Abstract
Glucolipids in Bacillus subtilis are synthesized by UgtP processively transferring glucose from UDP-glucose to diacylglycerol. Here we conclude that the abnormal morphology of a ugtP mutant is caused by lack of glucolipids, since the same morphology arises after abolition of glucolipid production by disruption of pgcA and gtaB, which are involved in UDP-glucose synthesis. Conversely, expression of a monoglucosyldiacylglycerol (MGlcDG) produced by 1,2-diacylglycerol 3-glucosyltransferase from Acholeplasma laidlawii (alMGS) almost completely suppressed the ugtP disruptant phenotype. Activation of extracytoplasmic function (ECF) sigmas (SigM, SigV, and SigX) in the ugtP mutant was decreased by alMGS expression, and was suppressed to low levels by MgSO4 addition. When alMGS and alDGS (A. laidlawii 1,2-diacylglycerol-3-glucose (1-2)-glucosyltransferase producing diglucosyldiacylglycerol (DGlcDG)) were simultaneously expressed, SigX activation was repressed to wild type level. These observations suggest that MGlcDG molecules are required for maintenance of B. subtilis cell shape and regulation of ECF sigmas, and DGlcDG regulates SigX activity.
Graphical abstract
The abnormal morphology of the B. subtilis ugtP mutant was suppressed by expression of heterologous MGlcDG synthase (left). Effect of heterologous glucolipids on activitiy of ECF sigmas (right).
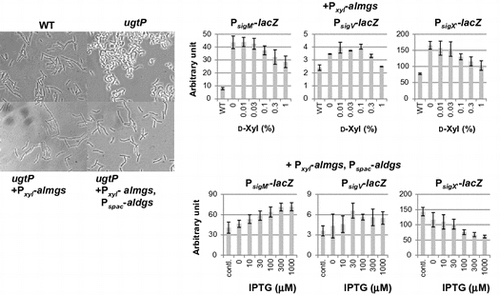
Lipid molecules in the cell membrane have multiple functions in vivo. For example, in Escherichia coli cells, phosphatidylethanolamine (PE) is not only important in maintaining the proper structure of LacY permease,Citation1) but also in cell division, where its capacity to form a nonbilayer is required.Citation2) The cell membrane in Bacillus subtilis consists of a greater variety of lipids compared with that of E. coli, and there are differences in function in the same lipid molecule between these bacteria. In E. coli, phosphatidylglycerol (PG) is dispensable in an lpp background,Citation3) whereas it is indispensable in B. subtilis.Citation4) However, the physiological functions of the other lipids are still unclear. In this paper, we focus on the functions of glucolipids in vivo for aspects of cell shape maintenance and regulation of extracytoplasmic function (ECF) sigmas, which are regulated by their cognate membrane-bound antisigma factors in B. subtilis.
B. subtilis 168 contains three kinds of glucolipid: monoglucosyldiacylglycerol (MGlcDG), diglucosyldiacylglycerol (DGlcDG), and triglucosyldiacylglycerol which amount to 1.2, 9.8, and 0.3% of total membrane lipids during the stationary phase.Citation5) These are synthesized processively by UgtP transferring glucose to diacylglycerol (DG) from UDP-glucose. DGlcDG in cell membranes is used as an anchor of lipoteichoic acids (LTA).Citation6) In Staphylococcus aureus, LTA is essential for normal cell growth.Citation7) Therefore, there might conceivably be a relationship between the phenotype of the ugtP mutant and LTA mutants. B. subtilis has four LTA synthases (the products of ltaS, yfnI, yqgS, and yvgJ). In quadruple mutant of LTA synthases, cell shape changes to abnormal but is different from that of the ugtP mutant.Citation8) It seems that not only DGlcDG, as a substrate of LTA synthesis, but other free glucolipids have many roles in the B. subtilis cell. However, it is still unclear what the exact biological functions of each molecular species of glucolipids are.
In this study, we show that disruption of pgcA and gtaB, which are involved in UDP-glucose synthesis, leads to a cell shape that is known to occur as a consequence of ugtP disruption. Since these mutants lack glucolipids, we conclude that the abnormal morphology of the ugtP mutant is almost certainly caused by the lack of glucolipids. However, this does not resolve the question as to what types of glucolipids are important for maintaining cell shape and/or regulation of ECF sigma activities. We tried to identify molecular species by expressing heterologous glucosyltransferase genes from Acholeplasma laidlawii in a B. subtilis ugtP mutant. We also investigated the effect of Mg2+ in the ugtP mutant on cell shapes and ECF sigma activities.
Materials and methods
Bacterial strains and plasmids
The bacterial strains and plasmids used in this study are listed in Table . The primers used are listed in Table . To amplify the objective DNA fragments, appropriate template DNA and primers were reacted with KOD plus Neo DNA polymerase (TOYOBO). The amplified fragments were then digested with appropriate restriction enzymes (New England Biolabs) and cloned into appropriate cloning sites of plasmids by transformation of E. coli DH5α. The ECF sigmas investigated in this study are autoregulated.Citation9) Therefore, to measure ECF sigma activities, the recognition sequences of the respective sigma genes were cloned into EcoRI-HindIII sites of pDG1663Citation10) which has lacZ downstream of the multiple cloning site and is integrated at the thrC locus into the B. subtilis genome. All plasmids constructed in this study were introduced into B. subtilis by transformation.Citation11,12)
Table 1. Strains and plasmids used in this study.
Table 2. Primers used in this study.
Growth, media, transformation and general techniques
Bacterial strains were grown aerobically with shaking at 37 °C. Cell growth was measured by TAITEC mini-photo 518R equipped with a 530 nm interference filter (TAITEC). Lennox Broth (LB) medium (Bacto Tryptone 10 g/1 L, yeast extract 5 g/L, NaCl 5 g/L, and 1.5% agar for solid media) was used for growing cells. Transformation of B. subtilis was carried out basically by the method of Spizizen.Citation12) Transformation of E. coli and DNA manipulation were performed as described previously.Citation13) The following antibiotics were added in the media: for selection of B. subtilis transformants: chloramphenicol (5 μg/mL), erythromycin (0.5 μg/mL), neomycin (5 μg/mL), and spectinomycin (100 μg/mL); for E. coli transformants: ampicillin (50 μg/mL). To induce almgs or aldgs in B. subtilis, d-xylose (up to 1%) or IPTG (up to 1000 μM) were used, respectively.
Observation of cell morphology using microscopy
1 mL aliquots of the cultures were harvested at OD530 ≈ 0.5 (log phase). After collection at room temperature, cell cultures were resuspended in 100 μL of LB medium. Cells were fixed on object slides coated with a layer of 1% (wt/vol) agarose gel in water. Images were viewed with an ECLIPSE E600 microscope (Nikon) and a Cooled CCD camera (ORCA-ER; Hamamatsu Photonics).
β-galactosidase assay for the promoter activities of ECF sigma factors
To determine the activity of each sigma, 0.9 ml aliquots of the culture were harvested at OD530 ≈ 0.5 (log phase), and the LacZ assay was performed by the method of MillerCitation14) with a minor modification as described previously.Citation15) All assays were repeated three times on different clones with three replicants each.
Lipid analysis
Lipids were extracted by the method of Bligh & DyerCitation16) and divided into two sets of samples, which were each subjected to thin layer chromatography (TLC) on silica gel (No. 60, Merck), developed with chloroform-methanol-acetic acid (13:5:2 [vol/vol/vol]). The TLC plates were dried and glucolipids were visualized on one TLC plate by spraying with orcinol sulfuric acid reagent (0.1 g orcinol in 45 ml of sulfuric acid-water-ethanol; 5:13:27[vol/vol/vol]) and baking for 10 min at 120 °C. Phospholipids were visualized on the second TLC plate by spraying with molybdenum blue reagent (Sigma).
Results and discussion
Lack of glucolipids leads to the abnormal morphology in B. subtilis cells
It has reported that ugtP mutant cells show abnormal morphology in previous studies.Citation17,18) We have also previously reported that ugtP mutant cells are bent and distended,Citation19) whereas Weart et al.Citation20) have reported that the sole distinguishing cell morphological feature of a ugtP mutant was that it was shorter than wild type. They proposed that UgtP regulates the timing of cell division by adjusting the assembly of FtsZ protein based on their finding of dissociation of FtsZ polymer in the presence of UgtP in vitro. At variance with this suggestion, we suspected that glucolipids are crucial for the maintenance of cell shape and that lack of glucolipids causes the phenotype. In order to demonstrate this, we disrupted pgcA and gtaB, which are involved in the UDP-glucose synthesis pathway. We analyzed lipid composition of the resulting cells and observed the cell morphology. As shown in Fig. (A), no glucolipids were detected in these strains, but ugtP is intact in these mutants. Furthermore, the two disruptants exhibited the same cell shape abnormalities as the ugtP mutant cells (Fig. (B)) and those in previous researches.Citation17,18) The phenotypic difference of ugtP mutants between ours and Weart et al.’s might be caused by genetic backgrounds of parental strains, as we used 168, whereas they used JH642. Indeed, JH642 genome has two deleted regions and 47 base differences compared with 168 genome.Citation21)
Fig. 1. pgcA and gtaB disruptants showed the same phenotype as the ugtP mutant.
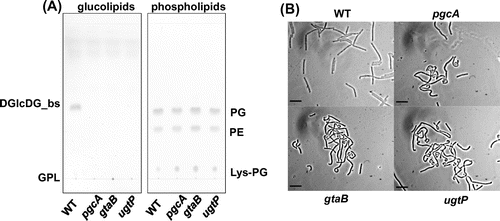
We predicted the active sites of UgtP by comparing it with E. coli MurG (N-acetylglucosaminyl transferase) using the ClustalW sequence alignment algorithmCitation22) to construct a nonfunctional UgtP by single amino acid substitution. We found that a MurG active site (histidine at position 18)Citation23) was conserved as histidine of UgtP (at the same position; data not shown). We changed this histidine to alanine in UgtP leading to complete loss of glycosyltransferase activity and constructed pMAT5 which harbors nonfunctional ugtP with Hisx6 at the C terminus (ugtPH18A; data not shown). The abnormal morphology was not suppressed when we introduced ugtPH18A into the ugtP mutant. The accumulation of DG is toxic to B. subtilis cellsCitation24) but the DG level increase by ugtP disruption did not affect the cell shape or growth, judging from a colony forming efficiency assay that was performed by serial dilution of WT and ugtP mutant.Citation24) In ugtP mutant cells, LTA is probably produced using DG as an alternative membrane anchor in the absence of DGlcDG, considering the case of S. aureus.Citation6,25) These results strongly suggest that it is indeed lack of glucolipids that leads to the abnormal morphology in B. subtilis cells.
Suppression of abnormal morphology of ugtP mutant by expression of heterologous glucolipid synthases
We wanted to know which of the three glucolipids was/were important to maintain cell shape. We introduced heterologous glucolipid synthases alMGS and alDGS from A. laidlawiiCitation26) into the B. subtilis ugtP mutant. The glucolipids produced by these heterologous genes, which have different glycosidic configuration than the ugtP products, were detected in the B. subtilis ugtP mutant (Fig. (A)).
Fig. 2. The abnormal morphology of the B. subtilis ugtP mutant was suppressed when heterologous MGlcDG synthase was expressed.
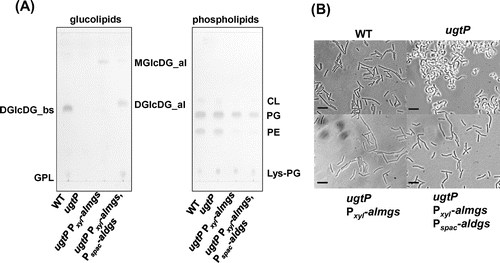
In A. laidlawii, glucolipids are synthesized by two enzymes, alMGS and alDGS, whereas they are synthesized processively by UgtP, a single enzyme, in B. subtilis. A further difference is that the structure of the glucose moiety in the glucolipids synthesized by alMGS and alDGS is not exactly the same. In B. subtilis, β-glucose binds to DG, and then the next glucose is bound to MGlcDG via β-1,6 linkage by UgtP.Citation27) In A. laidlawii, α-glucose is linked to DG by alMGS, and then the next glucose is linked to MGlcDG through an α-1,2 bond by alDGS. Amino acid identities between UgtP and alMGS, UgtP and alDGS, computed using the EMBOSS Needle pairwise sequence alignment algorithm,Citation28) were 17.5 and 15.7%, respectively, indicating that they are not orthologs. This assured us that the introduction of alMGS and alDGS into the ugtP mutant was a valid method to investigate the function of each glucolipid, although there are some differences in the structure between B. subtilis and A. laidlawii glucolipids.
Surprisingly, the cell shape phenotype was already nearly completely suppressed by expression of alMGS in the ugtP mutant (Fig. (B)), although DGlcDG is presumed to be important to maintain cell shape because of its abundance in the membrane of wild type cells. When alMGS and alDGS were expressed simultaneously, the cells attained an almost normal cell shape in spite of the decrease in heterologous MGlcDG (Fig. (A), and (B)). Furthermore, the cells expressing heterologous glucolipids exhibited a decrease in PE content (Fig. (A)). This phenomenon may be caused by a shift of the membrane lipid synthesis flow to DG synthesis because DG is consumed when the heterologous glucolipids are produced. Actually, in B. subtilis, by disruption of pssA, which is responsible for the production of PE, production of glucolipids is accelerated and there are no defects in the absence of PE,Citation15,29) whereas in E. coli, PE is required in cell division for its capacity to form a nonbilayer.Citation2) These results suggest that heterologous MGlcDG is sufficient to maintain normal cell shape in B. subtilis.
ECF sigma activities were affected by induction of heterologous glucosyltransferases
We have previously reported that the ECF sigmas, SigM, SigV and SigX, were activated in the ugtP mutant cells.Citation15) We therefore decided to investigate the effect of heterologous glucolipid production on the activities of ECF sigmas. We found that when alMGS was expressed, the activities of these ECF sigmas decreased (Fig. (A)). Interestingly, SigX activity alone was decreased to wild type level when alDGS was induced, whereas SigM and SigV activities increased (Fig. (B)). In wild type cells, when alMGS and alDGS were simultaneously expressed, SigM and SigV activities also increased with increasing inducer concentration (Fig. ). This indicates that accumulation of heterologous membrane proteins might be sensed as a stress by the cells.
Fig. 3. Effect of heterologous glucolipids on activitiy of ECF sigmas in the B. subtilis ugtP mutant.
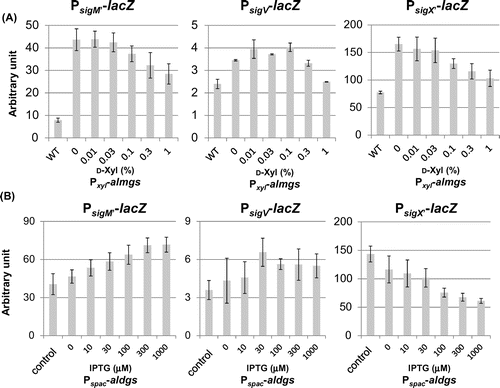
Fig. 4. Effect of heterologous glucolipids production on activitiy of SigM and SigV in the B. subtilis wild type.
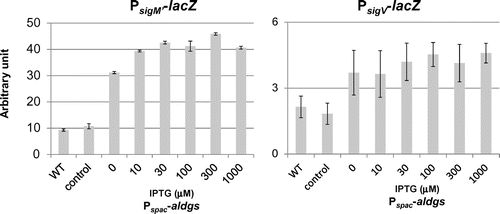
Fig. 5. Effect of ugtPH18A expression and Mg2+ addition on activities of ECF sigmas in B. subtilis ugtP mutant.
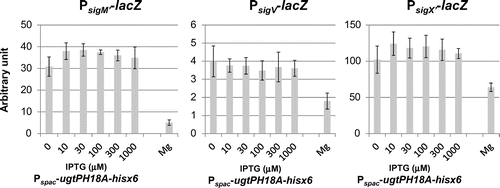
Fig. 6. Effect of expression of heterologous glucolipids on activitiy of SigV and SigX in the B. subtilis without their anit-sigma factors.
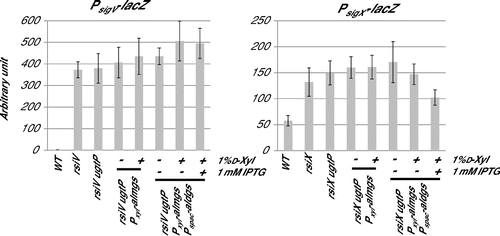
In order to confirm that the glucolipids, not the protein, are important for the regulation of ECF sigmas, we next introduced ugtPH18A-hisx6 into the ugtP mutant. We confirmed the existence of this UgtP variant by western blotting (data not shown), and there were no effects on the repression of the activation of ECF sigmas (Fig. ).
We also investigated the effect of magnesium ion on activation of ECF sigmas in the ugtP mutant. The abnormal morphology is known to be suppressed by the addition of magnesium ion to the growth mediumCitation19) We found that activation of ECF sigmas in the ugtP mutant was strongly suppressed when 10 mM MgSO4 was added (Fig. ).
We then investigated the effect of glucolipids on ECF sigma activities in conditions of their anti-sigma disruption, to reveal whether glucolipids affect ECF sigmas via anti-sigmas function. SigM has two anti-sigmas, YhdL and YhdK. yhdL is an essential gene,Citation30) because constitutive activation of SigM is toxic for B. subtilis cells. Therefore, we tried to use disruption of another anti-sigma, yhdK. However, in yhdK disruptant, SigM activity was unstable, as spontaneous inactivation of SigM was observed, for which we do not know the reason. We further investigated the cases of SigV and SigX. Fig. shows that the activities of SigV and SigX increased significantly by disruption of their anti-sigma genes, and there were no effects of lack of glucolipids and production of heterologous MGlcDG on ECF sigma activities in anti-sigma disrupted cells. Surprisingly, SigX activity decreased when DGlcDG_al was expressed in spite of disruption of its anti-sigma factor. This implies the existence of a regulatory system of SigX activity which is independent on RsiX.
These results suggest that glucolipids, especially MGlcDG, may be important for the regulation of these ECF sigmas. The analysis of the influence of glucolipids produced by UgtP on the activity of ECF sigmas in an E. coli heterologous expression system also suggested that glucolipids alone may be sufficient to strongly affect the activity of SigM and SigV.Citation31)
Lysozyme activates SigV by stimulation of the regulated intramembrane proteolysis of RsiV, the anti-sigma of SigV,Citation32) while MGlcDG may affect anti-sigmas of SigM and SigV via the in transmembrane region. In other words, the activation of ECF sigmas in the ugtP mutant may not be accompanied by regulated intramembrane proteolysis. Actually, we observed that RsiV remained intact in ugtP mutant cells (Seki et al., unpublished result). It has been suggested that an appropriate length of transmembrane region is required for proper integration of anti-sigma into the membrane.Citation33) DesK, a two-component sensor kinase in B. subtilis works as a thermosensor by sensing the thickness of the cell membrane.Citation34) Thus, the conditions of the cell membrane, such as the thickness, surface charge, etc., that affect cell membrane integrity may affect the function of membrane-anchored anti-sigmas. The magnesium ion may compensate for the lack of glucolipids in the maintenance of cell membrane integrity. On the other hand, DGlcDG may be specifically important for regulation of SigX. DGlcDG is the anchor for LTA in wild type cells. Indeed, production of glucophospholipid (GPL), a precursor of LTA,Citation25) was detected when heterologous DGlcDG was produced in the ugtP mutant (Fig. (A)). Since the induction of yqgS and yvgJ is dependent on SigX,Citation15) there may be a relationship between the LTA synthesis and SigX activity. Alternatively, GPL may be a key component of LTA in regulation of SigX.
In this study, we report that the abnormal morphology of a ugtP mutant is caused by lack of glucolipids. Production of heterologous MGlcDG in the ugtP mutant suppressed the abnormal morphology and repressed activation of SigM, SigV, and SigX. Furthermore, when heterologous alMGS and alDGS were expressed simultaneously, the SigX activity was specifically suppressed to wild type level. This indicates that MGlcDG is required for maintaining normal cell shape and controls the activities of certain ECF sigmas via membrane-anchored anti-sigma factors. Addition of magnesium ion allows the cell membrane to attain a condition similar to the condition it has when containing glucolipids. We suggest that DGlcDG and/or LTA may be involved in the regulation of SigX. Thus, each glucolipid species plays many different roles in B. subtilis cells. Further studies should reveal the biological functions of each glucolipid at the molecular level.
Author contributions
S.M. designed the study and wrote the manuscript. K.M. and H·H. contributed to the development of the manuscript. S.M. and T.S. performed the experiments and analyzed the data. All authors have read and approved the final manuscript.
Disclosure statement
No potential conflict of interest was reported by the authors.
Funding
This study was supported by a Japan Society for the Promotion of Science (JSPS KAKENHI) [grant number 15K18664].
Acknowledgments
We are greatly thankful to Drs. Åke Wieslander and Kei Asai for providing E. coli strain AW153 harboring pET15b-almgs and pET15b–aldgs, and pDG1663 and B. subtilis strain ASK441, respectively.
References
- Bogdanov M, Heacock P, Guan Z, et al. Plasticity of lipid-protein interactions in the function and topogenesis of the membrane protein lactose permease from Escherichia coli. Proc. Natl. Acad. Sci. U. S. A. 2010;107:15057–15062.10.1073/pnas.1006286107
- Wikström M, Xie J, Bogdanov M, et al. Monoglucosyldiacylglycerol, a Foreign Lipid, Can Substitute for Phosphatidylethanolamine in Essential Membrane-associated Functions in Escherichia coli. J. Biol. Chem. 2004;279:10484–10493.10.1074/jbc.M310183200
- Kikuchi S, Shibuya I, Matsumoto K. Viability of an Escherichia coli pgsA null mutant lacking detectable phosphatidylglycerol and cardiolipin. J. Bacteriol. 2000;182:371–376.10.1128/JB.182.2.371-376.2000
- Hashimoto M, Takahashi H, Hara Y, et al. Induction of extracytoplasmic function sigma factors in Bacillus subtilis cells with membranes of reduced phosphatidylglycerol content. Genes Genet. Syst. 2009;84:191–198.10.1266/ggs.84.191
- Kawai F, Hara H, Takamatsu H, et al. Cardiolipin enrichment in spore membranes and its involvement in germination of Bacillus subtilis Marburg. Genes Genet. Syst. 2006;81:69–76.10.1266/ggs.81.69
- Kiriukhin MY, Debabov DV, Shinabarger DL, et al. Biosynthesis of the glycolipid anchor in lipoteichoic acid of Staphylococcus aureus RN4220: role of YpfP, the diglucosyldiacyl-glycerol synthase. J. Bacteriol. 2001;183:3506–3514.10.1128/JB.183.11.3506-3514.2001
- Oku Y, Kurokawa K, Matsuo M, et al. Pleiotropic roles polyglycerolphosphate synthase of lipoteichoic acid in growth of Staphylococcus aureus cells. J. Bacteriol. 2009;191:141–151.10.1128/JB.01221-08
- Kiriyama Y, Yazawa K, Tanaka T, et al. Localization and expression of the Bacillus subtilis DL-endopeptidase LytF are influenced by mutations in LTA synthases and glycolipid anchor synthetic enzymes. Microbiology. 2014;160:2639–2649.10.1099/mic.0.080366-0
- Asai K, Yamaguchi H, Kang CM, et al. DNA microarray analysis of Bacillus subtilis sigma factors of extracytoplasmic function family. FEMS Microbiol. Lett. 2003;220:155–160.10.1016/S0378-1097(03)00093-4
- Guérout-Fleury AM, Frandsen N, Stragier P. Plasmids for ectopic integration in Bacillus subtilis. Gene. 1996;180:57–61.10.1016/S0378-1119(96)00404-0
- Matsuoka S, Arai T, Murayama R, et al. Identification of the nonA and nonB loci of Bacillus subtilis Marburg permitting the growth of SP10 phage. Genes Gent. Syst. 2004;79:311–317.10.1266/ggs.79.311
- Anagnostopoulos C, Spizizen J. Requirements for transformation in Bacillus subtilis. J. Bacteriol. 1961;81:741–746.
- Matsuoka S, Yukawa H, Inui M, et al. Synergistic interaction of Clostridium cellulovorans cellulosomal cellulases and HbpA. J. Bacteriol. 2007;189:7190–7194.10.1128/JB.00842-07
- Miller JH. A short course in bacterial genetics: a laboratory manual and handbook for Escherichia coli and related bacteria. Cold Spring Harbor, NY: Cold Spring Harbor Laboratory Press; 2000.
- Hashimoto M, Seki T, Matsuoka S, et al. Induction of extracytoplasmic function sigma factors in Bacillus subtilis cells with defects in lipoteichoic acid synthesis. Microbiology. 2013;159:23–35.10.1099/mic.0.063420-0
- Bligh EG, Dyer WJ. A rapid method for total lipid extraction and purification. Can. J. Biochem. Physiol. 1959;37:911–917.10.1139/o59-099
- Lazarevic V, Soldo B, Médico N, et al. Bacillus subtilis alpha-phosphoglucomutase is required for normal cell morphology and biofilm formation. Appl. Environ. Microbiol. 2005;71:39–45.10.1128/AEM.71.1.39-45.2005
- Salzberg Li, Helmann JD. Phenotypic and transcriptomic characterization of Bacillus subtilis mutants with grossly altered membrane composition. J. Bacteriol. 2008;190:7797–7807.10.1128/JB.00720-08
- Matsuoka S, Chiba M, Tanimura Y, et al. Abnormal morphology of Bacillus subtilis ugtP mutant cells lacking glucolipids. Genes Genet. Syst. 2011;86:295–304.10.1266/ggs.86.295
- Weart R, Lee A, Chien AC, et al. A metabolic sensor governing cell size in bacteria. Cell. 2007;130:335–347.10.1016/j.cell.2007.05.043
- Srivatsan A, Han Y, Peng J, et al. High-precision, whole-genome sequencing of laboratory strains facilitates genetic studies. PLoS Genet. 2008;4:e1000139.10.1371/journal.pgen.1000139
- Thompson JD, Higgins DG, Gibson TJ. CLUSTAL W: improving the sensitivity of progressive multiple sequence alignment through sequence weighting, position-specific gap penalties and weight matrix choice. Nucleic Acids Res. 1994;22:4673–4680.10.1093/nar/22.22.4673
- Crouvoisier M, Auger G, Blanot D, et al. Role of the amino acid invariants in the active site of MurG as evaluated by site-directed mutagenesis. Biochimie. 2007;89:1498–1508.10.1016/j.biochi.2007.06.011
- Matsuoka S, Hashimoto M, Kamiya Y, et al. The Bacillus subtilis essential gene dgkB is dispensable in mutants with defective lipoteichoic acid synthesis. Genes Genet. Syst. 2011;86:365–376.10.1266/ggs.86.365
- Wörmann ME, Corrigan RM, Simpson PJ, et al. Enzymatic activities and functional interdependencies of Bacillus subtilis lipoteichoic acid synthesis enzymes. Mol. Microbiol. 2011;79:566–583.10.1111/j.1365-2958.2010.07472.x
- Wikström M, Kelly AA, Georgiev A, et al. Lipid-engineered Escherichia coli membranes reveal critical lipid headgroup size for protein function. J. Bio. Chem. 2009;284:954–965.10.1074/jbc.M804482200
- Jorasch P, Wolter FP, Zhäringer U, et al. A UDP glucosyltransferase from Bacillus subtilis successively transfers up to four glucose residues to 1,2-diacylglycerol: expression of ypfP in Escherichia coli and structural analysis of its reaction products. Mol. Microbiol. 1998;29:419–430.10.1046/j.1365-2958.1998.00930.x
- Needleman SB, Wunsch CD. A general method applicable to the search for similarities in the amino acid sequence of two proteins. J. Mol. Biol. 1970;48:443–453.10.1016/0022-2836(70)90057-4
- Matsumoto K, Okada M, Horikoshi Y, et al. Cloning, sequencing, and disruption of the Bacillus subtilis psd gene coding for phosphatidylserine decarboxylase. J. Bacteriol. 1998;180:100–106.
- Kobayashi K, Ehrlich SD, Albertini A, et al. Essential Bacillus subtilis genes. Proc. Natl. Acad. Sci. U. S. A. 2003;100:4678–4683.10.1073/pnas.0730515100
- Seki T, Mineshima R, Hashimoto M, et al. Repression of the activities of two extracytoplasmic function σ factors, σM and σV, of Bacillus subtilis by glucolipids in Escherichia coli cells. Genes Genet. Syst. 2015;90:109–114.10.1266/ggs.90.109
- Hastie JL, Williams KB, Ellermeier CD. The activity of σV, an extracytoplasmic function σ factor of Bacillus subtilis, is controlled by regulated proteolysis of the anti-σ factor RsiV. J Bacteriol. 2013;195:3135–3144.10.1128/JB.00292-13
- Yano K, Inoue H, Mori H, et al. Heterologous expression of the Oceanobacillus iheyensis SigW and its anti-protein RsiW in Bacillus subtilis. Biosci. Biotechnol. Biochem. 2011;75:966–975.10.1271/bbb.110035
- Inda ME, Vandenbranden M, Fernández A, et al. A lipid-mediated conformational switch modulates the thermosensing activity of DesK. Proc. Natl. Acad. Sci. U. S. A. 2014;111:3579–3584.10.1073/pnas.1317147111
- Yoshimura M, Asai K, Sadaie Y, et al. Interaction of Bacillus subtilis extracytoplasmic function (ECF) sigma factors with the N-terminal regions of their potential anti-sigma factors. Microbiology. 2004;150:591–599.10.1099/mic.0.26712-0
- Yee LM, Matsuoka S, Yano K, et al. Inhibitory effect of prophage SPβ fragments on phage SP10 ribonucleotide reductase function and its multiplication in Bacillus subtilis. Genes Genet. Syst. 2011;86:7–18.10.1266/ggs.86.7
- Kim L, Mogk A, Schumann W. A xylose-inducible Bacillus subtilis integration vector and its application. Gene. 1996;181:71–76.10.1016/S0378-1119(96)00466-0
- Morimoto T, Loh PC, Hirai T, et al. Six GTP-binding proteins of the Era/Obg family are essential for cell growth in Bacillus subtilis. Microbiology. 2002;148:3539–3552.10.1099/00221287-148-11-3539
- Ogura M, Yamaguchi H, Yoshida K, et al. DNA microarray analysis of Bacillus subtilis DegU, ComA and PhoP regulons: an approach to comprehensive analysis of B.subtilis two-component regulatory systems. Nucleic Acids Res. 2001;29:3804–3813.10.1093/nar/29.18.3804