11q. Lancet. 1989;333:1292–1295.10.1016/S0140-6736(89)92687-1
- Sandford AJ, Shirakawa T, Moffatt MF, et al. Localisation of atopy and beta subunit of high-affinity IgE receptor (Fcepsilon RI) on chromosome 11q. Lancet. 1993;341:332–334.10.1016/0140-6736(93)90136-5
- Collée JM, ten Kate LP, de Vries HG, et al. Allele sharing on chromosome 11q13 in sibs with asthma and atopy. Lancet. 1993;342:936.10.1016/0140-6736(93)91988-X
- Shirakawa T, Hashimoto T, Furuyama J, et al. Linkage between severe and chromosome 11q13 in Japanese families. Clin. Genet. 1994;46:228–232.
- Hill MR, Cookson WOCM. A new variant of the beta subunit of the high-affinity receptor for immunoglobulin E (Fc epsilon RI-beta E237G): associations with measures of atopy and bronchial hyper-responsiveness. Hum. Mol. Genet. 1996;5:959–962.10.1093/hmg/5.7.959
- Hill MR, James AL, Faux JA, et al. Fc(epsilon)RI-(beta) polymorphism and risk of atopy in a general population sample. Br. Med. J. 1995;311:776–779.10.1136/bmj.311.7008.776
- Shirakawa T, Li A, Dubowitz M, et al. Association between atopy and variants of the β subunit of the high–affinity immunoglobulin E receptor. Nat. Genet. 1994;7:125–130.10.1038/ng0694-125
- Shirakawa T, Mao XQ, Sasaki S, et al. Association between atopic asthma and coding variant of Fc epsilon RI beta in a Japanese population. Hum. Mol. Genet. 1996;5:1129–1130.10.1093/hmg/5.8.1129
- Li A, Mackay GA, Hoplin JM. Functional analysis of histamine release from basophils and mast cells in subjects with the Ile-181→Leu variant of Fc epsilon RI-beta. Clin. Sci. 1997;93:279–286.
- Kim Y, Oh SY, Oh HB, et al. Coding single nucleotide polymorphism in the high-affinity immunoglobulin E receptor β chain (FcepsilonRI-beta) gene is associated with immunoglobulin E receptor-mediated histamine release from basophils. Clin. Exp. Allergy. 2002;32:751–755.10.1046/j.1365-2222.2002.01295.x
- Kim YK, Park HW, Yang JS, et al. Association and functional relevance of E237G, a polymorphism of the high-affinity immunoglobulin E-receptor β chain gene, to airway hyper-responsiveness. Clin. Exp. Allergy. 2007;37:592–598.10.1111/cea.2007.37.issue-4
- Furumoto Y, Hiraoka S, Kawamoto K, et al. Polymorphisms in FcϵRI β chain do not affect IgE-mediated mast cell activation. Biochem. Biophys. Res. Commun. 2000;273:765–771.10.1006/bbrc.2000.2989
- Zloh M, Anderson G, Clark-Lewis I, et al. Spectroscopic and conformational studies of the C-terminal cytoplasmic beta subunit 46-peptide of the high-affinity IgE receptor. Biochem. Soc. Trans. 1994;22:450S.10.1042/bst022450s
- Zloh M, Anderson G, Clark-Lewis I, et al. N.m.r studies of the cytoplasmic C-terminal β-subunit domain of the high-affinity IgE receptor. Biochem. Soc. Trans. 1994;22:1027–1029.10.1042/bst0221027
- Zloh M, Biekofsky RR, Duret J-A, et al. Conformational studies of the beta-subunit of the high-affinity IgE receptor: circular dichroism and molecular modeling. Biomed. Pept. Proteins Nucleic Acids. 1995;1:101–106.
- Privalov PL. Stability of proteins:small globular proteins. Adv. Protein Chem. 1979;33:167–241.10.1016/S0065-3233(08)60460-X
- Pace CN. Determination and analysis of urea and guanidine hydrochloride denaturation curves. Methods Enzymol. 1986;131:266–280.10.1016/0076-6879(86)31045-0
- Ramachandran GN, Sasisekharan V. Conformation of polypeptides and proteins. Adv. Prot. Chem. 1968;23:283–437.10.1016/S0065-3233(08)60402-7
- Richardson JS. The anatomy and taxonomy of protein structure. Adv. Prot. Chem. 1981;34:167–339.10.1016/S0065-3233(08)60520-3
- Kyte J, Doolittle RF. A simple method for displaying the hydropathic character of a protein. J. Mol. Biol. 1982;157:105–132.10.1016/0022-2836(82)90515-0
- Eiseman E, Bolen JB. Engagement of the high-affinity IgE receptor activates src protein-related tyrosine kinases. Nature. 1992;355:78–80.10.1038/355078a0
- Yamashita T, Mao SY, Metzger H. Aggregation of the high-affinity IgE receptor and enhanced activity of p53/56lyn protein-tyrosine kinase. Proc. Natl. Acad. Sci. USA. 1994;91:11251–11255.10.1073/pnas.91.23.11251
- Vonakis BM, Chen HX, Haleem-Smith H, et al. The unique domain as the site on Lyn kinase for its constitutive association with the high-affinity receptor for IgE. J. Biol. Chem. 1997;272:24072–24080.10.1074/jbc.272.38.24072
- Terada T, Nunomura S, Shimokawa T, et al. FcεRI-induced mast cell cytokine production critically involves an aspartic acid residue (D234) in the C-terminal intracellular domain of the FcεRIβ chain. Biochem. Biophys. Res. Commun. 2011;410:744–748.10.1016/j.bbrc.2011.06.030
- Minami T, Terada T, Takahashi T, et al. Comparative studies on the heterogeneity of plasma-derived and recombinant human albumins in laboratory use. Int. J. Biol. Macromol. 2014;69:79–87.10.1016/j.ijbiomac.2014.05.010