Abstract
The application of marine resources, instead of fossil fuels, for biomass production is important for building a sustainable society. Seaweed is valuable as a source of marine biomass for producing biofuels such as ethanol, and can be used in various fields. Alginate is an anionic polysaccharide that forms the main component of brown algae. Various alginate lyases (e.g. exo- and endo-types and oligoalginate lyase) are generally used to degrade alginate. We herein describe a novel alginate lyase, AlgC-PL7, which belongs to the polysaccharide lyase 7 family. AlgC-PL7 was isolated from the halophilic Gram-negative bacterium Cobetia sp. NAP1 collected from the brown algae Padina arborescens Holmes. The optimal temperature and pH for AlgC-PL7 activity were 45 °C and 8, respectively. Additionally, AlgC-PL7 was thermostable and salt-tolerant, exhibited broad substrate specificity, and degraded alginate into monosaccharides. Therefore, AlgC-PL7 is a promising enzyme for the production of biofuels.
Graphical Abstract
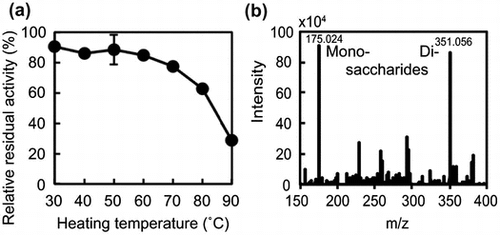
The development of alternatives to fossil fuels is important for building a sustainable society. The use of algal biomass as an alternative energy source has attracted considerable attention.Citation1–3) Alginate is a linear anionic polysaccharide that forms the major component of the cell wall of brown algae and an exopolysaccharide synthesized by various bacterial species, including Pseudomonas aeruginosa.Citation4–6) The constituent sugar residues [i.e. α-l-guluronic acid (G) and β-d-mannuronic acid (M)] in alginate are linked by 1,4-O-glycosidic bonds. Therefore, alginate consists of the following three distinct regions: a polyguluronic acid-rich region (polyG), a polymannuronic acid-rich region (polyM), and a random region (polyMG).Citation4) For biofuel production, the alginate polysaccharides must be degraded to monosaccharides, which are then converted to 4-deoxy-L-erythro-5-hexoseulose uronic acid. Biofuel is ultimately produced through the activities of metabolically engineered recombinant micro-organisms.Citation7,8) Moreover, the oligosaccharides that are partially degraded from alginate have various specific physiological functions and biological activities, including anti-oxidant, anti-hypersensitive, and anti-proliferative activities, anti-allergic properties, and plant root growth-promoting functions.Citation9–15) Thus, alginate and its derivatives are useful in the biotechnology, food, and pharmaceutical industries.
Alginate lyases, which are polysaccharide lyases (PLs), catalyze the depolymerization of alginate through β-elimination of the 4-O-glycosidic bonds accompanied by the formation of a double bond between C-4 and C-5.Citation16) The PLs are classified into 24 families in the Carbohydrate-Active enZYmes Database (http://www.cazy.org), with alginate lyases belonging to the PL-5, -6, -7, -14, -15, -17, and -18 families.Citation17) Various alginate lyases have been isolated from marine algae, marine mollusks, fungi, bacteria, bacteriophages, and viruses.Citation16) Alginate lyases are generally divided into two groups based on substrate specificity. The first group includes the polyG lyases (EC 4.2.2.11), which are specific to the polyG and polyMG regions, while the second group is composed of polyM lyases (EC 4.2.2.3), which are specific to the polyM region. Furthermore, the alginate lyases of both groups act as exolytic or endolytic enzymes. However, some alginate lyases are active against both polyG and polyM, implying that these enzymes degrade alginate more effectively.Citation18–20) To date, over 50 alginate lyases have been isolated from various sources and characterized.Citation16,21)
During alginate degradation by marine bacteria, various alginate lyases are involved in extra- and intercellular degradation pathways. Alginate lyases belonging to the PL-5 and -7 families degrade alginate endolytically into oligosaccharides. Oligoalginate lyases from the PL-15 and -17 families then degrade oligosaccharides exolytically into monosaccharides.Citation22) Therefore, at least two alginate lyases degrade alginate during the production of biofuels in an engineered yeast system.Citation8,23) Identifying novel thermostable and salt-tolerant alginate lyases with a broader substrate specificity and the ability to efficiently degrade alginate for bioengineering applications is important.
In this study, we focused on thermostable alginate lyases derived from marine bacteria and isolated a novel alginate lyase (AlgC-PL7) from the halophilic Gram-negative bacterium Cobetia sp. NAP1. Although AlgC-PL7 was only slightly more active than the other PL-7 family members, it exhibited thermostability, broad substrate specificity, and the ability to degrade alginate into monosaccharides.
Materials and methods
Screening of marine bacterial enzymes capable of degrading alginate
To identify marine bacteria that produce alginate-degrading enzymes, we isolated various species of green, red, and brown marine algae from the coastal waters of Tottori, Japan. A 5-g sample of each marine algae was added to culture medium [23 mM K2HPO4, 3.7 mM KH2PO4, 1.7 mM MgSO4, 15 mM (NH4)2SO4, 0.5 M NaCl (3%, w/v)] supplemented with a metal solution and vitamin mixture, with 0.5% (w/v) sodium alginate (300 cps) (Nacalai Tesque Inc., Kyoto, Japan) as the sole carbon source (alginate medium). The marine bacterial cultures were incubated at 30 °C. Pure strains were obtained using a repeated enrichment culture procedure and an agar plate dilution method. Marine bacteria were identified based on a phylogenetic analysis of their 16S rRNA sequence.
Purification of enzymes from marine bacteria
To purify enzymes from Cobetia sp. NAP1, we optimized the growth conditions (i.e. temperature and shaking speed) for a large-scale culture. The bacterial cells were grown in alginate medium at 20 °C with shaking (180 rpm) for 24 h. Cells were harvested and sonicated in 50 mM Tris–HCl buffer (pH 7.0). Samples were centrifuged and the supernatants containing AlgC-PL7 were heated at 90 °C for 5 min (heat treatment) and centrifuged again. After the heat treatment, 5% streptomycin sulfate (2.5% final concentration) was added to the supernatants, which were then centrifuged to remove nucleic acids. Supernatants were dialyzed in 50 mM Tris–HCl (pH 7.0) and loaded onto a Resource-Q column (GE Healthcare, Little Chalfont, UK). Proteins were eluted using the AKTA protein purification system (GE Healthcare) with a linear gradient of 0–1.0 M NaCl. Protein fractions were collected and loaded onto a Superdex™ 75 column (GE Healthcare). The fractions containing AlgC-PL7 were dialyzed and then reloaded on the Resource-Q column. The AlgC-PL7 concentration was determined using a commercial Bradford protein assay kit (Bio-Rad Laboratories, Hercules, CA, USA) with bovine serum albumin as the standard protein.
N-terminal amino acid sequencing
To determine the N-terminal amino acid sequence of the purified AlgC-PL7, proteins were separated by 12.5% sodium dodecyl sulfate-polyacrylamide gel electrophoresis (SDS-PAGE) and then transferred to a polyvinylidene difluoride membrane. The amino acid sequence was determined using the PPSQ-30 protein sequencer (Shimadzu Corporation, Kyoto, Japan).
Enzyme activity assay
Enzyme activities were determined based on a lyase activity assay and size exclusion chromatography (SEC) (i.e. analysis of degradation products). Briefly, a 1-mL reaction solution consisting of 0.1 M Tris–HCl buffer (pH 7.0), 0.2% (v/v) substrate containing sodium alginate (300 cps), polyMG, polyM, and polyG, 5–10 μL AlgC-PL7 (0.02–0.04 U), 10 μL recombinant AlgC-PL7 (0.03U), and 10 μL cell free extract (CFE) from Escherichia coli BL21 (DE3) cells was used to determine lyase activity. PolyMG, polyM, and polyG were prepared according to the method described by Haug et al.Citation24) Samples were analyzed at 40 °C for 3–5 min, and the absorbance (232 nm wavelength; A232) was recorded. To determine the optimal temperature (20–55 °C), pH (4–9.5), salt (0.1–2.0 M NaCl and KCl), and metal (1 mM) concentrations, and substrate specificity (polyMG, polyM, and polyG) for the enzyme, samples were incubated at 4 °C before measurements. To analyze thermostability, samples were pre-incubated at 30–90 °C for 1 h and then cooled on ice for at least 30 min. Samples were analyzed at 40 °C. The A232 value increased to 5.5 following the production of 1 mM unsaturated uronic acid. One unit of lyase activity was defined as the amount of enzyme that produced 1 μmol oligo sugars containing unsaturated uronic acid per minute. In this study, we calculated the units using 300 cps alginate at 40 °C. A UV-2600 spectrophotometer was used to measure lyase activity (Shimadzu Corporation).
For SEC analysis, a 1-mL reaction solution consisting of 0.1 M Tris–HCl buffer (pH 7.0), 0.25% (v/v) sodium alginate (300 cps), and 5 μL (0.02 U) enzyme was prepared. Samples were degraded at 30 °C for 1 h, after which the reaction was stopped by an incubation at 80 °C for 5 min. Degraded products were detected using a high-performance liquid chromatography (HPLC) system with a refractive index detector (Shimadzu Corporation) coupled with a TSK-gel GMPW XL mixed bed scouting column (Tosoh Bioscience LLC, King of Prussia, PA, USA).
Mass spectrometry
The molecular weight of the purified enzyme was determined by matrix-assisted laser desorption/ionization-time of flight-mass spectrometry (MALDI-TOF-MS) (AutoFLEX; Bruker Corporation, Billerica, MA, USA). Samples were prepared using ZipTip® Pipette Tips (C-18) and were mixed with sinapic acid (Sigma-Aldrich Corporation, St. Louis, MO, USA) as the matrix. All measurements were conducted in the linear mode.
The degradation products of sodium alginate were examined by electrospray ionization mass spectrometry (ESI-MS) (Xcalibur; Thermo Fisher Scientific, Waltham, MA, USA). The ESI-MS analysis was conducted in the negative mode. To prepare samples, reaction mixtures were incubated at 30 °C for 15 min, 110 min, or 48 h. After centrifugation, the samples were loaded onto a Superdex™ peptide 10/300 GL prepacked column for high-resolution SEC (GE Healthcare). The A232 values were monitored to separate the degradation products from AlgC-PL7 and unreacted sodium alginate. Finally, the collected fractions were loaded onto an Amide-80 column (Tosoh Bioscience LLC) and the degradation products were eluted using a linear gradient of 95–70% acetonitrile.
Cloning and expression of the algc-pl7 gene
The Cobetia sp. NAP1 genome was analyzed by next-generation sequencing using the HiSeq 2500 Ultra-High-Throughput Sequencing System (Illumina, Inc., San Diego, CA, USA). The algc-pl7 gene and its potential signal sequence were identified and cloned using a specific primer pair (forward: 5′-GGAGATATACATATGAATGACACTCCCCCCGGA-3′ and reverse: 5′-GTGGTGGTGCTCGAGTTATCACTGAATCTTGCCGC-3′). For sub-cloning, the algc-pl7 gene was ligated into the pET-21a expression vector using the In-Fusion HD Cloning Kit (Takara Bio Inc., Shiga, Japan). The expression construct was used to transform E. coli HST08 cells (Takara Bio Inc.). E. coli BL21 (DE3) cells were transformed with the purified pET-21a-algc-pl7 vector and cultured in LB broth containing ampicillin (50 μg/ml final concentration) at 37 °C. Isopropyl β-D-1-thiogalactopyranoside (1 mM final concentration) was added to induce the expression of algc-pl7. Soluble and insoluble forms of the recombinant AlgC-PL7 were produced. The signal sequence was deleted from the recombinant AlgC-PL7. Cells were sonicated in 50 mM Tris–HCl buffer (pH 7.0). After centrifugation, we separated the supernatant and precipitated fractions. The precipitated fraction was re-dissolved in 50 mM Tris–HCl containing 8 M urea buffer (pH 7.0), dialyzed to remove urea, and used for subsequent analyses.
Results
Screening of alginate-degrading micro-organisms
Alginate-degrading bacteria were isolated from the brown algae Padina arborescens Holmes. Although several bacterial colonization and growth rates were observed, we selected a bacterium that exhibited a rapid growth rate (i.e. high utilization) in alginate medium at 30 °C. After isolating the bacterium, genomic DNA was extracted for a subsequent phylogenetic analysis of the 16S rRNA sequence. The alginate-degrading bacterium was identified as a Cobetia sp., which we named Cobetia sp. NAP1. Cobetia spp. were recently separated from Halomonas spp., which are well-characterized halophilic Gram-negative marine bacteria. To determine the optimal growth conditions for Cobetia sp. NAP1, we tested various NaCl concentrations (3–10%), temperatures (20–30 °C), and shaking speeds (100–200 rpm). We then analyzed the enzyme activities of CFEs (data not shown). Based on the results, we decided the optimal conditions for Cobetia sp. NAP1 growth were 3% NaCl, 20 °C, and 180 rpm. To assess the possibility of the presence of extracellular enzymes, we analyzed enzyme activities in the culture medium, but did not detect any.
Purification and analysis of AlgC-PL7 produced from Cobetia sp. NAP1
To examine the degradative activity of Cobetia sp. NAP1, we prepared CFEs and conducted SEC analyses. Control samples were prepared by adding the CFE to the reaction buffer and immediately heating the samples at 80 °C for 5 min to stop the reaction (enzyme inactivation). However, the reaction did not stop after samples were heated. Additionally, the retention times of the heated samples were consistent with those of the non-heated samples, which were reacted for 1 h. These results suggested that a thermostable alginate-degrading enzyme (AlgC-PL7) was present (data not shown). To characterize the AlgC-PL7 produced by Cobetia sp. NAP1, we purified the enzyme from a wild-type strain. The cell lysate was heated at 90 °C for 5 min before being analyzed by ion-exchange chromatography, which enabled us to focus on the thermostable alginate lyase. The supernatant retained lyase activity after being heated, suggesting that AlgC-PL7 was thermostable. During the purification process, the lyase activities of the samples were monitored. Additionally, the purified enzyme was observed to have a molecular weight of about 35 kDa according to SDS-PAGE (Fig. (A)). The MALDI-TOF-MS results revealed that the molecular mass was about 35.4 kDa, which was consistent with the SDS-PAGE estimation. We did not purify other types of alginate lyases, likely because the samples were heated.
Fig. 1. Results of SDS-PAGE and the AlgC-PL7 amino acid sequence.
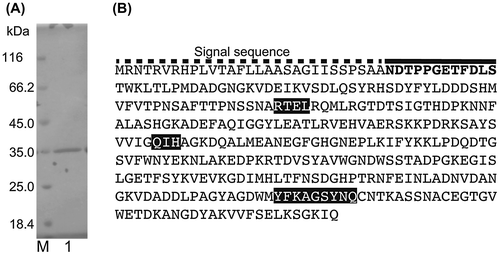
The N-terminal amino acid sequence of purified AlgC-PL7 was determined using an Edman degradation method. The 12 amino acids at the N-terminus were NDTPPGETFDLS (Fig. (B)). Furthermore, a draft Cobetia sp. NAP1 genome sequence was generated by next-generation sequencing. According to these results, we identified the gene encoding AlgC-PL7 and determined the corresponding amino acid sequence (Fig. (B)). Because an additional sequence was detected at the N-terminus, the possibility AlgC-PL7 carries a signal sequence was assessed using the SignalP 4.1 Server (http://www.cbs.dtu.dk/services/SignalP/). The results indicated that AlgC-PL7 contains a signal sequence, suggesting the enzyme is localized to the periplasm. Additionally, the theoretical isoelectric point and molecular weight of AlgC-PL7 lacking a signal sequence were 4.97 and 35.7 kDa, respectively, which were consistent with the SDS-PAGE and MALDI-TOF-MS results. Based on the draft genome sequence and a BLAST search (http://blast.ncbi.nlm.nih.gov/Blast.cgi), we determined that AlgC-PL7 belongs to the PL-7 family.
Characterization of the optimal temperature and pH for AlgC-PL7 activity
We investigated the effects of temperature and pH on AlgC-PL7 activity. The specific activity was calculated from the slope of the initial velocity and protein concentration. To determine the optimal temperature for AlgC-PL7 activity, lyase activity was measured at 20–55 °C. The results revealed that maximum enzyme activity occurred at approximately 45 °C (Fig. (A)). Although the optimal pH was approximately 8.0, AlgC-PL7 retained half of its lyase activity from pH 6 to 9 (Fig. (B)). These results suggest that AlgC-PL7 activity is generally optimal under neutral conditions.
Fig. 2. Effects of temperature, pH, and salt on AlgC-PL7 activity.
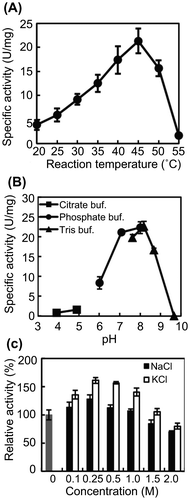
Effects of salts on AlgC-PL7 activity
Cobetia sp. NAP1 was able to grow in the presence of high NaCl concentrations (10–12.5%). Marine bacteria generally prefer NaCl concentrations of 0.2–5%. Thus, Cobetia sp. NAP1 was classified as a halobacterium in the family Halomonadaceae. Cobetia sp. NAP1 is thought to produce salt-tolerant alginate-degrading enzymes. Therefore, we used sodium alginate as the sole carbon source. To confirm this hypothesis, the effects of various concentrations of NaCl and KCl on AlgC-PL7 lyase activity were examined. AlgC-PL7 exhibited the highest lyase activity at 0.25 M NaCl and KCl, with higher activity levels in the presence of KCl than in NaCl (Fig. (C)). As expected, about 70% of the activity was retained at 2.0 M NaCl and KCl, indicating that AlgC-PL7 is a salt-tolerant enzyme. We also examined the effects of metals on AlgC-PL7 activity, and observed that AlgC-PL7 is strongly inhibited by Cu2+ (data not shown). A similar result was observed for another alginate lyase (Aly-W02).Citation25)
Thermostability of AlgC-PL7
We added a heat-treatment step to the protein purification procedure because we were interested in thermostable alginate lyases. However, because the cell lysate was incubated at 90 °C for 5 min, it was unclear whether AlgC-PL7 was thermostable. Therefore, to assess the thermostability of AlgC-PL7, the enzyme was incubated at various temperatures for 1 h, after which lyase activity was measured. Approximately 80% of the AlgC-PL7 activity was maintained up to 70 °C (Fig. (A)). About 60 and 30% of the AlgC-PL7 activity were retained following incubations at 80 and 90 °C for 1 h, indicating that AlgC-PL7 was thermostable. We also recorded time-lapse measurements of lyase activity at 70–90 °C. More than 90% of the AlgC-PL7 activity was retained after heating samples at 70 and 80 °C for 15 min (Fig. (B)), while activities decreased to about 80% after heating at 90 °C for 15 min. These results imply that AlgC-PL7 is a novel thermostable alginate lyase (see Discussion).
Fig. 3. Thermostability of Cobetia sp. NAP1-produced AlgC-PL7 and recombinant AlgC-PL7.
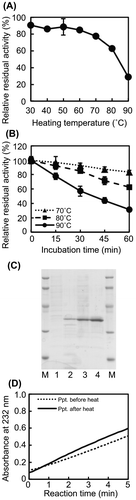
We also confirmed the thermostability of recombinant AlgC-PL7 produced in E. coli. The cell lysate was separated into supernatant and precipitated fractions after a sonication step. Recombinant AlgC-PL7 was produced in E. coli BL21 (DE3) cells in soluble and insoluble forms. The precipitated fraction was dissolved in urea buffer and then dialyzed to remove the urea. The results of a comparison of the supernatant and precipitated fractions before and after the heat treatment are presented in Fig. (C). The protein band corresponding to soluble recombinant AlgC-PL7 (i.e. supernatant fraction) from heat-treated samples was clearly visible in the stained SDS-PAGE gel (Fig. (C), lanes 1 and 2). Because experiments were performed using the same protein concentrations for samples collected before and after the heat treatment, the recombinant AlgC-PL7 was concentrated by the removal of non-thermostable proteins following heat treatment. The protein bands representing the recombinant AlgC-PL7 (approximately 35 kDa) were clearly observed, indicating the heat treatment did not affect enzyme size. Moreover, the lyase activity retained after the heat treatment offered strong evidence that AlgC-PL7 is thermostable (Fig. (D)).
Substrate specificity of AlgC-PL7
Regarding substrate specificity, alginate lyase is usually classified as polyM-specific or polyG-specific. However, a polyMG-specific alginate lyase was recently described.Citation26) To clarify the substrate specificity of AlgC-PL7, polyM, polyG, and polyMG was synthesized and a lyase activity assay involving each substrate was performed. Although AlgC-PL7 was most active against polyMG, it also exhibited some activity against polyM and polyG (Fig. (A)). The same activities were also observed for recombinant AlgC-PL7 (Fig. (B)). These results indicate that AlgC-PL7 has broad substrate specificity. However, the specific activity of recombinant AlgC-PL7 was higher than that of Cobetia sp. NAP1-produced AlgC-PL7, likely because of differences in enzyme stability during purification. The purification of Cobetia sp. NAP1-produced AlgC-PL7 required 3–4 weeks, while the recombinant AlgC-PL7 was purified in 1 week because it was highly expressed in an E. coli system. Therefore, this difference in purification time likely influenced the activities of each enzyme.
Fig. 4. Substrate specificity of Cobetia sp. NAP1-produced AlgC-PL7 (A) and recombinant AlgC-PL7 (B).
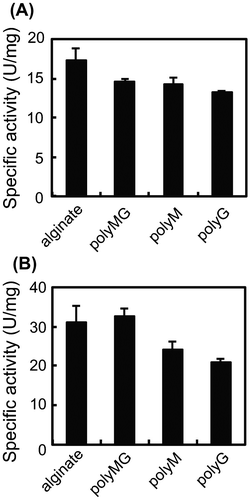
Detection of alginate components degraded by AlgC-PL7
To obtain monosaccharides from alginate, various alginate-degrading enzymes (e.g. polyM-, polyG-, or polyMG-specific, and exo- or endo-types) are usually required. Because AlgC-PL7 exhibits broad substrate specificity, we examined the products resulting from the degradation of alginate by this enzyme. After incubations for 15 min, 110 min, or 48 h, fractions collected by HPLC were analyzed by ESI-MS (Fig. ). At 15 min, mono-, di-, tri-, and tetrasaccharides were not detected, but pentasaccharides were observed (Fig. (A)). After 110 min, five peaks were detected (i.e. mono-, di-, tri-, tetra-, and pentasaccharides) (Fig. (B)). Finally, after 48 h, ESI-MS analysis revealed two major peaks (m/z: 175.024 and 351.056), indicating that the final degraded products are mono- and disaccharides. Additionally, the m/z values of the peaks were consistent with those of previous reports (Fig. (C)).Citation27–29) Our findings indicated that the degradation of alginate by AlgC-PL7 produces mono- and disaccharides.
Fig. 5. ESI-MS analysis of the degradation products resulting from AlgC-PL7 activity.
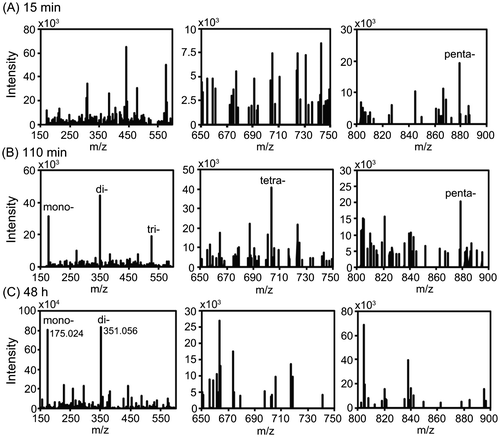
Discussion
Alginate lyases of Cobetia sp. NAP1
Many alginate lyases have been isolated from various sources and characterized. Among marine bacteria, alginate lyases from Vibrio sp., Pseudomonas sp., and Sphingomonas sp. have been thoroughly studied.Citation22,28,30–35) Recently, halophilic Cobetia spp. were separated from Halomonas spp. in the family Halomonadaceae based on the results of genomic analyses.Citation36,37) Furthermore, although Cobetia sp., Vibrio sp., and Pseudomonas sp. are members of the class Gammaproteobacteria, Cobetia spp. form unique bacterial communities that have dissimilar compositions according to PCoA and Bray–Curtis analyses.Citation38) An investigation of alginate-degrading marine bacteria revealed that relatively few alginate lyases have been isolated from members of the family Halomonadaceae.Citation39,40) Thus, our findings provide new insights into the alginate lyases of Halomonadaceae species. Additionally, an analysis of the draft genome sequence indicated Cobetia sp. NAP1 produces one PL-15 (80 kDa) and two PL-17 (80 and 97 kDa) enzymes. We also detected a hypothetical protein (30 kDa) whose amino acid sequence is similar to that of a PL according to a BLAST search. Although we identified AlgC-PL7, which contains a QIH sequence, we were unable to detect the other type of PL-7 enzyme that has a QVH sequence. To fully characterize the mechanism underlying alginate degradation by Cobetia sp. NAP1, a more thorough investigation of these enzymes and related proteins will be necessary.
Salt tolerance of AlgC-PL7
AlgC-PL7 exhibited salt tolerance in the presence of NaCl and KCl (Fig. (C)). Several salt-tolerant alginate lyases have been reported for various marine bacteria, including Vibrio sp., Pseudoalteromonas sp., Isoptericola halotolerans, and Agarivorans sp.Citation26,41–43) More recently, novel thermostable and salt-tolerant alginate lyases were isolated from Halomonas sp. and Cobetia sp.Citation25,40) A comparison of these enzymes as well as AlgC-PL7 and alginate lyases from Halomonas sp. (OalY1 and OalY2) and Cobetia sp. (Aly-W02) indicated that Aly-W02 is more salt-tolerant than AlgC-PL7, and the properties of OalY1 and OalY2 are similar to those of AlgC-PL7. The optimal salt concentrations for Aly-W02 were 0.8 M NaCl and 1.5 M KCl, whereas those for AlgC-PL7 were 0.25 M for both NaCl and KCl.Citation25) However, AlgC-PL7 retained up to 80% of its activity at 2.0 M NaCl and KCl, suggesting that these differences resulted from the intracellular localization of the enzyme. Predictions according to signal sequence analysis suggested that AlgC-PL7 is located in the periplasm (Fig. ), while Aly-W02 is secreted into the extracellular spaces. Thus, extracellular Aly-W02 is considered to be more salt-tolerant than AlgC-PL7. Halomonas spp. and Cobetia spp. are halophilic Gram-negative bacteria, which may produce halophilic enzymes.
Acidic amino acids tend to be relatively abundant in halophilic enzymes. Thus, we analyzed the acidic amino acid contents (i.e. number of Asp and Glu/total number of amino acids) of the following alginate lyases: AlgC-PL7 (14.2%, 50/352), Corynebacterium sp. ALY-1 AlyPG (GenBank™ accession number: AB030481) (9%, 23/256), Sphingomonas sp. A1 alginate lyase (GenBank™ accession number: BAB03312) (10.9%, 70/641), Klebsiella pneumoniae AlyA (GenBank™ accession number: L19657) (10.4%, 32/307), Pseudoalteromonas sp. IAM14594 AlyPEEC (GenBank™ accession number: AF082561) (12%, 48/398), Photobacterium sp. ATCC 43367 polyM lyase (GenBank™ accession number: CAA49630) (10.2%, 29/285), Gayadomonas joobiniege alginate lyase (NCBI reference sequence: WP_017446425) (11.2%, 41/367), and Catenovulum agarivorans alginate lyase (NCBI reference sequence: WP_035013234) (12%, 44/367). AlgC-PL7 was observed to have the highest proportion of acidic amino acids. Therefore, AlgC-PL7 is considered to be salt-tolerant. Additionally, the alginate lyases of halophilic Gram-negative bacteria have high Asp and Glu contents [e.g. Halomonas sp. Victoria JH alginate lyase (GenBank™ accession number: ANB32492) (16.1%, 55/342) and Cobetia crustatorum alginate lyase (NCBI reference sequence: WP_024952190) (15.9%, 56/352)], suggesting Asp and Glu contents may be useful for predicting protein salt tolerance levels.
Thermostability of AlgC-PL7
The Cobetia sp. NAP1-produced AlgC-PL7 and recombinant AlgC-PL7 were tolerant to temperatures up to 90 °C (Fig. ). We also isolated another alginate-degrading bacterium, Shewanella sp., using the same methods, but the alginate lyase from this bacterium was not thermostable at temperatures ≥40 °C (unpublished data). This finding suggests that among the alginate lyases, thermotolerance is unique to AlgC-PL7. However, two other thermotolerant alginate lyases have been isolated from marine bacteria. One was detected in the gut of the red sea bream, Pagrus major, while the other was identified in Halomonas sp.Citation40,44) Poly (α-L-guluronate) lyase (PG lyase) shares similar characteristics to those of AlgC-PL7. An investigation of its thermostability revealed that about 90, 80, and 45% of PG lyase activity was retained after a 15 min incubation at 70, 80, and 90 °C, respectively.Citation44) In contrast, we observed that approximately 95, 90, and 80% of AlgC-PL7 activity was retained after a 15 min incubation at 70, 80, and 90 °C, respectively (Fig. (B)). Additionally, OalY1 and OalY2, which are oligoalginate lyases derived from Halomonas sp., retained about 40% of their activities after a 1 h incubation at 50 °C.Citation40) AlgC-PL7 retained about 90% of its activity under the same conditions. Furthermore, although a Vibrio sp. PL7-type lyase (i.e. A9mT) was reported to be thermostable, it was less active than AlgC-PL7.Citation41) Thus, our results indicate that AlgC-PL7 is a novel thermostable alginate lyase.
We detected structural differences between AlgC-PL7 and the other PL-7 alginate lyases based on the secondary structures of AlgC-PL7, Sphingomonas sp. A1 Al-II′ (PDB ID: 2CWS), Vibrio halioticoli AlyVGI (GenBank™ accession number: AAF22512), P. aeruginosa PA1167 (GenBank™ accession number: AAG04556), K. pneumoniae AlyA, and Corynebacterium sp. ALY-1 AlyPG predicted by CRNPRED (http://pdbj.org/crnpred/).Citation45) The PL-7 alginate lyases have several conserved sequences and structural similarities.Citation46–48) Two short flexible loops (L1 and L2) are important for catalytic activity.Citation32) The L2 loop follows the QIH or QVH sequences, which are involved in substrate recognition. Secondary structure predictions suggested this region consists of a QIH (QVH) – coil – β-strand – coil – β-strand structure in the PL-7 alginate lyases, except for AlgC-PL7. Additionally, the predicted structure of this region in Sphingomonas sp. A1 Al-II′ is consistent with the crystal structure.Citation47) In contrast, the predicted AlgC-PL7 secondary structure indicated that an α-helix is present in this region (QIH – α-helix – coil – β-strand – coil – β-strand). The presence of α-helices can enhance structural stability, and known thermotolerant proteins have high α-helix contents.Citation49,50) We examined the abundance of amino acids related to thermostability (i.e. Arg, Asn, Gln, Glu, Lys, Ser, and Thr),Citation51) but ultimately concluded that most of the differences between AlgC-PL7 and the other PL-7 alginate lyases are related to the L2 loop structure. Therefore, the thermostability of AlgC-PL7 may be associated with the inclusion of an α-helix.
Mechanism underlying the degradative activities of AlgC-PL7
We observed that AlgC-PL7 exhibits broad substrate specificity and the ability to degrade alginate into monosaccharides. Several common properties of PL7-type lyases have been confirmed by statistical and structural analyses, and suggest that conserved amino acid sequences influence substrate specificity.Citation21,32,41) The PL-7 lyases have two important amino acid sequences (QVH and QIH) that are thought to dictate substrate specificity (i.e. QVH: polyM-specific, QIH: polyG- and polyMG-specific). AlgC-PL7 has a conserved QIH sequence, implying it is a polyG lyase. Nevertheless, our results indicated that AlgC-PL7 has broad substrate specificity and the same specific activity for each substrate. However, AlgC-PL7 is not highly active. The A1-II′ enzyme of Sphingomonas sp. A1 has a globe-like conformation, with several conserved sequences, a hydrogen network, and L1 and L2 loops.Citation32) The flexibility of L1 and L2 is important for substrate uptake. Based on predicted secondary structures, AlgC-PL7 is similar to the other PL-7 alginate lyases except regarding the L2 loop. We concluded that the mobility of AlgC-PL7 L2 is regulated by an α-helix. Moreover, it may affect the local structures (e.g. cavity) and hydrogen networks, which may influence the broad substrate specificity of AlgC-PL7. Therefore, the α-helix may decrease AlgC-PL7 activity, but increase protein stability.
We searched for other Cobetia sp. NAP1 alginate lyases using the annotated genome sequence, but were unable to detect any other QVH sequences, which are characteristic of PL-7 enzymes. In general, PL-7 lyases depolymerize alginate into oligoalginate regardless of whether QIH or QVH is present. However, we detected only one PL-7 lyase (QIH) in Cobetia sp. NAP1. Thus, AlgC-PL7 is considered to have bifunctional lyase activities against alginate instead of being specific for QVH-containing alginate. Other bifunctional alginate lyases have been isolated from I. halotolerans sp. and Pseudoalteromonas sp.Citation26,52)
AlgC-PL7 produces monosaccharides from alginate. Therefore, we conducted a time-lapse analysis of the products resulting from AlgC-PL7 activity. After a 15 min reaction, we were unable to detect a monosaccharide peak, although some oligosaccharide peaks were detected (Fig. (A)). However, mono- and oligosaccharide peaks were detected after 110 min, suggesting that AlgC-PL7 degrades alginate endolytically into monosaccharides (Fig. (B)). Considering these results, trisaccharides are the smallest unit required for AlgC-PL7 activity. Although AlgC-PL7 can degrade trisaccharides into mono- and disaccharides, it cannot degrade disaccharides into monosaccharides. Therefore, there are likely more disaccharides than monosaccharides among the final degradation products. However, monosaccharide production from marine biomass resources is important.
To compare degradation mechanisms, we examined the products resulting from the activities of the polyG-specific PL7-type lyase derived from our isolated Shewanella sp. However, monosaccharides were not detected (unpublished data). Moreover, Aly-W02, which is a polyM-specific lyase derived from Cobetia sp. WG-007, releases di-, tri-, and tetrasaccharides from alginate and polyG.Citation25) Although the detailed mechanisms underlying alginate degradation by AlgC-PL7 are still unclear, AlgC-PL7 is a promising enzyme for alginate degradation. Structural analyses may be useful for characterizing the degradation mechanism, as has been demonstrated for A1-II′ of Sphingomonas sp. A1.Citation30,32) Therefore, we will analyze the AlgC-PL7 structure in future studies.
Author contributions
H.Y. and T.O. designed the study. H.Y., A.F., and N.I. conducted the experiments. H.Y. and A.F. analyzed the data. H.Y. and T.O. wrote the manuscript.
Disclosure statement
No potential conflict of interest was reported by the authors.
Funding
This work was supported by the Takeda Science Foundation, the Uehara Memorial Foundation, and the Ministry of Education, Science, Sports, and Culture of Japan [Grant for Scientific Research, 15K18490 (2015–2016)].
Notes
Abbreviations: CFE, cell free extract; ESI-MS, electrospray ionization mass spectrometry; HPLC, high-performance liquid chromatography; MALDI-TOF-MS, matrix-assisted laser desorption/ionization-time of flight-mass spectrometry; polyG, polyguluronic acid-rich region; polyM, polymannuronic acid-rich region; PL, polysaccharide lyase; polyMG, random region; SEC, size exclusion chromatography; SDS-PAGE, sodium dodecyl sulfate-polyacrylamide gel electrophoresis.
References
- Wargacki AJ, Leonard E, Win MN, et al. An engineered microbial platform for direct biofuel production from brown macroalgae. Science. 2012;335:308–313.10.1126/science.1214547
- Beer LL, Boyd ES, Peters JW, et al. Engineering algae for biohydrogen and biofuel production. Curr. Opin. Biotechnol. 2009;20:264–271.10.1016/j.copbio.2009.06.002
- Chisti Y. Biodiesel from microalgae beats bioethanol. Trends Biotechnol. 2008;26:126–131.10.1016/j.tibtech.2007.12.002
- Gasesa P. Alginates. Carbohydr. Polym. 1988;8:161–182.10.1016/0144-8617(88)90001-X
- Evans LR, Linker A. Production and characterization of the slime polysaccharide of Pseudomonas aeruginosa. J. Bacteriol. 1973;116:915–924.
- Costerton JW, Stewart PS, Greenberg EP. Bacterial biofilms: a common cause of persistent infections. Science. 1999;284:1318–1322.10.1126/science.284.5418.1318
- Takase R, Ochiai A, Mikami B, et al. Molecular identification of unsaturated uronate reductase prerequisite for alginate metabolism in Sphingomonas sp. A1. Biochim. Biophys. Acta. 2010;1804:1925–1936.10.1016/j.bbapap.2010.05.010
- Enquist-Newman M, Faust AM, Bravo DD, et al. Efficient ethanol production from brown macroalgae sugars by a synthetic yeast platform. Nature. 2014;505:239–243.
- Tusi SK, Khalaj L, Ashabi G, et al. Alginate oligosaccharide protects against endoplasmic reticulum- and mitochondrial-mediated apoptotic cell death and oxidative stress. Biomaterials. 2011;32:5438–5458.10.1016/j.biomaterials.2011.04.024
- Wang P, Jiang X, Jiang Y, et al. In vitro antioxidative activities of three marine oligosaccharides. Nat. Prod. Res. 2007;21:646–654.10.1080/14786410701371215
- Liu S, Liu G, Yi Y. Novel vanadyl complexes of alginate saccharides: synthesis, characterization, and biological activities. Carbohydr. Polym. 2015;121:86–91.10.1016/j.carbpol.2014.11.069
- Burana-osot J, Hosoyama S, Nagamoto Y, et al. Photolytic depolymerization of alginate. Carbohydr. Res. 2009;344:2023–2027.10.1016/j.carres.2009.06.027
- Uno T, Hattori M, Yoshida T. Oral administration of alginic acid oligosaccharide suppresses IgE production and inhibits the induction of oral tolerance. Biosci. Biotechnol. Biochem. 2006;70:3054–3057.10.1271/bbb.60391
- Tomoda Y, Umemura K, Adachi T. Promotion of barley root elongation under hypoxic conditions by alginate lyase-lysate (A.L.L.). Biosci. Biotechnol. Biochem. 1994;58:202–203.10.1271/bbb.58.202
- Xu X, Iwamoto Y, Kitamura Y, et al. Root growth-promoting activity of unsaturated oligomeric uronates from alginate on carrot and rice plants. Biosci. Biotechnol. Biochem. 2003;67:2022–2025.10.1271/bbb.67.2022
- Wong TY, Preston LA, Schiller NL. Alginate lyase: review of major sources and enzyme characteristics, structure-function analysis, biological roles, and applications. Annu. Rev. Microbiol. 2000;54:289–340.10.1146/annurev.micro.54.1.289
- Garron ML, Cygler M. Structural and mechanistic classification of uronic acid-containing polysaccharide lyases. Glycobiology. 2010;20:1547–1573.10.1093/glycob/cwq122
- Kim HT, Chung JH, Wang D, et al. Depolymerization of alginate into a monomeric sugar acid using Alg17C, an exo-oligoalginate lyase cloned from Saccharophagus degradans 2-40. Appl. Microbiol. Biotechnol. 2012;93:2233–2239.10.1007/s00253-012-3882-x
- Park HH, Kam N, Lee EY, et al. Cloning and characterization of a novel oligoalginate lyase from a newly isolated bacterium Sphingomonas sp. MJ-3. Mar. Biotechnol. 2012;14:189–202.10.1007/s10126-011-9402-7
- Jagtap SS, Hehemann JH, Polz MF, et al. Comparative biochemical characterization of three exolytic oligoalginate lyases from Vibrio splendidus reveals complementary substrate scope, temperature, and pH adaptations. Appl. Environ. Microbiol. 2014;80:4207–4214.10.1128/AEM.01285-14
- Zhu B, Yin H. Alginate lyase: review of major sources and classification, properties, structure-function analysis and applications. Bioengineered. 2015;6:125–131.10.1080/21655979.2015.1030543
- Hashimoto W, Miyake O, Momma K, et al. Molecular identification of oligoalginate lyase of Sphingomonas sp. strain A1 as one of the enzymes required for complete depolymerization of alginate. J. Bacteriol. 2000;182:4572–4577.10.1128/JB.182.16.4572-4577.2000
- Takagi T, Yokoi T, Shibata T, et al. Engineered yeast whole-cell biocatalyst for direct degradation of alginate from macroalgae and production of non-commercialized useful monosaccharide from alginate. Appl. Microbiol. Biotechnol. 2016;100:1723–1732.10.1007/s00253-015-7035-x
- Haug A, Larsen B, Smidsrød O. Studies on the sequence of uronic acid residues in alginic acid. Acta Chem. Scand. 1967;21:691–704.10.3891/acta.chem.scand.21-0691
- Gong JS, Liu XM, Zhang MJ, et al. Purification and characterization of a high salt-tolerant alginate lyase from Cobetia sp. WG-007. Biotechnol. Appl. Biochem. 2016. doi:10.1002/bab.1506
- Dou W, Wei D, Li H, et al. Purification and characterisation of a bifunctional alginate lyase from novel Isoptericola halotolerans CGMCC 5336. Carbohydr. Polym. 2013;98:1476–1482.10.1016/j.carbpol.2013.07.050
- Chaki T, Kakimi H, Shibata A, et al. Detection of alginate oligosaccharides from mollusks. Biosci. Biotechnol. Biochem. 2006;70:2793–2796.10.1271/bbb.60313
- Ryu M, Lee E. Saccharification of alginate by using exolytic oligoalginate lyase from marine bacterium Sphingomonas sp. MJ-3. J. Ind. Eng. Chem. 2011;17:853–858.10.1016/j.jiec.2011.08.001
- Swift SM, Hudgens JW, Heselpoth RD, et al. Characterization of AlgMsp, an alginate lyase from Microbulbifer sp. 6532A. PLoS ONE. 2014;9:e112939.10.1371/journal.pone.0112939
- Miyake O, Ochiai A, Hashimoto W, et al. Origin and diversity of alginate lyases of families PL-5 and -7 in Sphingomonas sp. strain A1. J. Bacteriol. 2004;186:2891–2896.10.1128/JB.186.9.2891-2896.2004
- Fu X, Lin H, Kim S. Purification and characterization of a Na+/K+ dependent alginate lyase from turban shell gut Vibrio sp. YKW-34. Enzyme Microb. Technol. 2007;41:828–834.10.1016/j.enzmictec.2007.07.003
- Ogura K, Yamasaki M, Mikami B, et al. Substrate recognition by family 7 alginate lyase from Sphingomonas sp. A1. J. Mol. Biol. 2008;380:373–385.10.1016/j.jmb.2008.05.008
- Farrell EK, Tipton PA. Functional characterization of AlgL, an alginate lyase from Pseudomonas aeruginosa. Biochemistry. 2012;51:10259–10266.10.1021/bi301425r
- Zhu BW, Huang LS, Tan HD, et al. Characterization of a new endo-type polyM-specific alginate lyase from Pseudomonas sp. Biotechnol. Lett. 2015;37:409–415.10.1007/s10529-014-1685-0
- Zhu B, Tan H, Qin Y, et al. Characterization of a new endo-type alginate lyase from Vibrio sp. W13. Int. J. Biol. Macromol. 2015;75:330–337.10.1016/j.ijbiomac.2015.01.053
- Arahal DR, Castillo AM, Ludwig W, et al. Proposal of Cobetia marina gen. nov., comb. nov., within the Family Halomonadaceae, to include the species Halomonas marina. Syst. Appl. Microbiol. 2002;25:207–211.10.1078/0723-2020-00113
- Kim MS, Roh SW, Bae JW. Cobetia crustatorum sp. nov., a novel slightly halophilic bacterium isolated from traditional fermented seafood in Korea. Int. J. Syst. Evol. Microbiol. 2010;60:620–626.10.1099/ijs.0.008847-0
- Martin M, Barbeyron T, Martin R, et al. The cultivable surface microbiota of the brown alga Ascophyllum Nodosum is enriched in macroalgal-polysaccharide-degrading bacteria. Front. Microbiol. 2015;6:1–14. Article 1487.
- Kraiwattanapong J, Tsuruga H, Ooi T, et al. Cloning and sequencing of a Deleya marina gene encoding for alginate lyase. Biotechnol. Lett. 1999;21:169–174.10.1023/A:1005435725903
- Yang X, Li S, Wu Y, et al. Cloning and characterization of two thermo- and salt-tolerant oligoalginate lyases from marine bacterium Halomonas sp. FEMS Microbiol. Lett. 2016;363:1–7.
- Uchimura K, Miyazaki M, Nogi Y, et al. Cloning and sequencing of alginate lyase genes from deep-sea strains of vibrio and agarivorans and characterization of a New Vibrio Enzyme. Mar. Biotechnol. 2010;12:526–533.10.1007/s10126-009-9237-7
- Wang Y, Guo EW, Yu WG, et al. Purification and characterization of a new alginate lyase from a marine bacterium Vibrio sp. Biotechnol. Lett. 2013;35:703–708.10.1007/s10529-012-1134-x
- Kobayashi T, Uchimura K, Miyazaki M, et al. A new high-alkaline alginate lyase from a deep-sea bacterium Agarivorans sp. Extremophiles. 2009;13:121–129.10.1007/s00792-008-0201-7
- Takeshita S, Sato N, Igarashi M, et al. A highly denaturant-durable alginate lyase from a marine bacterium: purification and properties. Biosci. Biotechnol. Biochem. 1993;57:1125–1128.10.1271/bbb.57.1125
- Kinjo RA, Nishikawa K. Predicting secondary structures, contact numbers, and residue-wise contact orders of native protein structures from amino acid sequences using critical random networks. Biophysics. 2005;1:67–74.10.2142/biophysics.1.67
- Yamasaki M, Moriwaki S, Miyake O, et al. Structure and function of a hypothetical Pseudomonas aeruginosa protein PA1167 classified into family PL-7: a novel alginate lyase with a beta-sandwich fold. J. Biol. Chem. 2004;279:31863–31872.10.1074/jbc.M402466200
- Yamasaki M, Ogura K, Hashimoto W, et al. A structural basis for depolymerization of alginate by polysaccharide lyase family-7. J. Mol. Biol. 2005;352:11–21.10.1016/j.jmb.2005.06.075
- Osawa T, Matsubara Y, Muramatsu T, et al. Crystal structure of the alginate (poly alpha-l-guluronate) lyase from Corynebacterium sp. at 1.2 A resolution. J. Mol. Biol. 2005;345:1111–1118.10.1016/j.jmb.2004.10.081
- Petukhov M, Kil Y, Kuramitsu S, et al. Insights into thermal resistance of proteins from the intrinsic stability of their alpha-helices. Proteins. 1997;29:309–320.10.1002/(ISSN)1097-0134
- Facchiano AM, Colonna G, Ragone R. Helix stabilizing factors and stabilization of thermophilic proteins: an X-ray based study. Protein Eng. 1998;11:753–760.10.1093/protein/11.9.753
- Fukuchi S, Nishikawa K. Protein surface amino acid compositions distinctively differ between thermophilic and mesophilic bacteria. J. Mol. Biol. 2001;309:835–843.10.1006/jmbi.2001.4718
- Li JW, Dong S, Song J, et al. Purification and characterization of a bifunctional alginate lyase from Pseudoalteromonas sp. SM0524. Mar. Drugs. 2011;9:109–123.10.3390/md9010109