Abstract
Wheat noodles cooked for different periods of time were stored at 5 °C, and color changes in their cross sections were quantitatively assessed by digital image analysis. The color of noodles with flattened moisture distributions whitened greatly during the early stages of chilled storage due to the retrogradation of starch, with the color change showing a significant correlation with the changes in noodle fragility. Color changes were also measured for wheat noodles and noodles containing modified starch with internal moisture distributions, and local changes within the noodles were kinetically analyzed. The addition of modified starch significantly reduced the color change in the noodle interior, where the moisture content was relatively low. Scanning calorimetric measurements indicated differences in the gelatinized state of modified starch and original wheat starch at low moisture contents, which affected the rate of color change in the interior of noodles containing modified starch.
Graphical abstract
Changes in the average gray level of the cross-sectional image of wheat noodles cooked for 5 (), 13.8 (), and 20 min () during the storage at 5 °C.
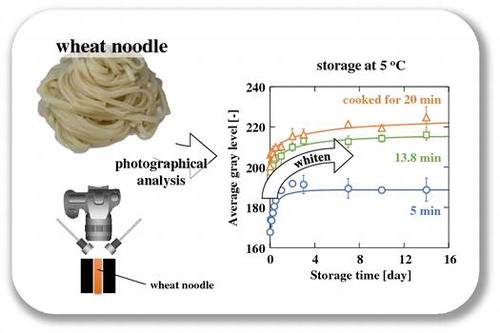
Wheat noodles, a common food prepared from wheat flour, are consumed worldwide. Noodle quality is affected by many factors, such as color, taste, and appearance, with texture being the most important factor.Citation1) Starch and gluten present in wheat flour affect the texture of the cooked noodle. Starch, which is a major component of wheat flour, swells during the water sorption process. The swelling properties of starchCitation2) and the state of starch granules after cookingCitation3) affect the cooked noodle texture. The content,Citation4) composition,Citation5) and thermal stabilityCitation6) of gluten also affect the texture. The addition of gluten to wheat flour increases the tensile strength of the cooked wheat noodle.Citation7) Furthermore, the effects of moisture distribution within the noodleCitation8,9) and the addition of chemically modified starch to wheat flourCitation10) on the noodle texture have been reported.
Chilled storage usually lowers the quality of cooked wheat noodles due to flattening of the moisture distribution in the noodleCitation11) and retrogradation of the gelatinized starch. Retrogradation consists of two steps, the gelation of amylose and the recrystallization of amylopectin, and diminishes the texture and digestibility of foods containing starch.Citation12,13) Huang and Lai reported that starch retrogradation is the main cause of changes in wheat noodle texture.Citation14) In this context, understanding retrogradation behavior within the wheat noodle is important for controlling noodle quality during storage.
Starch retrogradation is usually assessed by X-ray diffraction,Citation15) differential scanning calorimetry (DSC),Citation16) as well as enzymaticCitation17) and spectroscopic methods.Citation18,19) However, these methods are not suitable for the quantitative monitoring of amylose gelation, which occurs during the early stages of starch retrogradation. Amylose leaked from starch granules during gelatinization forms double-helical aggregates during chilled storage, resulting in a whitening of the starch paste.Citation20,21) Many studies on retrogradation-related changes in the turbidity of starch paste have been carried out.Citation22,23) We kinetically analyzed the retrogradation process of concentrated wheat starch solutions based on color changes.Citation24)
In this study, retrogradation-induced color changes in the wheat noodle cross section were quantitatively analyzed using digital image analysis.Citation25) The relationship between changes in color and texture was also examined. The color change in wheat noodles containing modified starch was also kinetically analyzed, and the effects of modified starch addition on the retrogradation behavior are discussed.
Materials and methods
Materials
Wheat flour suitable for the preparation of Udon or Japanese white-salted noodles and hydroxypropylated tapioca starch (degree of substitution = 0.03; referred to as modified starch) was supplied by Nisshin Seifun Group, Inc. (Tokyo, Japan). The protein and ash contents of the flour were 8.6 and 0.35% (w/w) on a wet basis, respectively.
Preparation of wheat noodles
Wheat flour (500 g) was gradually mixed with tap water (150 g) using a blender (KSM 150, KitchenAid, USA) for 20 min to produce dough. Wheat noodles were prepared by passing the dough through a pasta-making machine (Magica, Bottene, Italy) equipped with a Teflon-coated die (2.25 mmϕ) under reduced pressure (41.3–51.3 kPa abs.). The noodle with no modified starch added is referred to as the original wheat noodle, or noodle S0.
Modified starch was mixed with the wheat flour to produce starch contents of 10, 20, or 30% (w/w). Wheat flour containing 10, 20, or 30% modified starch (each 500 g) was mixed with 160, 170, and 175 g tap water, respectively, to produce dough and noodles using the method described above. Noodles containing 10, 20, and 30% modified starch are referred to as noodles S10, S20, and S30, respectively.
Water sorption
Distilled water (45 mL) in a 50-mL culture tube (VIO-50R, As One, Osaka, Japan) was heated to 98 °C or higher by immersing in boiling water. An 8-cm noodle was immersed into the distilled water for a specific period. After that period, the noodle was removed and excess water on the noodle surface was blotted away with a paper towel (Nippon Paper Crecia, Tokyo). The amount of water sorption was estimated from the difference in weight before and after drying at 135 °C for 5 h in a DN-400 oven (Yamato Scientific, Tokyo).
Storage
The noodle, having undergone water sorption for a specific period, was wrapped with a film and an aluminum laminate film (AL-E, Seisannipponsha, Tokyo), and stored at 80 °C for 3 days in order to flatten the moisture distribution within the noodle. This sample is called flattened noodle S0. Samples containing the modified starch were stored at 5 °C immediately after water sorption, and labeled as unflattened noodles.
Color change during storage
After storage at 5 °C for a specific period, the noodle was cut along the width with a stainless steel blade (B43-1SW, Sogo Laboratory Glass Works, Kyoto) and fixed to a sample holder with a hole of the same diameter as the sample. A cross-sectional image of the sample was taken using an EOS 40D digital camera (Canon, Tokyo) equipped with a macro lens (MP-E 65 mm, Canon) with illumination from above by a fiber-type light source (PICL-NSX, NPI, Tokyo). The emissivity of both the holder and the inside surface of the measurement box was 0.94. The cross-sectional image was divided into 10 parts using free image-editing software (GIMP2). Each image was resolved to red (R), green (G), and blue (B) images from the original 24-bit RGB image, and the 8-bit blue image was used to obtain brightness distribution. The 8-bit images were expressed using 256 gray levels (0–255). The brightness distribution was obtained by plotting the average gray levels for pixels in the 10 parts against their distance from the noodle center. The average gray level over the whole cross-section, Gt, was calculated using Equation (Equation1(1) ).
(1)
where n is the pixel number from the center and Gn is the gray level at the nth pixel. Measurements were carried out in triplicate for each sample.
Break test
A break test was performed for flattened noodle S0 stored at 5 °C using a creep meter (RE2-33005S, Yamaden, Tokyo) equipped with a wedge-shaped plunger (No. 49, Yamaden) to obtain a load–strain curve. The moving velocity of the plunger was 1 mm/s, and the maximum strain was set at 99%. Breaking and fragility loads were estimated from the load–strain curve. Measurements were carried out in triplicate for each sample.
Degree of gelatinization
Wheat flour and modified starch (1.5–2.5 mg) had moisture contents of 0.155 and 0.152 kg-H2O/kg-dm, respectively. Wheat flour or modified starch was weighed precisely using an electric balance (BM20, A & D, Tokyo) and put into an aluminum cell (SSC000C009, Hitachi High-Technologies, Tokyo). The adequate amount of water was added to the cell to reach 4.0 kg-H2O/kg-dm. The cell was tightly sealed using a sample sealer (Hitachi High-Technologies), and kept at 5 °C for 30 min or more. DSC measurements were performed using a DSC7020 calorimeter (Hitachi High-Technologies) in the temperature range 5–95 °C, increasing at a rate of 5 °C/min. Measurements were carried out in triplicate for each sample in order to confirm the reproducibility.
Results and discussion
Color change in wheat noodles with flattened moisture distributions
Wheat noodles S0 were cooked for 5, 13.8, and 20 min, then moisture distributions within the noodles were flattened as described above. The resulting moisture contents were 1.35, 2.10, and 2.53 kg-H2O/kg-dm, respectively. The noodles were stored at 5 °C and changes in the average gray levels of their cross sections were monitored (Fig. ). The average gray level increased greatly during the early stage (the first three days) of storage, reaching an equilibrium level with prolonged storage. The increase in gray level indicated that the color tone of the cross section had whitened during storage. As the moisture distribution had been flattened, no water migration occurred during storage at 5 °C. Therefore, the change in moisture distribution had not caused the color change. Gelatinized starch retrogrades during storage at low temperature. The retrogradation of starch proceeds through both the gelation of amylose and recrystallization of amylopectin. The former occurs within several hours at low temperature,Citation26) and the turbidity of gelatinized starch increases greatly along with gelation.Citation21) Therefore, the increase in gray level reflects the change in color tone caused by the gelation of amylose during the early stages of storage.
Fig. 1. Changes in the average gray level, Gt, for wheat noodles cooked for 5 (○), 13.8 (□), and 20 min (△) during storage at 5 °C.
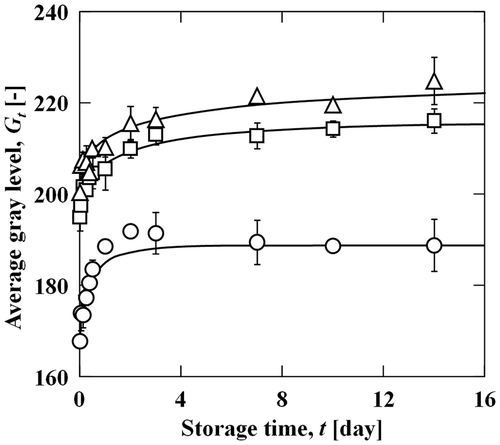
The transient change in the gray level, which was normalized to the change after 14 days, was expressed using the Weibull equation (Equation (Equation2(2) )).Citation27)
(2)
where G0 and G14 are the average gray levels immediately after storage and on day 14, respectively, k is the rate constant, and n is the shape constant. Equation (Equation2(2) ) can be transformed as follows (Equation (Equation3
(3) )):
(3)
Fig. shows plots of the left-hand side of Equation (Equation3(3) ) against log t values for noodles cooked for 5, 13.8, and 20 min. The k and n values for these noodles were estimated as 2.70 × 10−5 s−1 and 0.642, 0.755 × 10−5 s−1 and 0.529, and 0.425 × 10−5 s−1 and 0.451, respectively. Solid curves in Fig. were constructed using the estimated k and n values, and represent the experimental points well (R2 = 0.935–0.979).
Fig. 2. vs. log t for wheat noodles cooked for 5 (○), 13.8 (□), and 20 min (△) during storage at 5 °C.
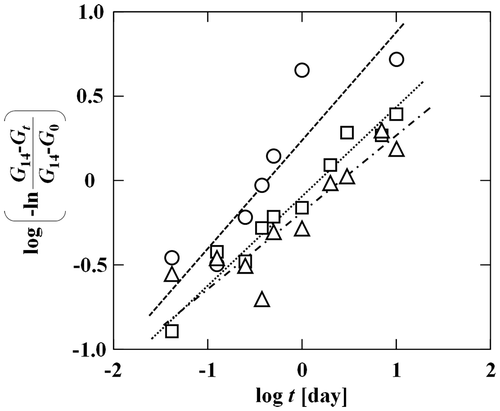
The k values for noodles cooked for longer durations, i.e. noodles with higher moisture contents, were smaller. This fact indicated that an increase in the noodle moisture content slowed the retrogradation of starch during the early stages of storage, which was caused mainly by the gelation of amylose. The retrogradation of starch is affected by the crystal structure of starch granules remaining after gelatinizationCitation28) and the degree of starch gelatinization at the beginning of storage.Citation29) Because amylose chains, which contribute to the whitening of the wheat noodle, leak from the starch granules with disintegration of the granules during gelatinization, the state of amylose depends on the average moisture content. Amylose remains within the granules at low moisture contents, because starch keeps the granule structure. On the other hand, amylose leaks from the granule at high moisture contents as the granule structure disintegrates. Amylose would be prone to gelatinizing during starch retrogradation through the aggregation of amylose molecules, due to their restricted mobility within starch granules. Therefore, higher k values for noodles with lower moisture contents would be ascribed to remaining amylose within the granules. On the other hand, the n value, which reflects the mechanism for whitening, was about 0.5 for all noodles, irrespective of moisture content. This result indicated that whitening proceeds via almost the same mechanism by the gelation of amylose in any moisture content.
In this context, the change in wheat noodle color tone during chilled storage would be ascribed to gel development, which was controlled by the diffusion rate of amylose chains released in the gelatinization step, and retrogradation of wheat noodle would proceed via the common mechanism, independently of moisture content.
Textural change in wheat noodles
Fig. shows the transient changes in breaking and fragility loads for moisture distribution flattened S0 wheat noodles. The breaking load scarcely changed during the first two days of storage, and slightly increased during days 3 to 7 of storage. In the starch retrogradation process, recrystallization of amylopectin takes a few days.Citation26) Therefore, the slight change in breaking load from days 3 to 7 is due to the recrystallization of amylopectin. Both the breaking and fragility loads decreased with increasing moisture content, although these changes were not proportional to the change in moisture content. In general, the gluten structure predominantly affects the mechanical properties of wheat noodles. However, starch granules embedded within the gluten network also affect the properties for noodles with identical gluten network structures. Thus, starch granules would disintegrate more at higher noodle moisture contents due to starch gelatinization, resulting in the low breaking and fragility loads. The change in fragility load was similar to the change in the average gray level (Fig. ). This tendency coincided with our previous observations on the change in turbidity of starch gel.Citation24) Fig. shows the relationship between relative gray level (Gt/G0) and relative fragility load (Wt/W0), where subscripts 0 and t indicate the initial and any selected storage times, respectively. At each moisture content, there was a strong positive correlation (R2 = 0.795–0.974) between these two quantities during the early stages of storage. The relative fragility load increased greatly with small changes in the relative gray level during prolonged storage. Recrystallization, which occurred at the late stages of storage, brought about textural changes in each wheat noodle. These observations indicated that the increase in the average gray level of the wheat noodle cross section reflected textural changes in the wheat noodle during the early stages of storage, and that retrogradation could be quantitatively estimated from color changes in the cross-sectional image. Image analysis techniques could be applicable to the loss in quality induced by starch retrogradation in wheat flour-based foods.
Local change in gray level within wheat noodles
Fig. shows the transient changes in average gray level for noodles prepared using wheat flour containing modified starch and cooked to produce the overall moisture contents of ca. 2.0 kg-H2O/kg-dm. Moisture distributions within the noodles were not flattened. Noodles with different modified starch contents had greatly increased the average gray levels over the distributions during the first three days of storage. The large increase in average gray levels for unflattened noodles was similar to that observed for flattened noodles (Fig. ). Water migration occurred in the original noodle due to the moisture gradient. Therefore, the increase in average gray level reflected both starch retrogradation and the change in moisture distribution. The transient change in gray level was expressed by Equation (Equation2(2) ) (R2 = 0.903–0.973) as used for the flattened noodle. The k and n values were estimated using plots of Equation (Equation3
(3) ). Fig. shows the dependence of k and n values on the modified starch content of wheat noodles. The n values (ca. 0.2–0.5) were independent of modified starch content. This result indicated that the mechanism for whitening in the cross-sectional image of the noodle containing modified starch was almost the same as within original noodle S0. On the other hand, the addition of modified starch decreased the k values. These results indicated that the addition of modified starch to the noodle increased the noodle transparency, making it difficult to detect the change in color tone, and retarded starch retrogradation within the noodles, in agreement with the results of previous studies.Citation30,31)
Fig. 5. Changes in the average gray level, Gt, for wheat noodles containing 0 (●), 10 (□), 20 (△), and 30% (w/w) (○) chemically modified starch during storage at 5 °C.
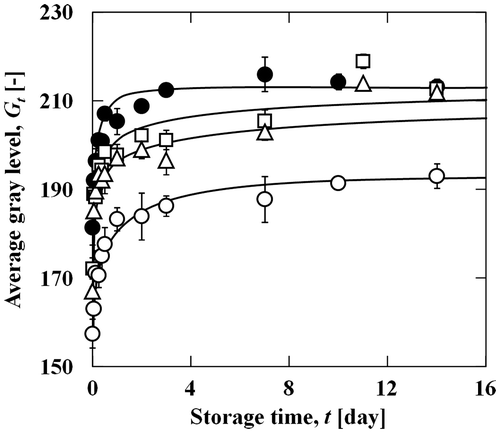
Fig. 6. Dependence of k (○) and n (●) values on the chemically modified starch content of wheat noodles.
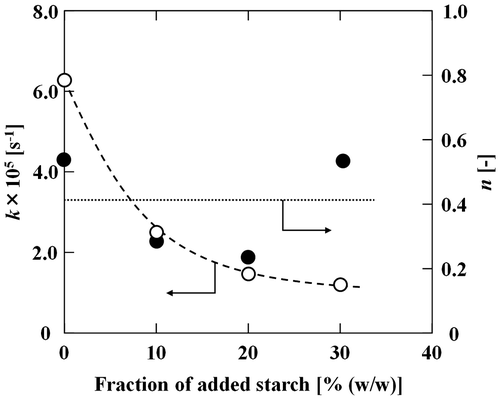
Next, the local change in gray level of wheat noodles containing a moisture gradient, labeled as unflattened noodles, was measured. The cross-sectional images of wheat noodles were divided into three regions (center, middle, and surface) and the average gray levels were measured for each region. The changes in average gray level during storage are shown in Fig. . The average gray level of each region increased during the first three days of storage, reaching an equilibrium level during prolonged storage, and was independent of modified starch content. For any region, the transient change could be expressed with Equation (Equation2(2) ) (R2 = 0.848–0.991), and the k and n values for each region were estimated by plotting Equation (Equation3
(3) ). Fig. shows the k and n values for S0, S10, S20, and S30 vs. moisture contents for each region, which were estimated from the moisture profiles within the noodles, as described in our previous study.Citation32) For all samples, S0, S10, S20, and S30, the differences in n values among the regions were small. This suggested that whitening in the noodle cross-sectional image proceeded via almost the same mechanism in any region, regardless of moisture content. On the other hand, the k value decreased with increasing moisture content. This decrease was also observed for the flattened noodle, as shown in the previous section. The state of starch granules in the center region was different from those in the surface region, and starch granules in the center region retained their shape.Citation3) Therefore, the high k values in the center region with low moisture could be ascribed to the gelation of amylose, which remained in the starch granules due to no granule disintegration occurring. This value was also affected by the large change in moisture content at the center region, which was induced by water migration from the surface region with high moisture content, to the center region with low moisture content. Although moisture contents in the center, middle, and surface regions of unflattened noodle S0 were almost the same as in flattened noodles cooked for 5, 13.8, and 20 min, respectively, their k values were much larger. The partially remaining starch granule structure in the unflattened noodle, with a lower degree of gelatinization, resulted in a larger k value, as well as water migration due to the moisture gradient in the unflattened noodle. Thus, the difference in k values between the flattened and unflattened noodles was ascribed to different starch granule states, while the addition of modified starch affected both starch retrogradation and changes in the moisture content of the center and middle regions, which had relatively low moisture contents.
Fig. 7. Changes in the average gray level, Gt, at the center (○), middle (□), and surface (△) regions of wheat noodles containing (a) 0, (b) 10, (c) 20, and (d) 30% (w/w) chemically modified starch during storage at 5 °C. Note: Bars indicate standard deviation for three independent measurements.
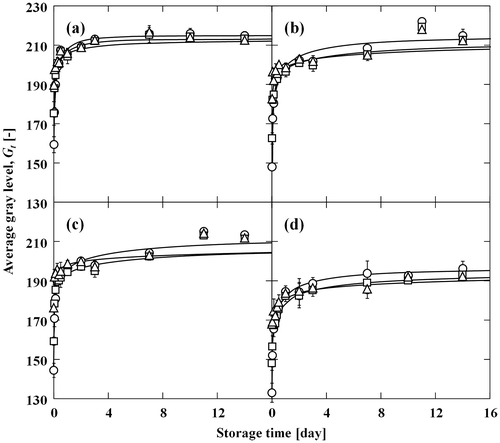
Fig. 8. The dependence of (a) k and (b) n values on the average moisture content in wheat noodles containing 0 (●), 10 (□), 20 (△), and 30% (w/w) (○) chemically modified starch.
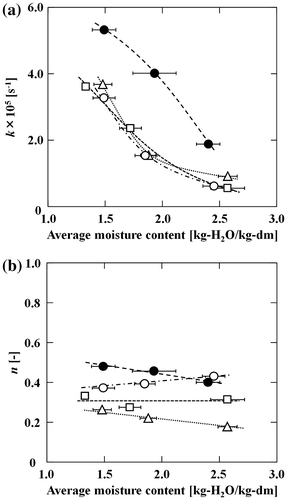
Moreover, adding modified starch influenced the k value. The k values of all regions, especially the center and middle regions, in S10, S20, and S30, were much lower than those of S0. This indicated that the addition of modified starch affected starch retrogradation and changed the moisture content, particularly in the center and middle regions, where the moisture contents were relatively low. Starch retrogradation during the early stages of storage was mainly ascribed to the gelation of amylose leached out of the starch granules during gelatinization. The amylose content of tapioca starch, which was the modified starch ingredient used in this study, is generally lower than that of wheat starch.Citation33) Thus, the lower amylose contents of S10, S20, and S30 relative to S0 contributed to the drop in starch retrogradation. However, other factors also affected the change in the rate constant, because changes in the k value depended on moisture content. The physicochemical properties of chemically modified starches are different from those of native starches. The swelling properties and solubilities of hydroxypropylated starches are higher than those of native starches,Citation34) while the gelatinization temperatures and enthalpies of gelatinization are lower.Citation35) These properties suggest that the water sorption and gelatinization behavior of modified starch used in this study were different to those of native starch in wheat flour, and contributed to changes in the state of starch granules within the noodle, starch retrogradation rate, and moisture distribution.
DSC analysis
The gelatinization properties of the modified and native starches in wheat flour were compared. The enthalpy of gelatinization, ΔH, was normalized as shown on the left-hand side of Equation (Equation4(4) ).
(4)
where ΔH0 and ΔHe are the enthalpies of gelatinization for starches with 0 and 4.0 kg-H2O/kg-dm of added water, respectively. Fig. shows normalized values against the added amount of water, X. Values of (ΔHX – ΔH0)/(ΔHe – ΔH0) for the modified starch and native starch greatly increased between 1.0 and 2.0 kg-H2O/kg-dm, and when the amount of added water was above 2.5 kg-H2O/kg-dm, the values were almost 1.0. This result indicated that the degree of starch gelatinization increased between 1.0 and 2.0 kg-H2O/kg-dm, and that the amount of water sufficient for starch gelatinization measured by DSC was 2.5 kg-H2O/kg-dm or more. The change in (ΔHX – ΔH0)/(ΔHe – ΔH0) was expressed using Equation (Equation4(4) ) (R2 = 0.985 and 0.992), where X′ is amount of water added when (ΔHX – ΔH0)/(ΔHe – ΔH0) = 0.5, and kh is constant, respectively. The X′ values of modified starch and native starch were 1.34 and 1.48 kg-H2O/kg-dm, with kh values of 3.11 and 4.55 kg-H2O/kg-dm, respectively. These results suggested that the degree of gelatinization of modified starch was higher than that of native starch at moisture contents insufficient for gelatinization. As the average moisture content in the center and middle regions of the wheat noodle were ca. 1.5–2.0 kg-H2O/kg-dm, the state of the added modified starch would be different from that of the native starch. Thus, the differences in starch state between the modified and native starches would be remarkable in the regions. The state of modified starch would be a factor affecting the favorable decrease in the rate constant for the average gray level.
Conclusion
The color changes of cross sections in wheat noodles cooked for different periods were quantitatively monitored by digital image analysis to examine starch retrogradation kinetics inside the noodles during chilled storage at 5 °C. Noodles with flattened moisture distributions whitened greatly during the early stages of chilled storage, and the color change was closely correlated with the change in noodle fragility. These changes were ascribed to starch retrogradation by the gelation of amylose. Color changes were also measured for wheat noodles and noodles containing modified starch with internal moisture distributions, and local color changes within the noodles were kinetically analyzed using the Weibull equation. The addition of modified starch significantly decreased the color change in the noodle interior due to different gelatinization states in the modified and original wheat starches at low moisture contents. This difference was partly responsible for the relatively small rate constant for retrogradation.
Author contributions
All authors conceived of and designed the experiments, and discussed the results. S.F. performed the experiments, analyzed the data along with T.O., and wrote the manuscript in consultation with T.O. and S.A.
Fundings
This work was partially supported by the Grant-in-Aid for Challenging Exploratory Research by Japan Society for the Promotion of Science [S.A.; grant number 26560045]; the Grant-in-Aid for the Japan Society for the Promotion of Science Fellows [T.O.; grant number 14J02443].
Disclosure statement
No potential conflict of interest was reported by the authors.
Acknowledgment
This study was carried out as part of a project conducted by the Cereal Science Consortium by the Graduate School of Agriculture, Kyoto University, Gifu University, and the Nissin Seifun Group, Inc.
References
- Toyokawa H, Rubenthaler GL, Powers JR, et al. Japanese noodle qualities. I. Flour components. Cereal Chem. 1989;66:382–386.
- Crosbie GB. The relationship between starch swelling properties, paste viscosity and boiled noodle quality in wheat flour. J. Cereal Sci. 1991;13:145–150.10.1016/S0733-5210(09)80031-3
- Lai HM, Hwang SC. Water status of cooked white salted noodles evaluated by MRI. Food Res. Int. 2004;37:957–966.10.1016/j.foodres.2004.06.008
- Del Nobile MA, Baiano A, Conte A, et al. Influence of protein content on spaghetti cooking quality. J. Cereal Sci. 2005;41:347–356.10.1016/j.jcs.2004.12.003
- Kovacs MIP, Howes NK, Leisle D, et al. Effect of two differential low molecular weight glutenin subunits on durum wheat pasta quality parameters. Cereal Chem. 1995;72:85–87.
- Kovacs MIP, Fu BX, Woods SM, et al. Thermal stability of wheat gluten protein: its effect on dough properties and noodle texture. J. Cereal Sci. 2004;39:9–19.10.1016/S0733-5210(03)00058-4
- Park SJ, Baik BK. Quantitative and qualitative role of added gluten on white salted noodles. Cereal Chem. 2009;86:646–652.10.1094/CCHEM-86-6-0646
- Irie K, Horigane AK, Naito S, et al. Moisture distribution and texture of various types of cooked spaghetti. Cereal Chem. 2004;81:350–355.10.1094/CCHEM.2004.81.3.350
- Ogawa T, Adachi S. Moisture distribution and texture of spaghetti rehydrated under different conditions. Biosci. Biotechnol. Biochem. 2016;80:769–773.10.1080/09168451.2015.1116930
- Eguchi S, Kitamoto N, Nishinari K, et al. Effects on esterified tapioca starch on the physical and thermal properties of Japanese white salted noodles prepared partly by residual heat. Food Hydrocoll. 2014;35:198–208.10.1016/j.foodhyd.2013.05.012
- Kojima TI, Horigane AK, Yoshida M, et al. Change in the status of water in Japanese noodles during and after boiling observed by NMR micro imaging. J. Food Sci. 2001;66:1361–1365.10.1111/jfds.2001.66.issue-9
- Ring SG, Gee JM, Whittam M, et al. Resistant starch: its chemical form in foodstuffs and effect on digestibility in vitro. Food Chem. 1988;28:97–109.10.1016/0308-8146(88)90139-2
- Yu S, Ma Y, Sun DW. Impact of amylose content on starch retrogradation and texture of cooked milled rice during storage. J. Cereal Sci. 2009;50:139–144.10.1016/j.jcs.2009.04.003
- Huang YC, Lai HM. Noodle quality affected by different cereal starches. J. Food Eng. 2010;97:135–143.10.1016/j.jfoodeng.2009.10.002
- Blazek J, Gilbert EP. Application of small-angle X-ray and neutron scattering techniques to the characterisation of starch structure: a review. Carbohydr. Polym. 2011;85:281–293.10.1016/j.carbpol.2011.02.041
- Stevens DJ, Elton GAH. Thermal properties of the starch/water part 1. Measurement of heat of gelatinisation by differential scanning calorimetry. Stach-Stӓrke. 1971;23:8–11.
- Kainuma K, Matsunaga A, Itagawa M, et al. New enzyme system-beta-amylase-pullulanase to determine the degree of gelatinization and retrogradation of starch or starch products. J. Jpn. Soc. Starch Sci. 1981;28:235–240.10.5458/jag1972.28.235
- Smits ALM, Ruhnau FC, Vliegenthart JFG. Ageing of starch based systems as observed with FT-IR and solid state NMR spectroscopy. Stach-Stӓrke. 1998;50:478–483.
- Fechner PM, Wartewig S, Kleinebudde P, et al. Studies of the retrogradation process for various starch gels using Raman spectroscopy. Carbohydr. Res. 2005;340:2563–2568.10.1016/j.carres.2005.08.018
- Gidley MJ. Molecular mechanisms underlying amylose aggregation and gelation. Macromolecules. 1989;22:351–358.10.1021/ma00191a064
- Ambigaipalan P, Hoover R, Donner E, et al. Retrogradation characteristics of pulse starches. Food Res. Int. 2013;54:203–212.10.1016/j.foodres.2013.06.012
- Miles MJ, Morris VJ, Ring SG. Gelation of amylose. Carbohydr. Res. 1985;135:257–269.10.1016/S0008-6215(00)90777-8
- Jacobson MR, Obanni M, Bemiller JN. Retrogradation of starches from different botanical sources. Cereal Chem. 1997;74:511–518.10.1094/CCHEM.1997.74.5.511
- Fukuzawa S, Ogawa T, Nakagawa K, et al. Kinetics on the turbidity change of wheat starch during its retrogradation. Biosci. Biotechnol. Biochem. 2016;80:1609–1614.
- Ogawa T, Adachi S. Measurement of moisture profiles in pasta during rehydration based on image processing. Food Bioprocess Technol. 2014;7:1465–1471.10.1007/s11947-013-1156-y
- Wang S, Copeland L. Molecular disassembly of starch granules during gelatinization and its effect on starch digestibility: a review. Food Funct. 2013;4:1564–1580.10.1039/c3fo60258c
- Cunha LM, Oliveira FAR, Oliveira JC. Optimal experimental design for estimation the kinetic parameters of processes described by the Weibull probability distribution function. J. Food Eng. 1998;37:175–191.10.1016/S0260-8774(98)00085-5
- Fisher DK, Thompson DB. Retrogradation of maize starch after thermal treatment within and above the gelatinization temperature range. Cereal Chem. 1997;74:344–351.10.1094/CCHEM.1997.74.3.344
- Fu ZQ, Wang LJ, Li D, et al. The effect of partial gelatinization of corn starch on its retrogradation. Carbohydr. Polym. 2013;97:512–517.10.1016/j.carbpol.2013.04.089
- Perera C, Hoover R. Influence of hydroxypropylation on retrogradation properties of native, defatted and heat-moisture treated potato starches. Food Chem. 1999;64:361–375.10.1016/S0308-8146(98)00130-7
- Miyazaki M, Maeda T, Morita N. Starch retrogradation and firming of bread containing hydroxypropylated, acetylated, and phosphorylated cross-linked tapioca starches for wheat flour. Cereal Chem. 2005;82:639–644.10.1094/CC-82-0639
- Fukuzawa S, Ogawa T, Nakagawa K, et al. Moisture profiles of wheat noodles containing hydroxypropylated tapioca starch. Int. J. Food Sci. Technol. 2016;51:1516–1522.10.1111/ijfs.2016.51.issue-6
- Jane J, Chen YY, Lee LF, et al. Effects of amylopectin branch chain length and amylose content on the gelatinization and pasting properties of starch. Cereal Chem. 1999;76:629–637.10.1094/CCHEM.1999.76.5.629
- Singh J, Kaur L, McCarthy OJ. Factors influencing the physico-chemical, morphological, thermal and rheological properties of some chemically modified starch for food applications—a review. Food Hydrocoll. 2007;21:1–22.10.1016/j.foodhyd.2006.02.006
- Perera C, Hoover R, Martin AM. The effect of hydroxypropylation on the structure and physicochemical properties of native, defatted and heat-moisture treated potato starch. Food Res. Int. 1997;30:235–247.10.1016/S0963-9969(97)00041-0