Abstract
Avt3p, a vacuolar amino acid exporter (656 amino acid residues) that is important for vacuolar amino acid compartmentalization as well as spore formation in Schizosaccharomyces pombe, has an extremely long hydrophilic region (approximately 290 amino acid residues) at its N-terminus. Because known functional domains have not been found in this region, its functional role was examined with a deletion mutant avt3(∆1–270) expressed in S. pombe avt3∆ cells. The deletion of this region did not affect its intracellular localization or vacuolar contents of basic amino acids as well as neutral ones. The defect of avt3Δ cells in spore formation was rescued by the expression of avt3+ but was not completely rescued by the expression of avt3(∆1–270). The N-terminal region is thus dispensable for the function of Avt3p as an amino acid exporter, but it is likely to be involved in the role of Avt3p under nutritional starvation conditions.
Graphical Abstract
The N-terminal long hydrophilic region of Schizosaccharomyces pombe Avt3p is suggested to be dispensable for the function as an amino acid exporter.
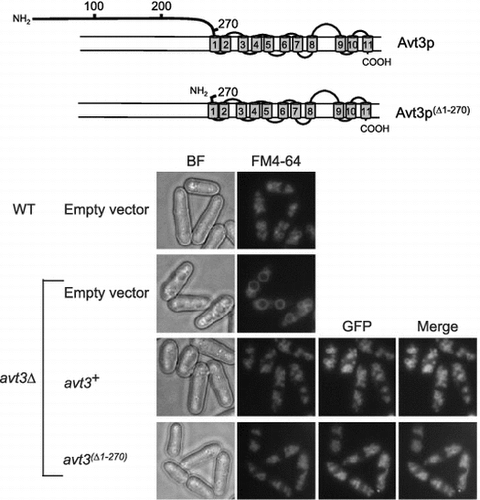
Yeast vacuoles are large organelles that function as a digestive compartment and also serve as a major storage compartment for various amino acids.Citation1−3) Vacuoles contain approximately 70‒90% of cellular basic amino acids, whereas some amino acids are almost excluded from the organelles; approximately 80% of alanine remains in the cytosol of Schizosaccharomyces pombe,Citation4) and 90% of acidic amino acids are localized in the cytosol of Saccharomyces cerevisiae.Citation2) Such differential distribution of amino acids implies the presence of specific transport machineries across the vacuolar membrane. These activities are most likely to be co-transport of H+ and amino acids, which is driven by a proton electrochemical gradient generated by the vacuolar-type H+-ATPase.Citation5−7)
To date, several gene families for vacuolar amino acid transporters have been identified and characterized in S. cerevisiae by experiments using isolated vacuolar membrane vesicles.Citation8−13) Avt3p (YKL146w) and the related Avt4p (YNL101w) of S. cerevisiae belong to the AVT family in the amino acid/auxin permease family, and were first reported to be involved in the export of glutamine and tyrosine from vacuoles of S. cerevisiae.Citation10) Recently, by investigating transport activity using vacuolar membrane vesicles and analyzing vacuolar amino acid contents, we found that both Avt3p and Avt4p show broad substrate specificity for neutral amino acids and Avt4p also recognizes basic amino acids, not only neutral ones.Citation13) Under nitrogen starvation, cells utilize the vacuolar amino acid pools as a nitrogen source.Citation14) Exporters of vacuolar amino acids are important for the recycling of amino acids for de novo protein synthesis or metabolic pathways, suggesting that vacuolar amino acid pools are essential for the maintenance of cellular function.Citation14−16) Mukaiyama et al. indicated that S. pombe Avt3p (SPAC3H1.09c), a homolog of S. cerevisiae Avt3p and Avt4p, is involved in spore formation of S. pombe cells.Citation17) Recently, we observed that vacuolar contents of amino acids were greatly increased in S. pombe avt3∆ cells and were lowered by the expression of the avt3+ gene, indicating that Avt3p is involved in vacuolar amino acid export and is important for vacuolar compartmentalization of amino acids in S. pombe cells.Citation18) It is quite difficult to characterize the genes that encode vacuolar transporters in S. pombe using isolated vacuolar membrane vesicles because the vacuoles are too small and a procedure to purify vacuolar membrane vesicles from S. pombe cells has not been established. We have succeeded in the specific expression of S. pombe avt3+ in the vacuolar membrane of S. cerevisiae and reported that Avt3p of S. pombe is a vacuolar transporter involved in the extrusion of amino acids from vacuoles.Citation18) Although the amino acid sequence of S. pombe Avt3p is more closely related to that of S. cerevisiae Avt3p than Avt4p, the substrate specificity of S. pombe Avt3p is similar to that of Avt4p because S. pombe Avt3p transports both basic and neutral amino acids.Citation18) Relatively fewer homologs of vacuolar amino acid transporters are found in the genome of S. pombe than in that of S. cerevisiae, implying less redundancy among their functions. Therefore, S. pombe has an advantage for understanding the physiological role of vacuolar amino acid transporters.
Very recently, we found that Fusarium oxysporum Avt3p transports alanine and glutamine.Citation19) The Avt3/Avt4 homologs, including S. pombe Avt3p as well as S. cerevisiae Avt3p and Avt4p, are widely distributed in yeast and fungi and are characterized by the presence of 11 putative transmembrane helices and a large hydrophilic region in the N-terminal region.Citation13,19) Such long hydrophilic domains in transporters have been suggested to play important roles in their regulation via organellar distribution, phosphorylation, and interaction with other proteins.Citation20−23) In this study, we investigated the role of the N-terminal hydrophilic region in the activity of S. pombe Avt3p by examining the effect of its deletion.
Materials and methods
Strains and media
The S. pombe strains used in this study are the wild-type ARC039 (h− leu1-32 ura4-C190T) and KJ100-7B (h90 leu1-32 ura4-D18) and their avt3+ gene disruptants isolated in previous studies.Citation17) Cells were aerobically grown at 30 °C in a standard medium (YES) and a synthetic minimal medium (EMM) as described elsewhere.Citation24) S. pombe cells were transformed by the lithium acetate method.Citation25) Other manipulations in yeast were done using standard procedures.Citation26,27)
Plasmid construction and fluorescence microscopy
To construct a plasmid for the expression of the deletion mutant avt3(∆1–270), the open reading frame of avt3+ was amplified by PCR using primers 5′-CCTAGGATCCTACGCCTGGAAATGCTTCAAAC-3′ and 5′-CCAACGCCGGCGCTAAAAAAATGTCATGTACGTAG-3′ and subcloned into pTN54, a derivative of the thiamine-repressible expression vector pREP41,Citation28) resulting in pTN54–avt3(∆1–270). S. pombe cells transformed with pTN54–avt3+ Citation18) or pTN54–avt3(∆1–270) were grown in EMM medium without leucine and thiamine at 30 °C for 20 h and were then labeled with the lipophilic dye FM4-64 (Invitrogen), as described previously.Citation29) Cells were observed under an IX71 fluorescence microscope (Olympus, Tokyo, Japan) equipped with a cooled charge-coupled device camera (ImagEMC9100-13; Hamamatsu, Japan). Images were acquired using Metamorph software (Universal Imaging, West Chester, PA).
Analysis of amino acid contents in the vacuolar pools of S. pombe cells
Vacuolar fractions of S. pombe cells were prepared using the cupric ion treatment method.Citation4) The amino acid contents in the fractions were analyzed with an amino acid analyzer (Hitachi L-8800).
Immunoblot analysis
Membrane fractions were prepared from cell lysates containing 100 μg of protein as described beforeCitation29) and applied to an SDS-PAGE gel containing 8% polyacrylamide and were then analyzed by immunoblotting using an anti-green fluorescent protein (GFP) antibody (JL-8, Clontech). Immunoblot signals were detected by the ECL system (GE Healthcare).
Estimation of sporulation efficiency
Cells grown in the EMM medium without leucine and thiamine to the logarithmic phase were transferred to a sporulation medium (MEA),Citation24) and were subsequently incubated at 28 °C. Cells were collected and suspended in water and observed as described above. Sporulation efficiency was measured after 3 days of incubation in the MEA medium as the ratio of tetrads to the number of cells.
Results
Effect of deletion of the N-terminal region on subcellular localization of Avt3p in S. pombe cells
Fig. (A) shows a topology model of S. pombe Avt3p predicted by the SOSUI program (http://harrier.nagahama-i-bio.ac.jp/sosui/sosui_submit.html). Avt3p has a long hydrophilic region at its N-terminus (1–290 residues) like other Avt3/Avt4 homologs,Citation13,19) and several residues in this N-terminal region have been indicated to be modified by phosphorylation,Citation30,31) but we could not find any remarkable motifs/domains in these sequences. Therefore, to understand the function and transport mechanism of Avt3/Avt4 homologs, we constructed a deletion mutant of S. pombe Avt3p, in which the N-terminal region was almost completely deleted (Avt3p(∆1–270), Fig. (A)), and its activity was examined. Although the expression level of GFP-tagged avt3(∆1–270) was slightly lower than that of GFP-tagged wild-type avt3+, the corresponding Avt3p proteins were certainly expressed in S. pombe avt3∆ cells (Fig. (B)). Both GFP–Avt3p and GFP–Avt3p(∆1–270) were exclusively localized at the vacuolar membrane in S. pombe avt3∆ cells (Fig. ), suggesting that the deletion of the N-terminal hydrophilic region did not affect the intracellular localization of Avt3p. It is noted that the enlargement of vacuoles observed in avt3∆ cells Citation18) was recovered by the overexpression of GFP–Avt3p(∆1–270) as well as GFP–Avt3p, implying that GFP–Avt3p(∆1–270) as well as GFP–Avt3p complements some change in the physiological status of vacuoles caused by the deletion of avt3+.
Fig. 1. Topology models of Avt3p and immunoblot analysis of GFP–Avt3p and GFP–Avt3p(Δ1–270) expressed in S. pombe avt3∆ cells.
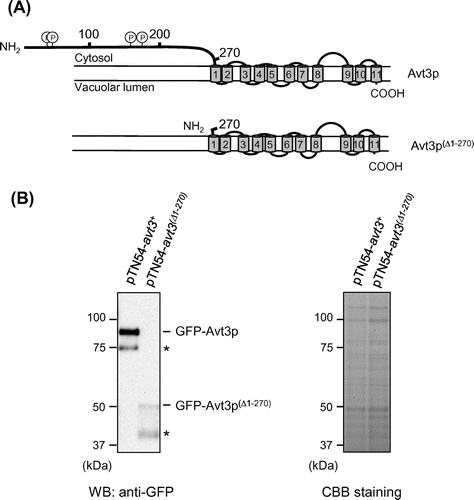
Effect of deletion of the N-terminal region on amino acid composition in vacuolar pools of S. pombe cells
Next, we examined the vacuolar amino acid pools of S. pombe wild-type and avt3∆ cells, and avt3∆ cells overexpressing either avt3+ or avt3 (∆1–270). As shown in Fig. , basic amino acids (lysine, histidine, and arginine) were highly accumulated in the vacuoles of wild-type cells and were clearly further increased in avt3∆ cells. The increased levels of these amino acids in the vacuolar fraction of avt3∆ cells were remarkably reduced by the overexpression of avt3+. The levels of a number of neutral amino acids in the vacuoles, such as threonine, glutamine, alanine, and proline, were also decreased by the overexpression of avt3+, as we reported in a previous study.Citation18) The overexpression of avt3(Δ1–270) also reduced the levels of these basic and neutral amino acids in the vacuoles (Fig. ). Although the expression level of avt3(∆1–270) was slightly lower than that of avt3+ in avt3Δ cells (Fig. ), the decrease in levels of several amino acids in the vacuoles of cells overexpressing avt3(∆1–270) were more remarkable than those in the cells overexpressing avt3+. The amount of threonine, serine, glutamine, glycine, alanine, tyrosine, proline, histidine, and arginine in cells overexpressing avt3(∆1–270) was 45‒65% of that in cells overexpressing avt3+. These results suggest that the Avt3p(∆1–270) mutant transporter is fully or more active when compared with wild-type Avt3p and that the N-terminal region of Avt3p is dispensable for the export of amino acids from vacuoles.
Fig. 2. Effect of deletion of the N-terminal region of Avt3p on its subcellular localization and vacuolar morphology in S. pombe cells.
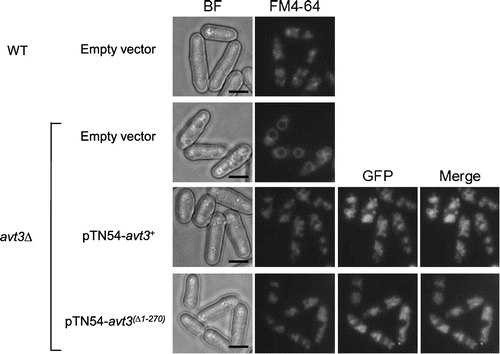
Effect of deletion of the N-terminal region of Avt3p on spore formation of S. pombe cells
Vacuolar amino acids have been suggested to be recycled for maintaining protein synthesis under nitrogen starvation conditions.Citation14,15) It has been reported that S. pombe Avt3p is required for efficient spore formation, which is induced in response to nitrogen starvation.Citation17) Recently, Avt3p and Avt4p in S. cerevisiae were also shown to be involved in spore formation.Citation12) Here, we examined the effect of N-terminal deletion on sporulation efficiency in S. pombe cells (Fig. ). As shown in Fig. (A), both GFP–Avt3p and GFP–Avt3p(Δ1–270) proteins were exclusively localized at the vacuolar membrane 24 and 48 h after transferring the cells to the sporulation medium (Fig. (A)). This suggested that the localization of Avt3p was not affected by the presence of its long N-terminal region under sporulation condition. In addition, both GFP–avt3+ and GFP–avt3(Δ1–270) were certainly expressed under the condition, by assessing from the fluorescence intensity of GFP-fused proteins. After incubation for 3 days in the sporulation medium, the wild-type homothallic strain KJ100-7B carrying pREP41 (empty vector) sporulated with an efficiency of approximately 75%, whereas its atg1∆ mutant cells, which show defects in the process of autophagy, showed almost no sporulation.Citation17) Corresponding to the previous results,Citation17) the sporulation efficiency was decreased to approximately 45% by the disruption of avt3+. The sporulation efficiency was almost completely rescued by the introduction of a plasmid pTN54–avt3+ into avt3∆ cells. These results suggest that avt3+ is important for the adaptation to nutrient starvation in S. pombe cells. The introduction of a plasmid pTN54–avt3(∆1–270) significantly recovered the sporulation efficiency of avt3∆ cells, but the rescue was incomplete, even though the activity of Avt3p(∆1–270) was suggested to be almost equal to or higher than wild-type Avt3p (Figs. and ). From this result, we suppose that the N-terminal region has a physiological function under nutrient starvation conditions.
Fig. 3. Effect of deletion of the N-terminal region of Avt3p on vacuolar amino acid composition in S. pombe cells.
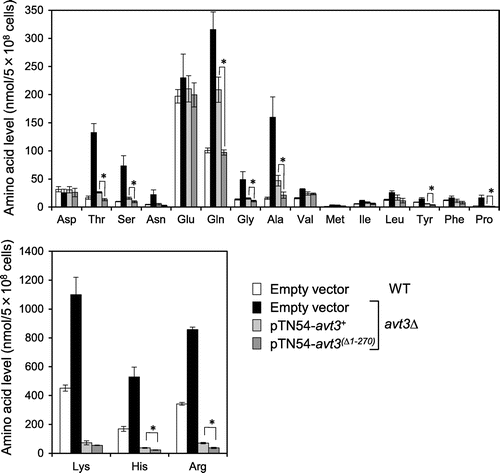
Discussion
In this study, we investigated the effects of deletion of the N-terminal region of Avt3p on vacuolar amino acid compartmentalization and spore formation in S. pombe cells. Our results showed that amino acid levels in the vacuoles in avt3∆ cells was decreased by the overexpression of mutant avt3(∆1–270) as well as wild-type avt3+ and that the vacuolar size of strains expressing avt3+ or avt3(∆1–270) were almost identical (Figs. and ). We previously indicated that vacuoles are larger in avt3Δ cells than in wild-type cells.Citation18) We postulate that the unusual levels of vacuolar amino acids in the avt3Δ cells affected vacuolar morphology. Although further experiments are needed to determine how Avt3p is involved in vacuolar morphology, these results indicate that the N-terminal region of Avt3p is not necessary for the export activity of amino acids from vacuoles to the cytosol (Figs. and ). In addition, Avt3p(∆1–270) is likely to be functional with higher export activity than wild-type Avt3p because although the cellular level of Avt3p(∆1–270) was slightly lower than that of wild-type Avt3p (Fig. ), the overexpression of avt3(∆1–270) reduced the amount of vacuolar amino acids even more than that of avt3+ (Fig. ).
Our results indicated that the N-terminal-deleted Avt3p was functional in the export of amino acids from vacuoles (Figs. and ), but the overexpression of avt3(∆1–270) did not completely rescue the sporulation efficiency of avt3∆ cells (Fig. ), suggesting that the N-terminal region has a physiological function under nutrient starvation conditions. It is known that large hydrophilic regions have regulatory roles in the function of transporters. In S. cerevisiae, the glucose signaling regulator Mth1p has been indicated to negatively regulate the activity of the Na+/H+ antiporter Nhx1p in response to extracellular carbon sources, and recently, evidence of binding between Mth1p and the long C-terminal hydrophilic region of Nhx1p was reported.Citation23) In this regard, it is interesting that the activity of Avt3p(∆1–270) is likely to be higher than that of wild-type Avt3p. The C-terminal region of the plasma membrane transporter Nhx1p and the N-terminal region of the vacuolar transporter Avt3p are considered to be cytoplasmically oriented (Fig. (A)).Citation23) It is possible that unknown cytoplasmic factor(s) bind to the N-terminal region of Avt3p to mask the activity of Avt3p. In fact, we found that the N-terminal deletion mutant of S. cerevisiae Avt4p showed higher activity in exporting amino acids from isolated vacuolar membrane vesicles (Sekito, T., et al., unpublished data). We postulate that appropriate activity of Avt3p is necessary for the efficient sporulation of S. pombe cells under nutrient starvation conditions. However, we should further examine the importance of 1–270 residues for sporulation using mutant strain constructed by genomic integration in future study.
Although we did not identify any remarkable motifs/domains in the N-terminal hydrophilic regions of Avt3/Avt4 homologs, we found some clusters of conserved residues among these transporters; there were four conserved sequence elements (CSEs), I-a, II, III, and IV, among the Avt3/Avt4 homologs from S. pombe (NP_593551), Aspergillus niger (EHA20494), A. oryzae (EIT78450), Penicillium marneffei (KFX46069), Ajellomyces capsulatus (EEH10659), Neurospora crassa (XP_961129), F. oxysporum Avt3p (FOXG_11334), and S. cerevisiae Avt3p (NP_012776) and Avt4p (NP_014298) (Fig. ). The analysis of export activities are currently in progress using isolated vacuolar membrane vesicles prepared from S. cerevisiae cells heterologously expressing CSE-deleted S. pombe Avt3p. The CSE-Ia is conserved among many fungal homologs but not in S. cerevisiae Avt3p and Avt4p. However, we found two other CSEs (I-b and I-c) (data not shown) near I-a in the sequences conserved among highly phylogenetically related Saccharomyces strains; the role of these CSEs in Saccharomyces Avt3p/Avt4p should be investigated to further understand the function of S. cerevisiae Avt3p/Avt4p. In addition, several residues in the N-terminal region of S. pombe Avt3p appear to be modified by phosphorylation (Fig. (A)).Citation30,31) These residues are not likely to reside in these CSEs, but their role in the regulation of Avt3p should be examined. In any case, the characteristics of Avt3p(∆1–270), in which nearly the entire N-terminal hydrophilic region of Avt3p was deleted, are valuable for understanding these CSEs and the function of Avt3p.
Fig. 4. Effect of deletion of the N-terminal region of Avt3p on spore formation of S. pombe.
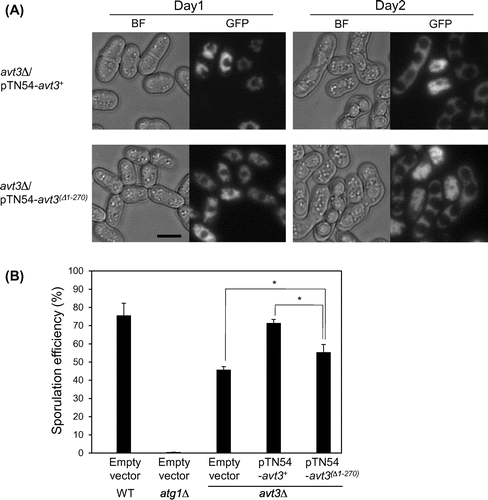
Fig. 5. Conserved sequence elements in the N-terminal hydrophilic regions of fungal Avt3/Avt4 homologs.
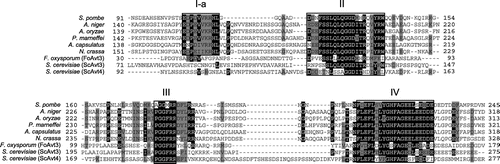
Author contributions
Study conception and design were done by Miyuki Kawano-Kawada, Soracom Chardwiriyapreecha, Takayuki Sekito, and Yoshimi Kakinuma.
Acquisition of data was done by Miyuki Kawano-Kawada, Soracom Chardwiriyapreecha, Kunio Manabe, and Takayuki Sekito.
Analysis and interpretation of data were done by Miyuki Kawano-Kawada, Soracom Chardwiriyapreecha, Kunio Manabe, Takayuki Sekito, and Koichi Akiyama.
Drafting of manuscript was done by Miyuki Kawano-Kawada and Takayuki Sekito.
Critical revision was done by Kaoru Takegawa and Yoshimi Kakinuma.
Disclosure statement
No potential conflict of interest was reported by the authors.
Funding
This work was supported in part by a Grant-in-Aid from the Japan Society for the Promotion of Science (JSPS) under the JSPS Postdoctoral Fellowship for Foreign Researchers (to S.C.), the Elizabeth Arnold Fuji Foundation (to M.K-K.), and the JSPS KAKENHI [grant number 15H04486] to YK, [grant number 15K07396] to TS.
Acknowledgments
We dedicate this work to the memory of the late Yoshimi Kakinuma, a mentor to so many of us.
Notes
Abbreviations: BF, bright field; CSE, conserved sequence element; GFP, green fluorescent protein.
References
- Li SC, Kane PM. The yeast lysosome-like vacuole: endpoint and crossroads. Biochim. Biophys. Acta. 2009;1793:650–663.10.1016/j.bbamcr.2008.08.003
- Kitamoto K, Yoshizawa K, Ohsumi Y, et al. Dynamic aspects of vacuolar and cytosolic amino acid pools of Saccharomyces cerevisiae. J. Bacteriol. 1988;170:2683–2686.
- Wiemken A, Durr M. Characterization of amino acid pools in the vacuolar compartment of Saccharomyces cerevisiae. Arch. Microbiol. 1974;101:45–57.10.1007/BF00455924
- Chardwiriyapreecha S, Hondo K, Inada H, et al. A simple and specific procedure to permeabilize the plasma membrane of Schizosaccharomyces pombe. Biosci. Biotechnol. Biochem. 2009;73:2090–2095.10.1271/bbb.90319
- Sato T, Ohsumi Y, Anraku Y. Substrate specificities of active transport systems for amino acids in vacuolar-membrane vesicles of Saccharomyces cerevisiae. Evidence of seven independent proton/amino acid antiport systems. J. Biol. Chem. 1984;259:11505–11508.
- Ohsumi Y, Anraku Y. Active transport of basic amino acids driven by a proton motive force in vacuolar membrane vesicles of Saccharomyces cerevisiae. J. Biol. Chem. 1981;256:2079–2082.
- Kakinuma Y, Ohsumi Y, Anraku Y. Properties of H+-translocating adenosine triphosphatase in vacuolar membranes of Saccharomyces cerevisiae. J. Biol. Chem. 1981;256:10859–10863.
- Sekito T, Fujiki Y, Ohsumi Y, et al. Novel families of vacuolar amino acid transporters. IUBMB Life. 2008;60:519–525.10.1002/iub.v60:8
- Shimazu M, Sekito T, Akiyama K, et al. A family of basic amino acid transporters of the vacuolar membrane from Saccharomyces cerevisiae. J. Biol. Chem. 2005;280:4851–4857.10.1074/jbc.M412617200
- Russnak R, Konczal D, McIntire SL. A family of yeast proteins mediating bidirectional vacuolar amino acid transport. J. Biol. Chem. 2001;276:23849–23857.10.1074/jbc.M008028200
- Tone J, Yoshimura A, Manabe K, et al. Characterization of Avt1p as a vacuolar proton/amino acid antiporter in Saccharomyces cerevisiae. Biosci. Biotechnol. Biochem. 2015;79:782–789.10.1080/09168451.2014.998621
- Tone J, Yamanaka A, Manabe K, et al. A vacuolar membrane protein Avt7p is involved in transport of amino acid and spore formation in Saccharomyces cerevisiae. Biosci. Biotechnol. Biochem. 2015;79:190–195.10.1080/09168451.2014.963501
- Sekito T, Chardwiriyapreecha S, Sugimoto N, et al. Vacuolar transporter Avt4 is involved in excretion of basic amino acids from the vacuoles of Saccharomyces cerevisiae. Biosci. Biotechnol. Biochem. 2014;78:969–975.10.1080/09168451.2014.910095
- Onodera J, Ohsumi Y. Autophagy is required for maintenance of amino acid levels and protein synthesis under nitrogen starvation. J. Biol. Chem. 2005;280:31582–31586.10.1074/jbc.M506736200
- Yang Z, Huang J, Geng J, et al. Atg22 recycles amino acids to link the degradative and recycling functions of autophagy. Mol. Biol. Cell. 2006;17:5094–5104.10.1091/mbc.E06-06-0479
- Mukaiyama H, Nakase M, Nakamura T, et al. Autophagy in the fission yeast Schizosaccharomyces pombe. FEBS Lett. 2010;584:1327–1334.10.1016/j.febslet.2009.12.037
- Mukaiyama H, Kajiwara S, Hosomi A, et al. Autophagy-deficient Schizosaccharomyces pombe mutants undergo partial sporulation during nitrogen starvation. Microbiology. 2009;155:3816–3826.10.1099/mic.0.034389-0
- Chardwiriyapreecha S, Manabe K, Iwaki T, et al. Functional expression and characterization of Schizosaccharomyces pombe Avt3p as a vacuolar amino acid exporter in Saccharomyces cerevisiae. PLoS One. 2015;10:e0130542.10.1371/journal.pone.0130542
- Lunprom S, Pongcharoen P, Sekito T, et al. Characterization of vacuolar amino acid transporter from Fusarium oxysporum in Saccharomyces cerevisiae. Biosci. Biotechnol. Biochem. 2015;79:1972–1979.10.1080/09168451.2015.1058703
- Fukura N, Ohgaki R, Matsushita M, et al. A membrane-proximal region in the C-terminal tail of NHE7 is required for its distribution in the trans-Golgi network, distinct from NHE6 localization at endosomes. J. Membr. Biol. 2010;234:149–158.10.1007/s00232-010-9242-9
- Feki K, Quintero FJ, Pardo JM, et al. Regulation of durum wheat Na+/H+ exchanger TdSOS1 by phosphorylation. Plant Mol. Biol. 2011;76:545–556.10.1007/s11103-011-9787-8
- Yamaguchi T, Aharon GS, Sottosanto JB, et al. Vacuolar Na+/H+ antiporter cation selectivity is regulated by calmodulin from within the vacuole in a Ca2+- and pH-dependent manner. Proc. Natl. Acad. Sci. U.S.A. 2005;102:16107–16112.10.1073/pnas.0504437102
- Mitsui K, Matsushita M, Kanazawa H. Saccharomyces cerevisiae glucose signalling regulator Mth1p regulates the organellar Na+/H+ exchanger Nhx1p. Biochem. J. 2010;432:343–352.10.1042/BJ20100796
- Moreno S, Klar A, Nurse P. Molecular genetic analysis of fission yeast Schizosaccharomyces pombe. Methods Enzymol. 1991;194:795–823.10.1016/0076-6879(91)94059-L
- Morita T, Takegawa K. A simple and efficient procedure for transformation of Schizosaccharomyces pombe. Yeast. 2004;21:613–617.10.1002/(ISSN)1097-0061
- Alfa C, Fantes P, Hyams J, et al. Experiments with fission yeast: a laboratory course manual. Cold Spring Harbor (NY): Cold Spring Harbor Laboratory Press; 1993.
- Amberg DC, Burke DJ, Strathern JN. Methods in yeast genetics: a cold spring harbor laboratory course manual, 2005th ed. Cold Spring Harbor (NY): Cold Spring Harbor Laboratory Press; 2005.
- Nakamura T, Nakamura-Kubo M, Hirata A, et al. The Schizosaccharomyces pombe spo3+ gene is required for assembly of the forespore membrane and genetically interacts with psy1+-encoding syntaxin-like protein. Mol. Biol. Cell. 2001;12:3955–3972.10.1091/mbc.12.12.3955
- Nakase M, Nakase Y, Chardwiriyapreecha S, et al. Intracellular trafficking and ubiquitination of the Schizosaccharomyces pombe amino acid permease Aat1p. Microbiology. 2012;158:659–673.10.1099/mic.0.053389-0
- Koch A, Krug K, Pengelley S, et al. Mitotic substrates of the kinase aurora with roles in chromatin regulation identified through quantitative phosphoproteomics of fission yeast. Sci. Signal. 2011;4:rs6.
- Carpy A, Krug K, Graf S, et al. Absolute proteome and phosphoproteome dynamics during the cell cycle of Schizosaccharomyces pombe (fission yeast). Mol. Cell Proteomics. 2014;13:1925–1936.10.1074/mcp.M113.035824