Abstract
In peaches, fruit flesh browns unattractively after peeling or cutting. A recently developed cultivar, Okayama PEH7, was distinct from other Japanese cultivars, including Okayama PEH8, with respect to its reduced browning potential. Homogenate prepared from Okayama PEH7 flesh had significantly less reddening during the browning reaction. Okayama PEH7 had less soluble phenolic compounds and higher polyphenol oxidase activity than Okayama PEH8. Reduced browning was observed even when phenols prepared from Okayama PEH7 were incubated with crude extract from Okayama PEH8, suggesting that phenols lower the browning potential of Okayama PEH7. In Okayama PEH7, contents of chlorogenic acid and its isomers were about one-tenth compared to Okayama PEH8. Exogenous addition of chlorogenic acid to Okayama PEH7 homogenate increased the browning potential and visibly enhanced reddening. These results indicate that the reduced browning of Okayama PEH7 flesh is due to a defect in chlorogenic acid accumulation.
Graphical abstract
Flesh homogenate of Okayama PEH7 had less reddening during the browning reaction owing to a defect in chlorogenic acid accumulation.
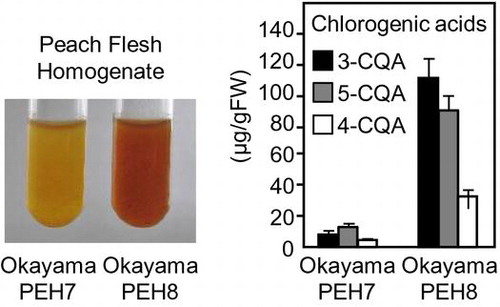
Key words:
The peach (Prunus persica (L.) Batsch) is popular and commercially important worldwide. However, after wounding, peeling, or cutting, the white to orange flesh of the fruit changes to umber within hours.Citation1,2) Flesh browning is an important problem during harvesting, transport, storage, and processing because it negatively affects the appearance and taste of fruit. Industrial processing uses boiling or antioxidants to inhibit flesh browning.Citation3,4) These treatments add to processing times and can be costly, and they can worsen texture and flavor. Therefore, the development of cultivars with less browning potential is desirable.
Enzymatic browning occurs when tissue color changes to brown as a result of oxidative polymerization of phenolic compounds catalyzed by polyphenol oxidase (PPO).Citation5) PPO is a copper-containing enzyme that catalyzes the oxidation of polyphenols to their corresponding quinones,Citation6,7) which are highly reactive and automatically polymerize to a brown polymer.Citation8) Enzymatic browning protects physically damaged tissues from pathogens and herbivores.Citation8) Transgenic tomato and strawberry plants that overexpressed the PPO gene showed enhanced tolerance to bacterial and fungal diseases, respectively.Citation9,10) Transgenic poplars that constantly expressed PPO were resistant to herbivory by forest tent caterpillars.Citation11) Recent studies have demonstrated that PPO activity is closely related to the browning potential of plant tissues. In transgenic potato with reduced PPO activity from the expression of sense and antisense tomato PPO cDNA, tuber browning was reduced.Citation12) Antisense suppression in transgenic apple showed reduced browning in shoot and callus tissues.Citation13,14)
Several studies have described that the browning potential is also related to the level of phenolic compounds.Citation2,15) In Prunus, including peach, the major phenolic compounds are chlorogenic acid isomers (CGAs) and flavonoids. Some studies reported that peach flesh contains two CGAs, chlorogenic acid (5-caffeoylquinic acid, 5-CQA) and neochlorogenic acid (3-caffeoylquinic acid, 3-CQA), whereas cryptochlorogenic acid (4-caffeoylquinic acid, 4-CQA) is scarcely or not detected.Citation2,16) Peach flavonoids are primarily (+)-catechin (CAT), (−)-epicatechin (EPC), and procyanidin B1 (PB1).Citation2,16−18) The composition of phenolic compounds varies among cultivarsCitation15,18,19) and is affected by growing conditions.Citation20)
A recently developed Japanese cultivar, Okayama PEH7, is unusual in that it bears fruit with reduced browning. In this study, we analyzed phenolic contents and PPO activity of Okayama PEH7 by comparing it with a normally browning cultivar.
Materials and methods
Plant materials
Okayama PEH7 is a late-maturing peach which has been recently developed at the Research Institute for Agriculture, Okayama Prefectural Technology Center for Agriculture, Forestry, and Fisheries, Japan. Hakuho, Shimizu-hakuto, and Okayama PEH8 were used to compare browning. All of these varieties are white-flesh peaches, although the flesh around the stone of Okayama PEH7 is red from anthocyanins. Trees of all cultivars used in this study were grown in the orchard of the Research Institute for Agriculture, Okayama Prefectural Technology Center for Agriculture, Forestry, and Fisheries (latitude 35°N, longitude 134°E). Fruits were sampled at the commercial ripening stage. Chopped fruit flesh was frozen in liquid nitrogen and stored at −80 °C until analysis.
Browning of flesh homogenate
The browning potential was estimated according to the method of Cheng and CrisostoCitation15) with a slight modification. Frozen flesh (100 mg) was homogenized in 200 μL of 100 mM sodium phosphate buffer (pH 6.5) at 4 °C, using a Multi-beads Shocker (Yasui kikai, Japan). Homogenates were centrifuged at 20,000 g at 4 °C for 5 min, and the supernatant was incubated at 25 °C for 1 h. After incubation, the absorbance of the supernatant was measured at 490 nm (A490nm, 1 cm in depth) using an Ultrospec 3300 pro spectrophotometer (Amersham Biosciences/GE Healthcare). To inhibit browning reaction during incubation, frozen flesh was boiled for 5 min before homogenization, or homogenized with ascorbic acid (20 mM), glutathione (5 mM), dithiothreitol (5 mM), or 2-mercaptoethanol (1%). The browning potential was calculated as the difference in absorbance between supernatants of boiled samples and incubated experimental samples.
The color of flesh homogenate was measured in a glass cell (2 mm in depth) using a CR-400 Chroma Meter (Konica Minolta, Japan). The color data were reported using the CIE Lab color scale. Five to six replicates were measured from each sample. Tukey’s honestly significant difference (HSD) test was performed with R program for comparing differences between groups (p < 0.05).
Soluble phenolic compounds
Total soluble phenolic compounds were measured with the Folin–Ciocalteu method.Citation21) Frozen flesh (100 mg) was boiled in 200 μL of 100 mM sodium phosphate buffer (pH 6.5) for 5 min, followed by homogenization. Homogenates were centrifuged at 20,000 g at 25 °C for 5 min. A mixture of 6 μL of supernatant, 114 μL of 100 mM sodium phosphate (pH 6.5), 30 μL of Folin–Ciocalteu reagent, and 26.5 μL of 20% sodium carbonate was incubated for 2 h at 25 °C in the dark. Absorbance of 150 μL of the mixture was measured at 765 nm with an Ultrospec 3300 pro. The estimated values were expressed as gallic acid equivalents, using a standard curve made with gallic acid.
PPO activity
PPO activity was determined spectrophotometrically, following a slightly modified protocol from Mayer et al.Citation22) and Nisha et al.Citation23) Frozen flesh (100 mg) was homogenized with 200 μL of 100 mM sodium phosphate buffer (pH 6.5) and centrifuged at 20,000 g at 25 °C for 5 min. The supernatant was incubated at 25 °C for 1 h to exhaust endogenous substrates, and the prepared crude extract was used as the enzyme source. Catechol (1 mM in 100 mM sodium phosphate, pH 6.5) was prepared as the substrate solution. After mixing 15 μL of the enzyme extract and 135 μL of the substrate solution, absorbance (A490nm) of the sample (1 cm in depth) was measured at 30 °C. PPO activity was expressed as the change in A490nm per hour.
High-performance liquid chromatography and mass spectrometry analyses
The components of the soluble phenolic compounds were separated using a LCMS-2020 liquid chromatograph mass spectrometer (Shimadzu, Japan) on a Symmetry C18 column (3.5 μm, 2.1 × 50 mm; Waters; Milford, MA, USA) at 40 °C. The separation was performed within 20 min, using a linear gradient of 0.1% formic acid in water and from 1 to 20% acetonitrile, at a flow rate of 0.3 mL/min. The compounds in peach extracts were detected at 280 or 325 nm. The MS spectra of CGAs, CAT/ECT, and PB1 were detected by electrospray ionization in the negative-ion mode (ESI−) at mass-to-charge (m/z) ratios of 353, 289, and 577, respectively.
Results
Color change of homogenized flesh
When peach flesh was homogenized in buffer, the homogenate began to discolor immediately, and the Okayama PEH8 homogenate was umber within 1 h (Fig. (A)). Okayama PEH7 is distinct from other Japanese cultivars with respect to its lesser degree of browning. Even after 2 h, the Okayama PEH7 homogenate was merely a light yellowish brown. Homogenate discoloration is comparable to that of sliced flesh, so we analyzed the browning reaction of homogenate in the following experiments. Boiling the homogenate before incubation prevented browning in both cultivars.
Fig. 1. Reduced browning phenotype in flesh homogenate of Okayama PEH7.
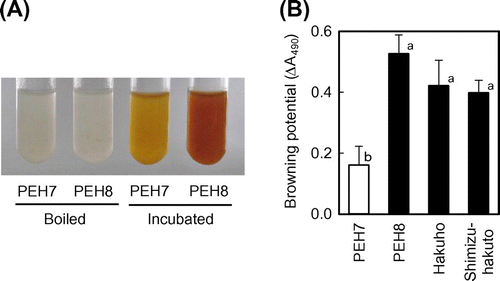
To compare the intensity of discoloration among cultivars, we prepared flesh homogenates from Okayama PEH7, Okayama PEH8, Hakuho, and Shimizu-hakuto. As shown in Table , all of the boiled homogenates showed a similar color: high lightness (L*), little redness (a*), and moderate yellowness (b*). After incubation at 25 °C for 2 h, the redness and yellowness of the homogenates significantly increased in Okayama PEH8, Hakuho, and Shimizu-hakuto. In Okayama PEH7, yellowness highly increased as for the other three cultivars, but remarkably, redness increased only slightly and was not statistically significant. Lightness decreased in Okayama PEH8 but was not significantly different in Okayama PEH7.
Table 1. Color values of flesh homogenate prepared from Japanese peach cultivars.
Browning potential is reduced in Okayama PEH7 flesh
The potential of the enzymatic browning reaction has often been calculated by measuring the absorbance of buffered extract.Citation15) We observed the change in absorbance at 490 nm during incubation. The absorbance increased rapidly just after the incubation started. The change in absorbance gradually declined with time and nearly stopped within 1 h, as previously reported.Citation15) Therefore, we expressed the change in absorbance during the first hour as the browning potential. When we added antioxidants including ascorbic acid, glutathione, dithiothreitol, or 2-mercaptoethanol, which have been shown to inhibit enzymatic browning,Citation24) Okayama PEH8 homogenate did not brown (data not shown). This implies that the enzymatic oxidation of phenolic compounds is primarily responsible for browning. The browning potential of Okayama PEH7 is significantly lower compared to that of Okayama PEH8, Hakuho, and Shimizu-hakuto (Fig. (B)).
Comparison of phenolic compounds and PPO activity
We compared the contents of phenolic compounds and the activity of PPO between Okayama PEH7 and Okayama PEH8 to identify their contributions to the reduction in browning potential in Okayama PEH7. As shown in Fig. (A), Okayama PEH8 contained more than 0.8 mg/g fresh weight (FW) of soluble phenolic compounds, whereas Okayama PEH7 had less than 0.4 mg/g FW. In contrast, PPO activity of Okayama PEH7 was apparently higher than that of Okayama PEH8 (Fig. (B)).
Fig. 2. Comparison of phenolic compounds and PPO activity between Okayama PEH7 and Okayama PEH8.
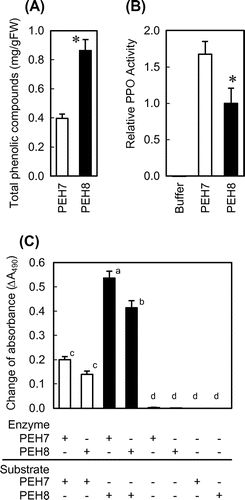
Phenolics prepared from Okayama PEH7 reduce browning
In order to identify the primary factor that limits the browning potential of Okayama PEH7, we analyzed the characteristics of the phenolic compounds and enzyme of Okayama PEH7. We prepared the phenolic compounds and crude extract from flesh of Okayama PEH7 and Okayama PEH8 separately. When we mixed the phenolic compounds prepared from Okayama PEH8 with crude extract from flesh of either Okayama PEH7 or Okayama PEH8, the change in absorbance was strongly induced irrespective of the origin of the enzyme solution (Fig. (C)). On the other hand, when we used the phenolic compounds from Okayama PEH7, the change in absorbance was greatly reduced. These clearly showed that the phenolic compounds from Okayama PEH7 was responsible for the reduction in browning potential. When the crude extract from Okayama PEH7 was used, the change in absorbance was slightly induced compared to when that from Okayama PEH8 was used. This was not consistent with the higher PPO activity of Okayama PEH7 shown in Fig. (B).
Defective CGAs accumulation in Okayama PEH7
The soluble components of the substrate solution were separated using liquid chromatography–mass spectrometry (LC–MS) with a C18 column and the absorption at 280 nm was monitored. Among detected peaks, three (1, 4, and 6) were remarkably lower in Okayama PEH7 than in PEH8 (Fig. (A)). Interestingly, their m/z ratios were approximately identical to 353.3, the expected value from CGAs that are the major polyphenols found in peach flesh. CGAs absorb at 325 nm, so the substrate solution was analyzed at this wavelength. Only peaks 1, 4, and 6 were detected at 325 nm (Fig. (A)). In addition, the retention times of the three peaks matched those of 3-CQA, 5-CQA, and 4-CQA standards, respectively (data not shown). These data indicated that the accumulation of CGAs is greatly reduced in Okayama PEH7. The CGAs contents were estimated using the peak area (Fig. (B)). The concentrations of 3-CQA, 4-CQA, and 5-CQA were approximately 108, 32, and 87 μg/g FW, respectively, in Okayama PEH8. In Okayama PEH7, the total CGAs accumulation was only 25 μg/g FW, about one-tenth of that in Okayama PEH8.
Fig. 3. Analysis of phenolic compounds in fruit flesh.
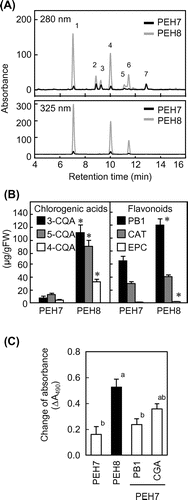
PB1, CAT, and EPC are other major polyphenols found in Prunus flesh. We identified three other peaks in our LC data (2, 3, and 7) as those generated from these three compounds by their m/z ratios and retention times (data not shown). Estimated EPC content was low in both Okayama PEH7 and Okayama PEH8 (Fig. (B)). CAT and PB1 accumulated in both cultivars, but the CAT and PB1 contents in Okayama PEH7 were 74 and 54% of those in Okayama PEH8, respectively. Peak 5 could not be identified in this study.
Effects of exogenous phenolic compounds on browning of Okayama PEH7
To investigate the effects of phenolic composition on browning, we added exogenous phenolic compounds to Okayama PEH7 homogenates and observed color changes after incubation. In the experiment, we added 5-CQA to supplement CGAs to equal the total CGAs content in Okayama PEH8. We also added PB1 to separate samples as a flavonoid representative to equal the total flavonoid content in Okayama PEH8. Added PB1 did not perceptibly change the browning reaction, whereas Okayama PEH7 supplemented with 5-CQA showed significant browning that matched that of Okayama PEH8 (Fig. (C)). As shown in Table , no statistically significant change resulted from PB1 addition. Conversely, the addition of 5-CQA induced reddening to a level that was statistically equal to that of Okayama PEH8, and a slight decrease in lightness was also observed.
Table 2. Color values of flesh homogenate of Okayama PEH7 incubated with PB1 and 5-CQA.
Discussion
The unsightly browning of peach flesh is a serious concern because Japanese consumers prefer white-fleshed fruits for fresh eating. Fresh peaches brown rapidly without artificial processing like boiling or applying antioxidants, so white-fleshed peaches with very little browning potential are desirable. Okayama PEH7, a recently developed cultivar, bears fruit that have less browning. We demonstrated that flesh homogenate from Okayama PEH7 had reduced browning and a low browning potential (Fig. ). Okayama PEH7 had lower levels of soluble phenolic compounds (Fig. (A)) and a higher level of PPO activity (Fig. (B)) compared to Okayama PEH8. The substrate–enzyme substitution experiments showed that browning reduced when substrates were derived from Okayama PEH7 (Fig. (C)). These data clearly indicate that the reduced browning potential of Okayama PEH7 arises from the decrease in phenolic compounds rather than from PPO activity. We used LC–MS to identify the profiles of the CGAs and flavonoids that are major phenolic compounds in fruit flesh of Prunus (Fig. (B)). The flesh of Okayama PEH7 had lower contents of both CGAs and flavonoids than Okayama PEH8. In particular, CGAs were present at approximately one-tenth of the amount in Okayama PEH8. The addition of 5-CQA to flesh homogenate from Okayama PEH7 effectively raised the browning potential to a level that was statistically equivalent to that of Okayama PEH8, while the addition of PB1 was less effective (Fig. (C)). This suggests that the reduced browning potential of Okayama PEH7 is primarily caused by reduced CGAs accumulation. A significant positive correlation between CGAs contents and browning potential was reported from various peach cultivars.Citation15) Amaki et al.Citation25) found that CGAs quinone produced from CGAs by PPO was essential for browning in apples. CGAs may play an important role in the enzymatic browning in peach homogenate.
The color measurements of peach flesh revealed that darkening, reddening, and yellowing are induced during the browning reaction, and that the suppression of reddening and darkening were the primary components of reduced browning in Okayama PEH7 (Table ). The color of products after the PPO reaction depends on substrates.Citation26) Reddening and darkening were enhanced by the addition of exogenous 5-CQA (Table ). On the other hand, PB1 addition did not induce a perceptible color change. These results indicate that CGAs have a key role in reddening and darkening during enzymatic browning. Reddening and darkening strengthen the visual impression of browning in white flesh peach. CGAs depletion or inhibition may be effective ways to suppress peach flesh browning.
Browning potential is a phenotype that is generally regulated by multiple genes. For example, discoloration of potato tubers is genetically regulated by multiple quantitative trait loci (QTLs).Citation27) In apple, several QTLs for polyphenolic compounds and browning potential in fruit were identified.Citation28,29) This suggests that a random cross breeding strategy would not be sufficient to develop a peach that does not brown. DNA markers are sought to select individuals with reduced browning.
The genetic basis of the CGAs defect(s) in Okayama PEH7 is unknown. Genes that encode for or regulate the enzymes involved in the CGAs biosynthetic pathway are likely candidates, but this pathway is not well understood in peach. In Solanaceae, CGAs are formed from caffeoyl-CoA and quinic acid by hydroxycinnamoyl-CoA quinate hydroxycinnamoyl transferase.Citation30) Caffeoyl-CoA may be synthesized from p-coumaroyl CoA via p-coumaroyl shikimic acid and caffeoyl shikimic acid. In sweet potato, CGAs may be synthesized from caffeoyl glucose by UDP glucose:cinnamate glucosyltransferase and hydroxycinnamoyl D-glucose:quinate hydroxycinnamoyl transferase. Identification of the gene(s) responsible for the CGAs defect in Okayama PEH7 will provide valuable information for understanding the CGAs biosynthesis pathway in peach and provide useful DNA markers for breeding cultivars with reduced browning. Okayama PEH7 was produced by crossing between Okayama yume hakuto and Hakurei, both of which show non-astringent phenotype.Citation31–33) An astringent taste is often induced by phenolic compounds including tannin. Detailed analysis of these cultivars may help the understanding of genetic basis of the lower phenolic accumulation in Okayama PEH7.
While Okayama PEH7 flesh does not brown strongly owing to deficient CGAs accumulation, the fruit still becomes a light yellowish brown after peeling. This yellowing might be caused by oxidation of endogenous flavonoids, the other major polyphenols in peach flesh. Hence, introducing a novel mechanism to prevent flavonoid accumulation in Okayama PEH7 may produce a highly desirable peach with very little browning potential. Alternatively, browning potential might be reduced by introducing a mechanism to repress PPO activity, which is high in Okayama PEH7 (Fig. (B)).
As described above, Okayama PEH7 has equivalent or more activity of PPO and significantly less contents of CGAs compared to browning peach cultivars. The defect in CGAs accumulation causes reduced browning potential in Okayama PEH7. This finding would provide valuable information and suggestions for future breeding of peach.
Author contributions
NY, MU, SH, and KO conceived the project. NY, MU, TH, and KO designed the experiments. SH prepared the materials. NY, MU, and MH performed the experiments. TH supported biochemical assay. NY, MU, and KO wrote the manuscript.
Disclosure statement
No potential conflict of interest was reported by the author.
Funding
This work was supported by the Tojuro Iijima Foundation for Food Science and Technology; the Japan Society for the Promotion of Science under a Grant-in-Aid for Scientific Research [grant number 16K07610].
Notes
Abbreviations: CGAs, chlorogenic acid isomers; 5-CQA, chlorogenic acid; 3-CQA, neochlorogenic acid; 4-CQA, cryptochlorogenic acid; CAT, (+)-catechin; EPC, (−)-epicatechin; PB1, procyanidin B1; PPO, polyphenol oxidase.
References
- Brandelli A, Lopes C. Polyphenoloxidase activity, browning potential and phenolic content of peaches during postharvest ripening. J. Food Biochem. 2005;29:624–637.10.1111/jfbc.2005.29.issue-6
- Lee CY, Kagan V, Jaworski AW, et al. Enzymic browning in relation to phenolic compounds and polyphenoloxidase activity among various peach cultivars. J. Agric. Food Chem. 1990;38:99–101.10.1021/jf00091a019
- Vámos-Vigyázó L. Polyphenol oxidases and peroxidases in fruits and vegetables. Crit. Rev. Food Sci. Nutr. 1981;15:49–127.10.1080/10408398109527312
- Ávila IMLB, Silva CLM. Modelling kinetics of thermal degradation of colour in peach puree. J. Food Eng. 1999;39:161–166.10.1016/S0260-8774(98)00157-5
- Vaughn KC, Duke SO. Function of polyphenol oxidase in higher plants. Physiol. Plant. 1984;60:106–112.10.1111/ppl.1984.60.issue-1
- Mayer AM. Polyphenol oxidases in plants and fungi: going places? A review. Phytochemistry. 2006;67:2318–2331.10.1016/j.phytochem.2006.08.006
- Sullivan ML. Beyond brown: polyphenol oxidases as enzymes of plant specialized metabolism. Front Plant Sci. 2015;5:783. doi: 10.3389/fpls.2014.00783
- Duffey SS, Felton GW. Enzymatic antinutritive defenses of the tomato against insects. In: Hedin PA, editor. Naturally occurring pest bioregulators. Washington (DC): American Chemical Society; 1991. p. 166–197.10.1021/symposium
- Li L, Steffens JC. Overexpression of polyphenol oxidase in transgenic tomato plants results in enhanced bacterial disease resistance. Planta. 2002;215:239–247.10.1007/s00425-002-0750-4
- Jia H, Zhao P, Wang B, et al. Overexpression of polyphenol oxidase gene in strawberry fruit delays the fungus infection process. Plant Mol. Biol. Rep. 2016;34:592–606.10.1007/s11105-015-0946-y
- Wang J, Constabel CP. Polyphenol oxidase overexpression in transgenic Populus enhances resistance to herbivory by forest tent caterpillar (Malacosoma disstria). Planta. 2004;220:87–96.10.1007/s00425-004-1327-1
- Coetzer C, Corsini D, Love S, et al. Control of enzymatic browning in potato (Solanum tuberosum L.) by sense and antisense RNA from tomato polyphenol oxidase. J. Agric. Food Chem. 2001;49:652–657.10.1021/jf001217f
- Murata M, Haruta M, Murai N, et al. Transgenic apple (Malus × domestica) shoot showing low browning potential. J. Agric. Food Chem. 2000;48:5243–5248.10.1021/jf000771m
- Murata M, Nishimura M, Murai N, et al. A transgenic apple callus showing reduced polyphenol oxidase activity and lower browning potential. Biosci. Biotechnol. Biochem. 2001;65:383–388.10.1271/bbb.65.383
- Cheng GW, Crisosto CH. Browning potential, phenolic composition, and polyphenoloxidase activity of buffer extracts of peach and nectarine skin tissue. J. Am. Soc. Hort. Sci. 1995;120:835–838.
- Tomás-Barberán FA, Gil MA, Cremin P, et al. HPLC-DAD-ESIMS analysis of phenolic compounds in nectarines, peaches, and plums. J. Agric. Food Chem. 2001;49:4748–4760.10.1021/jf0104681
- Zhao X, Zhang W, Yin X, et al. Phenolic composition and antioxidant properties of different peach [Prunus persica (L.) Batsch] cultivars in China. Int. J. Mol. Sci. 2015;16:5762–5778.10.3390/ijms16035762
- Nakatani N, Kayano S, Kikuzaki H, et al. Identification, quantitative determination, and antioxidative activities of chlorogenic acid isomers in prune (Prunus domestica L.). J. Agric. Food Chem. 2000;48:5512–5516.10.1021/jf000422s
- Reig G, Iglesias I, Gatius F, et al. Antioxidant capacity, quality, and anthocyanin and nutrient contents of several peach cultivars [Prunus persica (L.) Batsch] grown in Spain. J. Agric. Food Chem. 2013;61:6344–6357.10.1021/jf401183d
- Carbonaro M, Mattera M, Nicoli S, et al. Modulation of antioxidant compounds in organic vs conventional fruit (peach, Prunus persica L., and pear, Pyrus communis L.). J. Agric. Food Chem. 2002;50:5458–5462.10.1021/jf0202584
- Singleton VL, Orthofer R, Lamuela-Raventos RM. Analysis of total phenols and other oxidation substrates and antioxidants by means of folin-ciocalteu reagent. Methods Enzymol. 1999;299:152–178.10.1016/S0076-6879(99)99017-1
- Mayer AM, Harel E, Ben-Shaul R. Assay of catechol oxidase—a critical comparison of methods. Phytochemistry. 1966;5:783–789.10.1016/S0031-9422(00)83660-2
- Nisha S, Revathi K, Chandrasekaran R, et al. Effect of plant compounds on induced activities of defense-related enzymes and pathogenesis related protein in bacterial blight disease susceptible rice plant. Physiol. Mol. Plant Pathol. 2012;80:1–9.10.1016/j.pmpp.2012.07.001
- Martinez MV, Whitaker JR. The biochemistry and control of enzymatic browning. Trends Food Sci. Technol. 1995;6:195–200.10.1016/S0924-2244(00)89054-8
- Amaki K, Saito E, Taniguchi K, et al. Role of chlorogenic acid quinone and interaction of chlorogenic acid quinone and catechins in the enzymatic browning of apple. Biosci. Biotechnol. Biochem. 2011;75:829–832.10.1271/bbb.100444
- Oszmianski J, Lee CY. Enzymic oxidative reaction of catechin and chlorogenic acid in a model system. J. Agric. Food Chem. 1990;38:1202–1204.10.1021/jf00095a009
- Werij JS, Kloosterman B, Celis-Gamboa C, et al. Unravelling enzymatic discoloration in potato through a combined approach of candidate genes, QTL, and expression analysis. Theor. Appl. Genet. 2007;115:245–252.10.1007/s00122-007-0560-y
- Chagné D, Krieger C, Rassam M, et al. QTL and candidate gene mapping for polyphenolic composition in apple fruit. BMC Plant Biol. 2012;12:12. doi: 10.1186/1471-2229-12-12
- Sun R, Li H. Mapping for quantitative trait loci and major genes associated with fresh-cut browning in apple. Hortscience. 2014;49:25–30.
- Niggeweg R, Michael AJ, Martin C. Engineering plants with increased levels of the antioxidant chlorogenic acid. Nat Biotechnol. 2004;22:746–754.10.1038/nbt966
- Hihara S, Tamura T. A new peach cultivar ‘Okayama PEH7 go’. Bull. Res. Inst. Agric. Okayama Pref. Tech. Cent. Agric, For Fish. 2014;5:7–11 [ in Japanese].
- Sasabe Y, Fujii Y, Kagami H, et al. A new peach cultivar ‘Hakurei’. Bull. Agric. Exp. Stn., Okayama Pref. Gen. Agric. Cent. 2001;19:25–27 [ in Japanese].
- Sasabe Y, Fujii Y, Kagami H, et al. A new peach cultivar ‘Okayama yume hakuto’. Bull. Agric. Exp. Stn., Okayama Pref. Gen. Agric. Cent. 2005;23:13–16 [ in Japanese].