Abstract
Free fatty acids are useful as source materials for the production of biodiesel fuel and various chemicals such as pharmaceuticals and dietary supplements. Previously, we attained a 9.2-fold increase in free fatty acid productivity by disrupting a predicted acyl-CoA synthetase gene (faaA, AO090011000642) in Aspergillus oryzae. In this study, we achieved further increase in the productivity by overexpressing a predicted transketolase gene of the pentose phosphate pathway in the faaA disruptant. The A. oryzae genome is predicted to have three transketolase genes and overexpression of AO090023000345, one of the three genes, resulted in phenotypic change and further increase (corresponding to an increased production of 0.38 mmol/g dry cell weight) in free fatty acids at 1.4-fold compared to the faaA disruptant. Additionally, the biomass of hyphae increased at 1.2-fold by the overexpression. As a result, free fatty acid production yield per liter of liquid culture increased at 1.7-fold by the overexpression.
Graphical abstract
The A. oryzae ΔfaaA_tktOE overexpressing a predicted transketolase gene in the pentose phosphate pathway increased FFA yield at 1.7-fold from the ΔfaaA and showed phenotypic change.
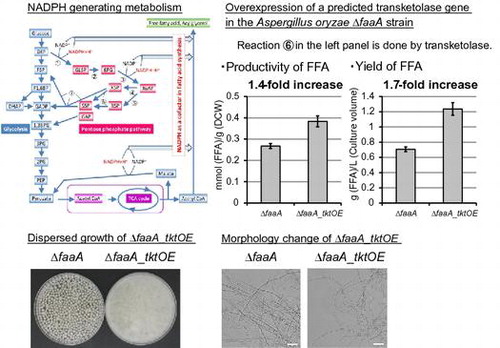
Involved in the increasing global demand of fossil fuels, biofuels generated from plant biomass have significantly been focused due to less effect on global environment in the carbon neutral characteristics.Citation1,2) In addition, some pharmaceuticals and dietary supplements produced from rare natural resources are expensive, and their production by the heterologous microbial biosynthesis for lowering the prices has been desired.Citation3,4)
Microbial lipid production has been researched in bacteria (Escherichia coli),Citation5−7) yeast (Saccharomyces cerevisiae,Citation8−10) Yarrowia lipolytica,Citation11) etc.), filamentous fungi (A. oryzae,Citation12,13) Mortierella alpina,Citation14) etc.), and photosynthetic microorganisms called algae.Citation15,16) Free fatty acid (FFA), acylglycerol, fatty acid methyl ester, and alkane have been the major targets of the microbial production.Citation1) Among them, FFA can be used as source materials of biodiesel fuels and chemicals.Citation1)
The filamentous fungus A. oryzae is well known for high production capability of hydrolases such as amylase, protease, and lipase.Citation17) In addition, A. oryzae is not only a producer of small molecules such as the kojic acid,Citation18) but also listed as a safe microorganism for the GRAS (i.e. Generally Recognized as Safe) status by the US Food and Drug Administration.Citation19) Thus, it implies A. oryzae is superior to the other filamentous fungi in the production of a wide range of biomolecules for industrial application. In fact, it has been used for producing sake (rice wine), shoyu (soy sauce), and miso (soybean paste) by converting starch, proteins, and lipids derived from rice, wheat, and soybean, to many varieties of small molecules through the catabolism and the assimilation.
In our research, A. oryzae has been used for accomplishing high production of lipids such as FFA and acylglycerol as source materials of biofuels and chemicals.Citation12,13) To date, overexpression of a fatty acid synthase (FAS) gene involved in the de novo fatty acid biosynthesis enhanced the FFA productivity at 2.1-fold.Citation12) Disruption of an acyl-CoA synthetase gene faaA also drastically increased the FFA productivity at 9.2-fold.Citation13) However, construction of a double mutant having both the FAS overexpression and the faaA disruption has been difficult for unknown reason and been under construction.
The pentose phosphate pathway (PPP) exists commonly in a broad range of organisms and generates both NADPH and pentose molecules. It begins with the glucose 6-phosphate molecule synthesized at the first reaction step of glycolysis, thus PPP competes the use with the glycolysis. PPP consists of seven reaction steps until it finally merges into glycolysis again (Fig. , left panel). Thus, PPP is categorized to a primary metabolism. NADPH is required for de novo fatty acid biosynthesis as a cofactor of FAS reaction. Therefore, PPP was considered to be one of possible bottlenecks regulating the FFA productivity. In fact, PPP is suggested as a major NADPH supplier of FFA biosynthesis in an oleaginous yeast Y. lypolitica.Citation11) Based on the information, the enzyme genes of PPP were individually overexpressed in the A. oryzae faaA disruptant aiming at further increase in FFA productivity in this study.
Fig. 1. Illustration of cytosolic NADPH generating metabolism (left) and a list of enzyme genes predicted to work in the PPP of A. oryzae (right).
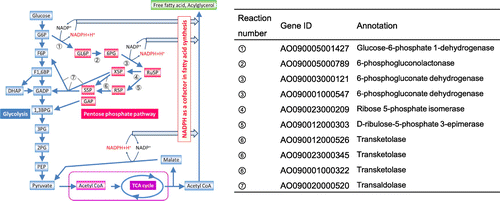
Materials and methods
Fungal strains and culture media
Overexpression mutants for the enzyme genes of PPP were constructed from the A. oryzae faaA disruptant (ΔfaaA) originated from the strain NS4,Citation20) the spontaneous auxotrophic mutant of the wild-type strain RIB40. Construction of the ΔfaaA strain is described in our previous paper.Citation13) The A. nidulans A851 strain was obtained from the Fungal Genetics Stock Center (Kansas City, MO, USA), and used to amplify A. nidulans pyrG, sC, and niaD (abbreviated AnpyrG, AnsC, and AnniaD) as selection markers. Genotype of the ΔfaaA strain is A. oryzae NS4 ΔligD::ptrA ΔfaaA::AnpyrG ΔpyrG::AnsC ΔniaD::AnniaD. Each A. oryzae strain was maintained on Czapek-Dox (CD) minimal agar medium with and without 5 mM uridine and 10 mM uracil. The CD medium contained 2% glucoseCitation21); however, the glucose concentration was increased to 10% for analyzing the FFA productivity.
Individual overexpression of PPP genes in the A oryzae ΔfaaA strain
Introduction of individual PPP gene overexpression to the ΔfaaA strain was performed by replacing its native promoter with the A. oryzae tef1 (AO090120000080) promoter named Ptef1. The Ptef1 has a constitutively high transcriptional activity and has been used for overexpression of the other genes in A. oryzae.Citation22) All primers used in this study are listed in Table S1. Individual PPP-gene-overexpressing ΔfaaA strains were constructed by the following two steps (Fig. S1A and B).
First, AnpyrG was removed from the chromosome of the ΔfaaA strain. Fragments 1.1 kb upstream and 1.2 kb downstream of faaA were amplified using a set of LU and LL [pyrG_del] primers and a set of RU [pyrG_del] and RL primers, respectively (Fig. S1C). Primer sequences in lower case designate the tails for fusion to respective adjacent DNA fragments during fusion PCR (Table S1). Each DNA fragment was amplified using KOD-PLUS DNA polymerase (Toyobo, Japan; distributed as KOD HOT START DNA polymerase worldwide). The two DNA fragments were purified by agarose gel extraction using the Wizard SV Gel and PCR Clean-Up System (Promega, Madison, WI, USA). The purified DNA fragments were subjected to fusion PCR using the LU/RL primer pair and the KOD-PLUS DNA polymerase (Fig. S1C). Fusion PCR was performed as follows: denaturation at 94 °C for 2 min, followed by 25 cycles of 94 °C for 15 s, 66 °C for 30 s, and 68 °C for 2 min 30 s, with a final extension at 68 °C for 2 min 30 s. The resulting 2.3-kb DNA disruption constructs were purified by agarose gel extraction and introduced into the ΔfaaA strain. Transformation was performed as described in a previous paper.Citation21) Transformants were regenerated on CD agar containing 1.2 M sorbitol, 5 mM uracil, 10 mM uridine, and 1 mg/mL 5-fluoroorotic acid. After single spore isolation, positive clones were selected by PCR with the LU and cL primers.
Second, the resulting ΔfaaA strain lacking AnpyrG (named ΔfaaA_pyrG-) was transformed with the DNA fragment for each PPP gene overexpression. Two 1-kb DNA fragments of the promoter and the coding sequence starting from a start codon of each PPP gene were amplified with LU/LL and RU/RL primer pairs from A. oryzae genomic DNA template (Fig. S1D). These DNA fragments were mixed with a 1.8-kb fragment of AnpyrG and a 1-kb Ptef1 DNA fragment, and were subjected to fusion PCR with primers LU and RL using an extension time of 5 min. The resultant fragment harboring each gene portion whose promoter was replaced with Ptef1 was introduced to the ΔfaaA_pyrG- strain by transformation. The transformants selected on CD agar medium were analyzed by PCR with LU and cL primers for every PPP gene overexpression, and by Southern hybridization for only AO090023000345 overexpression to confirm integration of the DNA fragments into the expected chromosomal locus as the individual PPP-gene-overexpressing ΔfaaA strains (Fig. S2).
Southern hybridization
Aliquots (3 μg) of genomic DNA were digested with each restriction enzyme (EcoRI, HincII), fractionated on 0.7% agarose gel, and transferred onto a Nitran SuperCharge membrane (GE Healthcare, Piscataway, NJ, USA). Hybridization and signal detection were performed with a digoxigenin (DIG) system according to the manufacturer instructions (GE Healthcare). Briefly, DIG-labeled probes (approximately 800-bases long) were prepared using a PCR DIG Probe Synthesis Kit (Roche Applied Science, Mannheim, Germany) with primers SBU and SBL (Table S1), followed by agarose gel extraction. The probes were hybridized to the DNA fragments bound to the membrane, and signals of the probes were detected via chemiluminescence arising from the anti-DIG Fab-fragment-alkaline phosphatase conjugate (Roche Applied Science) using the CDP-Star Detection Reagent (GE Healthcare).
Intracellular FFA assay for evaluating the productivity of A oryzae
The constructed overexpression mutants were subjected to intracellular FFA assay for evaluation of the FFA productivity. Cultures were prepared by inoculating 2.5 × 107 spores of each A. oryzae strain to 50 mL CD liquid medium supplemented with 10% glucose in 250-mL flasks. These were incubated at 30 °C, 200 rpm for 120 h. Hyphae were collected by filtration through Miracloth (EMD Chemicals, San Diego, CA, USA), followed by two washes with 100 mL Milli-Q water. Excess water was removed from the filtered hyphae by squeezing between paper towels. The hyphae were immediately frozen in liquid nitrogen and lyophilized overnight.
To determine intracellular FFA levels, 50 mg of lyophilized hyphae were mixed with 300 mg of 0.5 mm glass beads (TOMY, Tokyo, Japan) in a 2-mL screw-cap tube (SARSTEDT, Nümbrecht, Germany) containing 720 μL Milli-Q water and 720 μL chloroform containing 1% (w/v) Triton X-100. The hyphae were disrupted with a micro-homogenizing system MicroSmash MS-100 (TOMY) at 5,500 rpm for 60 s. After disruption, the hyphae were centrifuged at 14,000 × g at 25 °C for 10 min. After the bottom of the screw-cap tube was punctured with a needle, the lower chloroform layer was dropped down to a new 1.5 mL microcentrifuge tube by gravity. Aliquots (90 μL) of the dropped chloroform layer were exactly transferred to another new 1.5-mL microcentrifuge tube by a 0.1-mL glass syringe. Chloroform in the transferred sample was evaporated by overnight incubation at 50 °C in a fume hood. The pellet, which was a mixture of lipids and Triton X-100, was then dissolved by pipetting up and down after sequential addition of 1 μL ethanol, 14 μL Milli-Q water, and 735 μL of a solution containing 6% ethanol and 6% (w/v) Triton X-100. Aliquots (5 μL) of the sample were assayed for intracellular FFA using a commercial FFA enzyme assay kit (FFAs Half-micro-test kit; Roche Applied Science).
Quantitative reverse transcription polymerase chain reaction (RT-PCR)
To determine transcript levels using RT-PCR, each A. oryzae strain was cultured at 30 °C at 200 rpm for 48 h in the same inoculum and culture medium as the case of FFA productivity evaluation. Hyphae were harvested and immediately frozen with liquid nitrogen. Then, 2 μg of total RNA were prepared by the extraction using ISOGEN reagent (Nippongene, Toyama, Japan). Contaminating chromosomal DNA was removed by treatment with RNase-free DNase I (New England Biolabs, Ipswich, MA, USA). These samples were then used as templates for cDNA synthesis reaction using the high capacity RNA-to-cDNA kit (Applied Biosystems, Foster City, CA, USA). A portion of 1 μL of each resulting cDNA solution was applied to quantitative real-time PCR with the Power SYBR Green PCR master mix (Applied Biosystems) using the StepOnePlus Real-time PCR instrument (Applied Biosystems). Primers named “real_fwd” and “real_rev” were used for quantification of each gene expression level. The RT-PCR program was set as 45 cycles of 95 °C for 15 s and 60 °C for 1 min after an initial incubation step of 50 °C for 2 min and the subsequent 95 °C for 10 min. Each CT value was automatically calculated by the instrument.
DNA standards of the genes subjected to RT-PCR were also prepared by PCR with “real_fwd” and “real_rev” primers specific to each gene (Table S1). They were purified by gel extraction using Wizard SV Gel and PCR Clean-Up System (Promega) and the DNA concentration of each standard solution was assayed using the Quant-iT dsDNA BR assay kit (Invitrogen, Carlsbad, CA, USA) before utilized in RT-PCR. A standard curve for quantification was then made from serial dilutions of the DNA standard for each gene product from 0.002 pM to 250 pM by plotting CT values against concentrations.
Absolute transcript level of each gene was determined by measuring the cDNA concentration corresponding to the CT value using the standard curve. Previous DNA microarray results indicated a stable and constitutive expression level of the histone gene, AO090012000496, over a variety of culture conditions.Citation23) Thus, the cDNA amount of each sample was normalized by the expression level of the histone gene determined by RT-PCR with primers, 012-0496real_fwd and 012-0496real_rev.
Microscopic observation of the A oryzae hyphae
The 2.5 × 107 spores of each A. oryzae strain were inoculated to 50 mL of the CD (10% glucose) liquid culture medium and cultivated at 30 °C at 200 rpm for 48 h. A small amount of hyphae was transferred on a slide glass and observed at 200-fold magnification by optical microscopy using the BX53 microscope system (Olympus, Tokyo, Japan).
Results
Prediction of the A. oryzae PPP genes and their individual overexpression in the ΔfaaA strain
Based on the previous paper, PPP was deduced to play a major role in NADPH provision for de novo fatty acid biosynthesis.Citation11) To increase FFA productivity of A. oryzae by metabolic engineering, NADPH seemed to lack due to the high consumption at FFA biosynthesis reaction. Thus, we attempted enhancement of the PPP function. Introduction of individual PPP gene overexpression to the ΔfaaA strain was chosen as the strategy. Referring to an information about the improved gene annotation of A. oryzae genome,Citation24) 10 genes listed at the right panel of Fig. were predicted to encode the PPP genes in A. oryzae. The listed genes were individually subjected to overexpression in the ΔfaaA strain. The mutant strains having each PPP gene overexpression as well as the faaA knockout were constructed as described in Fig. S1 and the Materials and Methods. Homologous recombination in the construction was verified by PCR and southern hybridization analysis (data not shown and Fig. S2). As a result, each PPP gene overexpression mutant was successfully constructed as a homokaryon except for glucose 6-phosphate 1-dehydrogenase gene overexpression mutant, which was difficult in isolating a homokaryon by single-spore isolation.
Further increase in the FFA productivity by overexpression of a predicted transketolase gene in the ΔfaaA strain
The FFA productivity was analyzed in the constructed mutants of each predicted PPP gene overexpression with the faaA knockout as described in Materials and Methods. The results showed that a small increase in the FFA productivity occurred only in the ΔfaaA_tktOE strain that overexpressed a predicted transketolase gene AO090023000345 and lacked faaA (Fig. (A)). The ΔfaaA_tktOE strain produced FFA at 0.38 mmol/g dry cell weight [DCW]. It was 1.4-fold greater than the one in the parental ΔfaaA strain (0.27 mmol/g DCW). As the molar amount of produced FFA was converted to equivalent weight of palmitic acid, the FFA amount produced by the ΔfaaA_tktOE strain was 98 mg/g DCW, corresponding to 1.2 g of FFA production in the biomass obtained from a liter of culture medium (Fig. (B)). The other PPP gene overexpression in the ΔfaaA strain did not show significant increase in the FFA productivity from the parental ΔfaaA strain (Fig. S3). Intracellular acylglycerol amount was not affected by any PPP gene overexpression in the ΔfaaA strain (data not shown). No fatty acids were detected in the culture supernatant of any mutants, indicating that FFA was not secreted by any constructed mutants.
Phenotype of the A. oryzae ΔfaaA_tktOE strain
Overexpression of a predicted transketolase gene AO090023000345 in the ΔfaaA_tktOE strain was checked by quantitative RT-PCR. As a reference, the ΔfaaA strain was used. After the cultivation in CD liquid medium at 30 °C for 48 h, RNA was extracted. The cDNA made from the RNA was used as a template for RT-PCR. The constitutively expressing histone gene was used as an internal standard of a total RNA and relative expression level to the histone gene was calculated.Citation23) As a result, expression level of AO090023000345 was elevated at about 20,000-fold in the ΔfaaA_tktOE strain from the level in the ΔfaaA strain (Table ).
Table 1. Expression level of a predicted transketolase gene in each mutant of 2 days (48 h) growth.
Growth was then assessed on CD agar plates. An aliquot of 5 × 104 spores of each mutant was spotted at the center of the agar plate (Fig. (A)), while another set of plates was prepared by spreading 5 × 105 spores across the entire surface. After incubation at 30 °C for 7 days, the ΔfaaA_tktOE strain showed slightly slower growth rate at the 0.9-fold of the parental ΔfaaA strain (Table and Fig. (A)). However, because number of collected spores from the spread-inoculated plates was similar between the ΔfaaA_tktOE and the ΔfaaA strains, spore formation efficiency seemed to be approximately the same between both strains (data not shown). Overall, our results indicate that introduction of AO090023000345-overexpression to the ΔfaaA strain does not affect the efficiency of spore formation, but slightly decreases the radial growth rate on CD agar medium.
Fig. 3. Photographs of the ΔfaaA (left) and the ΔfaaA_tktOE (right) strains.
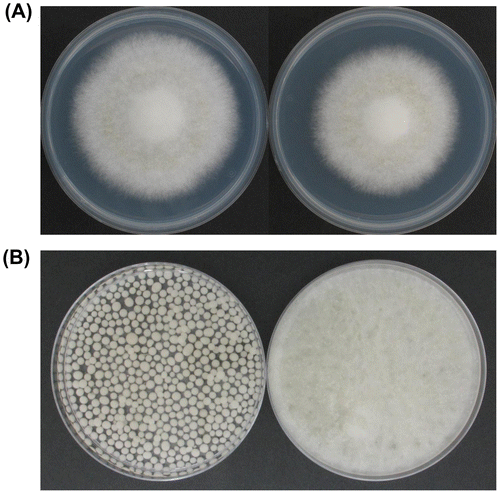
Table 2. Diameter of colony of each mutant of 7 days (144 h) growth.
Interestingly, the ΔfaaA_tktOE strain showed different appearances from the ΔfaaA strain in liquid culture. The ΔfaaA_tktOE strain grew as the hyphae being dispersed like pulp (Fig. (B), right). Generally, A. oryzae strains including the parental ΔfaaA strain grow as forming clusters of hyphae in the liquid culture (Fig. (B), left). Diameter of the clusters generally distributes in a broad range from 1 mm to 10 mm. When their hyphae at 48 h period from the initiation of liquid culture were observed by microscopy, hyphae of the ΔfaaA strain at the surface of the clusters generally looked straightforward like rods (Fig. (A) and (B)), while hyphae of the ΔfaaA_tktOE strain dispersing in the culture generally looked curved (Fig. (C) and (D)). In addition, spherical structures were frequently found in the hyphae (Fig. (E)). Some spherical structures were formed at the tip of hyphae, while the other spherical structures were made in the middle of hyphae. On the other hand, no spherical structures were found in the hyphae of the ΔfaaA strain.
Fig. 4. Microscopic observation of liquid cultured hyphae of the ΔfaaA (A, B) and the ΔfaaA_tktOE (C–E) strains.
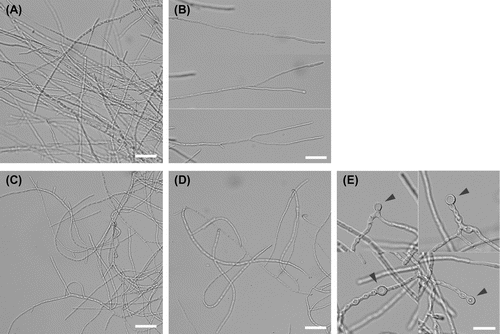
Discussion
In this study, with respect to PPP composed of sequential seven enzyme reactions, only overexpression of a predicted transketolase gene AO090023000345 increased FFA productivity from the parental A. oryzae ΔfaaA strain. It would imply the transketolase reaction is a bottleneck of PPP in A. oryzae. Transketolase converts a pair of ribose 5-phosphate and xylulose 5-phosphate to a pair of sedoheptulose 7-phosphate and glyceraldehyde 3-phosphate. Although the transketolase reaction does not generate NADPH, by enhancing the reaction by the gene overexpression, a total metabolic flux of PPP is deduced to elevate.
There are the other two genes predicted to encode transketolase in the A. oryzae genome. However, these overexpressions in the ΔfaaA strain did not increase FFA productivity. For one probable reason, the transketolase reaction would majorly be performed by the enzyme encoded by AO090023000345 in A. oryzae. However, as far as looking at their homologies to the identified transketolase gene TKL1 of S. cerevisiae by BLASTP, AO090012000526 is most homologous to TKL1 at 62% identity. On the other hand, both AO090023000345 and AO090001000322 have homologies to TKL1 at only 42% identity, respectively. Therefore, for another probable reason, AO090023000345 would function as an enzyme other than transketolase, and the resultant enhancement of this enzyme reaction would have caused increase in the FFA productivity in the ΔfaaA_tktOE strain.
In terms of possibility of the other bottleneck existence than PPP in FFA production, the malic enzyme reaction as described in left panel of Fig. is considered to be a potential bottleneck. The malic enzyme catabolizes conversion of malate to pyruvate in cytosol, and NADPH molecule is synthesized in the conversion similar to PPP. In fact, overexpression of a malic enzyme gene was confirmed to increase the lipid production amount at 2.5-fold in Mucor circinelloides.Citation25) Thus, overexpression of the endogenous malic enzyme gene of A. oryzae or heterologous expression of the gene from M. circinelloides would increase the FFA productivity in A. oryzae.
In terms of the phenotypic change of the ΔfaaA_tktOE strain, their hyphae were dispersed like pulp in the liquid culture, which was different from the aggregated cluster form of the A. oryzae wild-type strain and the parental ΔfaaA strain. The reason is unknown. The dispersed growth of hyphae was reported in the A. nidulans agsB gene disruptant.Citation26) Because agsB encodes an alpha-1,3-glucan synthase involved in the cell wall formation, the dispersed growth found in the agsB disruptant was deduced to be due to the change of cell wall structure. It is unclear whether the similar change of cell wall structure would have occurred in the ΔfaaA_tktOE strain. At least, the dispersed growth is considered to enable higher density cell culture of filamentous fungi in liquid culture,Citation26) which leads to more amount of fungal biomass yield. In fact, the ΔfaaA_tktOE strain yielded a 1.2-fold more amount of hyphae than the ΔfaaA strain in this study, which contributed to increase the FFA production yield at 1.7-fold together with the 1.4-fold increase in the FFA productivity.
Microscopic observation generally showed bent hyphae of the ΔfaaA_tktOE strain. In addition, spherical structures were frequently observed in the hyphae like stopping and redirecting the hyphae elongation. They were not observed in both parental ΔfaaA strain and the wild-type strain of A. oryzae. The similar spherical structures are reported in Aspergillus fumigatus when the hyphae are placed in the presence of micafungin, a fungicide inhibiting cell wall synthesis.Citation27) However, because there is no evidence showing cell wall impairment in the ΔfaaA_tktOE strain, it is difficult to consider that the bent hyphae and the spherical structures are due to cell wall impairment. In addition, because lipid droplets are considered to be composed of neutral lipids such as acylglycerols and sterol esters,Citation28) the spherical structures do not seem to correspond to lipid droplets and will not accumulate FFAs in them.
Authors contribution
Koichi Tamano conceived the experiments and wrote the manuscript. Ai Miura performed experiments and analyzed the data together with Koichi Tamano.
Disclosure statement
No potential conflict of interest was reported by the authors.
Supplemental material
The supplemental material for this paper is available at http://dx.doi.org/10.1080/09168451.2016.1162086.
TBBB_1162086_Supplementary_Material.zip
Download Zip (871.9 KB)References
- Lennen RM, Pfleger BF. Microbial production of fatty acid-derived fuels and chemicals. Curr. Opin. Biotechnol. 2013;24:1044–1053.10.1016/j.copbio.2013.02.028
- Liang MH, Jiang JG. Advancing oleaginous microorganisms to produce lipid via metabolic engineering technology. Prog. Lipid. Res. 2013;52:395–408.10.1016/j.plipres.2013.05.002
- Tamano K. Enhancing microbial metabolite and enzyme production: current strategies and challenges. Front. Microbiol. 2014;5:718.
- Delarue J, Guriec N. Opportunities to enhance alternative sources of long-chain n-3 fatty acids within the diet. Proc. Nutr. Soc. 2014;73:376–384.10.1017/S0029665114000123
- Peralta-Yahya PP, Zhang F, del Cardayre SB, et al. Microbial engineering for the production of advanced biofuels. Nature. 2012;488:320–328.10.1038/nature11478
- Ranganathan S1, Tee TW, Chowdhury A, et al. An integrated computational and experimental study for overproducing fatty acids in Escherichia coli. Metab. Eng. 2012;14:687–704.10.1016/j.ymben.2012.08.008
- Zheng Y, Li L, Liu Q, et al. Boosting the free fatty acid synthesis of Escherichia coli by expression of a cytosolic Acinetobacter baylyi thioesterase. Biotechnol. Biofuels. 2012;5:76.10.1186/1754-6834-5-76
- Chen L, Zhang J, Lee J, et al. Enhancement of free fatty acid production in Saccharomyces cerevisiae by control of fatty acyl-CoA metabolism. Appl. Microbiol. Biotechnol. 2014;98:6739–6750.10.1007/s00253-014-5758-8
- Leber C, Polson B, Fernandez-Moya R, et al. Overproduction and secretion of free fatty acids through disrupted neutral lipid recycle in Saccharomyces cerevisiae. Metab. Eng. 2015;28:54–62.10.1016/j.ymben.2014.11.006
- Runguphan W, Keasling JD. Metabolic engineering of Saccharomyces cerevisiae for production of fatty acid-derived biofuels and chemicals. Metab. Eng. 2014;21:103–113.10.1016/j.ymben.2013.07.003
- Wasylenko TM, Ahn WS, Stephanopoulos G. The oxidative pentose phosphate pathway is the primary source of NADPH for lipid overproduction from glucose in Yarrowia lipolytica. Metab. Eng. 2015;30:27–39.10.1016/j.ymben.2015.02.007
- Tamano K, Bruno KS, Karagiosis SA, et al. Increased production of fatty acids and triglycerides in Aspergillus oryzae by enhancing expressions of fatty acid synthesis-related genes. Appl. Microbiol. Biotechnol. 2013;97:269–281.10.1007/s00253-012-4193-y
- Tamano K, Bruno KS, Koike H, et al. Increased production of free fatty acids in Aspergillus oryzae by disruption of a predicted acyl-CoA synthetase gene. Appl. Microbiol. Biotechnol. 2015;99:3103–3113.10.1007/s00253-014-6336-9
- Sakuradani E, Ando A, Shimizu S, et al. Metabolic engineering for the production of polyunsaturated fatty acids by oleaginous fungus Mortierella alpina 1S-4. J. Biosci. Bioeng. 2013;116:417–422.10.1016/j.jbiosc.2013.04.008
- Liu X, Sheng J, Curtiss R 3rd. Fatty acid production in genetically modified cyanobacteria. Proc. Natl. Acad. Sci. U. S. A. 2011;108:6899–6904.10.1073/pnas.1103014108
- Ruffing AM. RNA-Seq analysis and targeted mutagenesis for improved free fatty acid production in an engineered cyanobacterium. Biotechnol. Biofuels. 2013;6:113.10.1186/1754-6834-6-113
- Kobayashi T, Abe K, Asai K, et al. Genomics of Aspergillus oryzae. Biosci. Biotechnol. Biochem. 2007;71:646–670.10.1271/bbb.60550
- Terabayashi Y, Sano M, Yamane N, et al. Identification and characterization of genes responsible for biosynthesis of kojic acid, an industrially important compound from Aspergillus oryzae. Fungal Genet. Biol. 2010;47:953–961.10.1016/j.fgb.2010.08.014
- Burdock GA, Carabin IG. Generally recognized as safe (GRAS): history and description. Toxicol Lett. 2004;150:3–18.10.1016/j.toxlet.2003.07.004
- Yamada O, Lee BR, Gomi K. Transformation system for Aspergillus oryzae with double auxotrophic mutations, niaD and sC. Biosci. Biotechnol. Biochem. 1997;61:1367–1369.10.1271/bbb.61.1367
- Tamano K, Satoh Y, Ishii T, et al. The β-1,3-exoglucanase gene exgA (exg1) of Aspergillus oryzae is required to catabolize extracellular glucan, and is induced in growth on a solid surface. Biosci. Biotechnol. Biochem. 2007;71:926–934.10.1271/bbb.60591
- Kitamoto N, Matsui J, Kawai Y, et al. Utilization of the TEF1-a gene (TEF1) promoter for expression of polygalacturonase genes, pgaA and pgaB , in Aspergillus oryzae. Appl. Microbiol. Biotechnol. 1998;50:85–92.10.1007/s002530051260
- Tamano K, Sano M, Yamane N, et al. Transcriptional regulation of genes on the non-syntenic blocks of Aspergillus oryzae and its functional relationship to solid-state cultivation. Fungal Genet. Biol. 2008;45:139–151.10.1016/j.fgb.2007.09.005
- Vongsangnak W, Olsen P, Hansen K, et al. Improved annotation through genome-scale metabolic modeling of Aspergillus oryzae. BMC Genomics. 2008;9:245.10.1186/1471-2164-9-245
- Zhang Y, Adams IP, Ratledge C. Malic enzyme: the controlling activity for lipid production? Overexpression of malic enzyme in Mucor circinelloides leads to a 2.5-fold increase in lipid accumulation. Microbiology. 2007;153:2013–2025.10.1099/mic.0.2006/002683-0
- Yoshimi A, Sano M, Inaba A, et al. Functional analysis of the α-1,3-glucan synthase genes agsa and agsb in Aspergillus nidulans: agsb is the major α-1,3-glucan synthase in this fungus. PLoS ONE. 2013;8:e54893.10.1371/journal.pone.0054893
- Nishiyama Y, Hasumi Y, Ueda K, et al. Effects of micafungin on the morphology of Aspergillus fumigatus. J. Electron. Microsc (Tokyo). 2005;54:67–77.
- Thiam AR, Farese RV Jr, Walther TC. The biophysics and cell biology of lipid droplets. Nat. Rev. Mol. Cell Biol. 2013;14:775–786.10.1038/nrm3699