Abstract
Tricholoma matsutake, a basidiomycete, forms ectomycorrhizas with Pinus densiflora as the host tree. Its fruiting body, “matsutake” in Japanese, is an edible and highly prized mushroom, and it grows in a circle called a fairy ring. Beneath the fairy ring of T. matsutake, a whitish mycelium-soil aggregated zone, called “shiro” in Japanese, develops. The front of the shiro, an active mycorrhizal zone, functions to gather nutrients from the soil and roots to nourish the fairy ring. Bacteria and sporulating fungi decrease from the shiro front, whereas they increase inside and outside the shiro front. Ohara demonstrated that the shiro front exhibited antimicrobial activity, but the antimicrobial substance has remained unidentified for 50 years. We have identified the antimicrobial substance as the (oxalato)aluminate complex, known as a reaction product of oxalic acid and aluminum phosphate to release soluble phosphorus. The complex protects the shiro from micro-organisms, and contributes to its development.
Graphical abstract
“Antimicrobial activity of the matsutake shiro” for left figure.“The antimicrobial substance, the (oxalato)aluminate complex, from the matsutake shiro” for right figure.
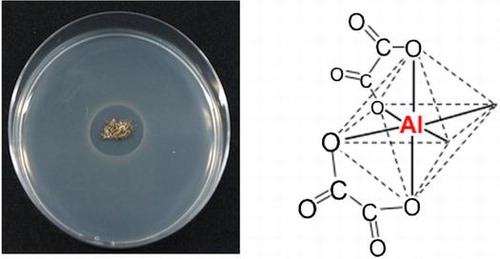
Mycorrhiza is a symbiotic organ consisting of plant roots and fungi.Citation1) The plant is supplied with nitrogen and mineral nutrients such as phosphorus, potassium, and water from fungi, and the fungi receive photosynthetic sugars such as glucose and fructose from the plant through mycorrhiza.Citation1,2) Tricholoma matsutake is an ectomycorrhizal fungus whose major host tree in Japan is the Japanese red pine, Pinus densiflora.Citation3) Its fruiting body, “matsutake” in Japanese, is a commercially valuable mushroom in Japan as well as truffle in Europe.Citation4) The artificial cultivation of T. matsutake has been attempted for a long time, but it has not yet succeeded.
T. matsutake forms a whitish mycelium-soil aggregated zone, called “shiro” in Japanese.Citation5) The shiro front corresponds to an active mycorrhizal zone forming a fairy ring of the fruiting body epigeously. The horizontal width of the shiro front is approximately 15 cm, its vertical depth ranges from 10 to 20 cm, and its front annually advances outwards at 10–15 cm. Bacteria and actinomycetes reportedly disappeared, and only Mortierella sp. (=Umbelopsis sp.) appeared in the shiro front, whereas they were abundant in the soil outside the shiro front and appeared inside the shiro front.Citation6) The elimination of micro-organisms from the shiro front was also observed in the shiro front at another site.Citation7) Ohara investigated the shiro in Iwakura, Kyoto, and demonstrated in 1966 that the active mycorrhizas and soil crusts from the shiro front inhibited the growth of Gram-positive and -negative bacteria from outside of the shiro, showing that an antimicrobial substance was present in the active mycorrhizas.Citation8) Antimicrobial activity was not found in the decayed zone of the shiro, newly developed roots with no mycorrhiza, the roots of red pine seedlings of two years old, a culture filtrate of Mortierella sp., and mycelia of T. matsutake.Citation8) This suggested that the antimicrobial activity was closely associated with the active mycorrhizas. The antimicrobial substance may protect the mycorrhizas from soil micro-organisms, and contribute to the preservation and development of the shiro.
Identification of the antimicrobial substance from the shiro front was attempted using Bacillus subtilis as a test bacterium that is also sensitive to the antimicrobial substance from the shiro front.Citation9) Volatile monoterpenes, α- and β-pinene, were proposed to be the antimicrobial compounds by analysis of the extract from the shiro front, and based on the antibacterial activity of the standard monoterpenes, although the antimicrobial substance was not isolated. We traced the antimicrobial activity of monoterpenes, α- and β-pinene, α-pinene oxide, (+)- and (−)-limonene, (+)-limonene oxide, and α-terpineol against B. subtilis using a paper disc method at a dose of 1 mg. However, we could not observe the antimicrobial activity of the monoterpenes except for (−)-limonene (not published). (−)-Limonene was not detected in the extract from the shiro,Citation9) and its low antimicrobial activity cannot account for the antimicrobial activity of the extract from the shiro front. These results strongly suggested that the active substance was not the monoterpenes. This paper clarifies the antimicrobial substance that has remained unidentified for 50 years, its distribution in the shiro, and its physiological and ecological functions.
Materials and Methods
Materials
The shiros at the Sakai Research Forest that have been conserved by Kyoto Prefectural Agriculture, Forestry and Fisheries Technology Center were used. The soils from the front of shiro No. 11, where fruiting bodies were 178 formed from 2009 to 2014, were collected in October 2012 and October 2013 for the detection of antimicrobial activity and isolation of the antimicrobial substance, respectively. The soils from shiro No. 32 and No. 7, where fruiting bodies were formed 15 and 48 from 2009 to 2014, respectively, were collected in October and November 2014, respectively. The soils were used for analysis of the contents of mycelium in T. matsutake, distribution of the (oxalato)aluminate complex, oxalic acid, antimicrobial activity, soluble phosphorus, densities of micro-organism, and pH. B. subtilis FKU101 was provided from Emeritus Professor K. Yamamoto, Kyoto University, and the other micro-organisms were purchased from RIKEN BioResource Center (Tsukuba, Japan). Rice (Oryza sativa L. cv. Nipponbare) seeds were provided from the Experimental Farm, Kyoto University, and lettuce (Lactuca sativa L. cv. Melbourne MT) seeds were purchased from a local supplier.
Chemicals
All chemicals were reagent grade. (Oxalato)aluminate hydrate as standard oxalato(aluminate) complex was purchased from Alfa Aesar (UK). Aluminum phosphate was purchased from Kanto Chemical (Japan). Yeast extract, tryptone, potato dextrose agar (PDA), and nutrient agar were purchased from Becton, Dickinson and Company (USA). Others were purchased from Wako Pure Chemical (Japan).
Antimicrobial assay
The antimicrobial activity against B. subtilis FKU101 was assayed at 30 °C for 24 h by the paper disc method.Citation10) All media were autoclaved for 15 min at 120 °C before use. B. subtilis was pre-incubated in a liquid medium consisted of 0.1% bonito extract, 0.1% yeast extract, and 0.05% NaCl overnight at 30 °C before the tests.
The pre-incubated media and an autoclaved new liquid media with 1.5% agar, were mixed at 1:19 to prepare agar media. The soil samples containing mycorrhizas at the front of shiro No.11 were directly placed on the agar media. Tested material was added into paper disc (diameter of 8 mm). The disc was placed on the agar media. Diameter of inhibitory zone was measured for evaluation of antimicrobial activity after incubation.
The agar media containing 5.5 mM aluminum phosphate were used for the antimicrobial assay of organic acids, which was added into a paper disc at 1 μmol. The agar media without aluminum phosphate were used as control. This assay was conducted in triplicate.
27Al-NMR measurement
AVANCE III 400 (104 MHz for 27Al) was used for the 27Al NMR measurement. AlCl3(H2O)6 was used as an external standard in the 27Al-NMR spectra. The ratio of individual speciation of the (oxalato)aluminate complex is calculated with the signal area. Distilled water was used as solvents.
Isolation of the antimicrobial substance
The soil (35 g) from the front of shiro No. 11 was extracted with 100 mL of 20% MeOH aqueous solution. The extract was filtered through a filter paper. The filtrate was concentrated to give a viscous liquid (353 mg), and the liquid was dissolved in a small amount of water. The solution was applied to an activated charcoal column (20 × 145 mm), and eluted with 100 mL of water and 10, 30, 60, and 100% MeOH. The material eluted with water was concentrated, and purified with a 32 × 380-mm column of TOYOPEARL DEAE-650 M (Tosoh, Japan) eluting with 600 mL of 0, 10, 30, 100, and 300 mM HCl aqueous solutions. The material (34 mg) eluted with 100 mM HCl was dried in vacuo at 50 °C for 3 h. The material was applied to an Oasis WAX column (150 mg/6 mL) that was preliminary washed with 10% NH4OH in MeOH (10 mL) and water (10 mL). The material dissolved in 10 mL water was applied on the column, and eluted with aqueous solution of 2% formic acid (10 mL), MeOH (15 mL), and 5 and 10% NH4OH in MeOH (15 mL). The material (21 mg) eluted with the 5% NH4OH in MeOH was concentrated, and dissolved in water for 27Al measurement. Slow evaporation of water yielded colorless needles, m.p. >300 °C. This was subjected to X-ray structural analysis.
X-ray structural analysis
Intensity data from the crystalline substance were obtained using a Rigaku Saturn 70 CCD diffractometer with a VariMax Mo Optic using Mo Kα radiation (λ = 0.71075 Å) (RIGAKU Corporation, Tokyo, Japan). Structures were solved by a direct method (SIR2004)Citation11) and refined by the full-matrix least squares method on F2 for all reflections (SHELXL-97).Citation12) All hydrogen atoms of H2O and H3O+ were placed at appropriate positions using DFIX instructions, while all other atoms were refined anisotropically. The crystalline [Al(C2O4)33−.·(H3O+)3(H2O)3]: M = 402.16, T = −170 °C, triclinic, P-1 (no.2), a = 7.8364(2) Å, b = 10.5104(5) Å, c = 10.5729(5) Å, α = 84.087(2)º, β = 70.4649(15)º, γ = 70.058(3)º, V = 771.43(6) Å3, Z = 2, Dcalc = 1.731 g cm−3, μ = 0.231 mm−1, 2θmax = 51.0º, measured/independent reflections = 11752/2837 (Rint = 0.0512), 271 refined parameters, GOF = 1.084, R1 = 0.0795 [I > 2σ(I)], wR2 = 0.2147 [for all data], largest diff. Peak and hole 1.003 and −0.909 e.Å−3 is contained in the supplementary crystallographic information for this paper. The data (CCDC-1432762) can be obtained free of charge from The Cambridge Crystallographic Data Centre via www.ccdc.cam.ac.uk/data_request/cif.
Effects of the coordination number on antimicrobial activity of the (oxalato)aluminate complex
AlCl3(H2O)6 and disodium oxalate were mixed at molar ratios of 1:1 for (oxalato)aluminate, 1:2 for bis(oxalato)aluminate, and 1:3 for tris(oxalato)aluminate in water.Citation13) Each solution was diluted with the liquid medium controlled at pH 5 to make test solutions whose concentration of Al was between 0 and 320 μM. The broth microdilution method was used to measure antimicrobial activity of the mixtures against B. subtilis.Citation14) The pre-incubated liquid medium was diluted with saline to 0.5 McFarland standard. The diluted medium was diluted to 1:10 with saline to prepare an inoculum medium. The inoculum medium and test solutions were put into 96-well microplate in the ratio of 1:19. A multi-length microplate reader (Multiskan JX, Thermo Fisher Scientific, USA) was used to measure the optical density of samples at 630 nm after incubation at 30 °C for 24 h. This test was conducted in triplicate.
Bacteriocidal activity of the (oxlato)aluminate complex
B. subtillis was pre-incubated in a liquid medium for 3 h at 30 °C. The incubated medium was divided equally. The (oxalato)aluminate complex was added to one of them to yield a concentration of 300 ppm. The pH of the other medium was adjusted to pH 6, which was the same as that of the 300 ppm broth. The two media were incubated for 2.5 h at 30 °C. The bacteria were washed with a new medium three times, and suspended in a new medium so that the optical density at 630 nm of the medium was 0.040. The suspensions were diluted 250 times by a new medium. The number of colonies was counted after incubation of 5 μL of the final suspensions on the agar plate for 18 h at 30 °C.
Effect of the (oxalato)aluminate complex on the growth of T matsutake
Blocks (5 mm × 5 mm), which cut from another colony of T. matsutake incubated for six months, were placed on 1/2 Hamada media, which consisted of 1% glucose, 0.1% yeast extract, 0.05% KH2PO4, and 1.5% agar, at pH 5 containing 30, 100, or 300 ppm of the (oxalato)aluminate complex.Citation3) After incubation at 23 °C for one month, the areas of colonies were measured by Image J (NIH, USA). This test was conducted in triplicate.
Seedling elongation test for rice
Seeds of rice were soaked in EtOH for 5 min, and washed three times with tap water.Citation15) The seeds were sterilized with 1% sodium hypochlorite for 1 h, and washed with running tap water for 3 h. The sterilized seeds were allowed to germinate in water for 2 days at 30 °C. Seven of the seedlings were placed in a glass tube containing 2 mL of a test solution, and grown in the tube capped with vinyl film for 1 week at 30 °C under continuous fluorescent light of 6000 lx. The length of the second leaf sheath was measured.
Lettuce germination assay
Fifty seeds of lettuce were placed on two filter papers (diameter of 55 mm) soaked in 3 mL of a test solution and allowed to germinate at 25 °C under consecutive 2500 lx for 48 h.Citation15) The germination ratio was defined as (number of germination seeds/50) × 100. This assay was conducted in triplicate.
Antimicrobial activity of the (oxalato)aluminate complex
The (oxalato)aluminate complex was added to the liquid media at pH 5 to yield a final concentration of 0, 30, 100, or 300 ppm, and added 1.5% agar to prepare agar media for antimicrobial assay. The 2 μL inoculum media of bacteria were inoculated directly on the agar plates. The inoculum medium was prepared with the same manner in “Effects of the coordination number on the activity of the (oxalato)aluminate complex”.
Spores obtained from fungi incubated for 6 days on plates of PDA were suspended in water. The suspensions were filtered through two layers of KimWipes S-200 (Nippon paper crecia, Japan), and centrifuged at 1100 × g for 1 min to collect spores. The spores were washed by saline and centrifuged. This washing was done three times. Spore concentration was adjusted to 0.5 McFarland standard with saline. The suspensions were diluted to 1:1000 with saline for inoculum suspensions.
The suspension of S. cerevisiae obtained from one-day-old PDA plate, whose concentration is 0.5 McFarland, was diluted to 1:1000 with saline for inoculum suspension. The inoculum suspensions of fungi and yeast were added 10 μL into paper discs. The discs were placed on the agar media, which were the same as the test for bacteria, for the assay.Citation14,16,17)
Analysis of the mycelium density
The soils of SI, SF, and SO from shiro No. 32 and 7 were lyophilized, pulverized with a Multi-beads Shocker (Yasui Kikai Corporation, Osaka, Japan), and their DNA content was extracted using CTAB lysis buffer containing 0.6% skim milk.Citation18,19) The DNA extracts were stored in TE buffer at -20 °C until use. qPCR analysis was performed using a LightCycler system (Roche Life Science, Mannheim, Germany), and the primer sets MY201f: 5′-GAGACACAACGGCGAGATT-3′/MY101r: 5′- ACCCTTACCCGCTCAGT-3′, which amplify, respectively, the 202-bp DNA fragment used to quantify T. matsutake mycelia. Plasmids for standard curve were constructed by inserting 202 bp of target DNA into the pT7Blue cloning vector (Novagen, Darmstadt, Germany).Citation20) DNA amplification and detection were performed in glass capillaries in a total volume of 20 μL containing 2 μL of LightCycler FastStart DNA Master SYBR Green I (Roche Diagnostics, Risch-Rotkreutz, Switzerland). The reaction mixture was composed of 3.2 μL (5 mM) of MgCl2, 1 μL (0.25 μM) of each primer, 10.8 μL of H2O (sterile PCR grade), and 2 μL of template DNA. To detect the signal derived from T. matsutake mycelia, the qPCR was performed using the following program as recommended by the manufacturer (Roche Life Science): 1 cycle at 95 °C for 10 min, followed by 50 cycles at 95 °C for 10 s, Ta for 2 s, 72 °C for 1 s, and 83 °C for 1 s, followed by signal detection. Unless stated otherwise, the standard curve was constructed using a 1.0-ng/μL sample of the respective plasmid containing the target DNA, which was serially diluted and added to the 20-μL reaction mixture. PCR products with a single melting curve that fitted the respective standard curve were considered to be authentic, quantified PCR products.
Analyses of the (oxalato)aluminate complex, antimicrobial activity, oxalic acid, and pH
Water (20 mL) was added to each soil (4 g) from shiro No. 32 and 7. After shaking for 30 min and filtration with filter paper, pHs of the filtrates were measured with a pH meter. The filtrates were lyophilized and dissolved in water. This solution was used for 27Al-NMR measurement to measure the content of the (oxalato)aluminate complex and antimicrobial assay with paper disc method. The solution was not concentrated for antimicrobial assay. The concentrations of the (oxalato)aluminate complex were calculated with a calibration curve between concentrations of 0.5 and 10 μM and the 27Al signal area of tris(oxalato)aluminate. The same filtrates were used for quantitation of oxalic acid with HPLC: Shodex RSpak KC-811 (300 × 8.0 mm, Showa Denko, Japan); 50 mM perchloric acid; 1.0 mL/min; 220 nm.
Densities of bacteria and fungi
Each soil (500 mg) from shiro No. 32 and 7 was passed through a sieve with a mesh size of 1 mm. The sieved soils were put into mortars and grinded. The grinded soils (100 mg) were transferred to glass tubes. Water (10 mL) containing 0.01% Tween 80 was added to the tubes. The tubes were shaken for 30 min. The supernatant (100 μL) was diluted to 1:10 by water, and spread on the nutrient agar media with 50 ppm iprodione for bacteria, and complete medium with 50 ppm kanamycin and 50 ppm cromycin for fungi. The complete medium was consisted of 1% glucose, 0.1% yeast extract, 0.1% tryptone, 0.15% Ca(NO3)2(H2O)4, 0.05% MgSO4(H2O)7, 0.05% KCl, 0.4% KH2PO4, 0.003% K2HPO4, and 1.5% agar. The number of colonies (CFU/g soil) were counted after incubation for 3 days at 23 °C. CFU meant “Colony Forming Unit”.
Quantification of phosphorus
Each soil (1 g) from shiro No. 32 and 7 was added to the 20 mL Bray 2 extractant consisted of 0.03 M NH4F and 0.1 M HCl.Citation21) The suspensions were shaken for 1 min and filtered through filter papers. The filtrates were analyzed by the ascorbic-molybdenum blue method with UV–vis spectrophotometer (UV-1800, Shimadzu, Japan).Citation22) Boric acid was used to eliminate possible interface from florides.Citation23)
Results
Isolation and identification of the antimicrobial substance
The soil containing mycorrhizas from the shiro front showed clear antibacterial activity against B. subtilis (Fig. (a)), confirming the results of Ohara.Citation8) The soil was extracted with ethyl acetate or a 20% MeOH aqueous solution. The extract with the 20% MeOH aqueous solution showed antibacterial activity, while the extract with ethyl acetate did not. The activity of the extract with the 20% MeOH aqueous solution was dose-dependent between 60 and 600 μg (Fig. (b)), and was not lost by heating at 100 °C for 60 min (data not shown). These properties of the antimicrobial substance showed that it was a water-soluble and heat-stable substance.
Fig. 1. The antimicrobial activity against B. subtilis. (a) The mycorrhiza from the shiro front, (b): Paper discs containing the extract from the shiro front.
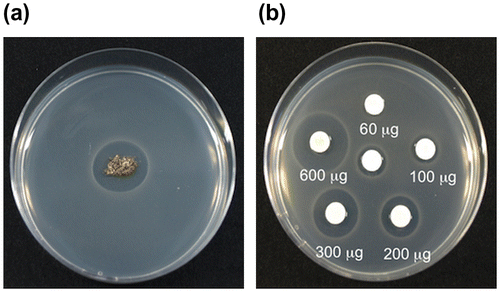
The extract with the 20% MeOH aqueous solution was applied to activated charcoal column. The antibacterial material eluted with water was purified with anion exchange resin of a DEAE type, and the antibacterial activity was detected in the material eluted with a 100 mM HCl aqueous solution. The material was further purified with another type of anion exchange resin, Oasis WAX, to yield an antibacterial fraction by elution with 5% NH4OH in MeOH. Crystallization of the material from the aqueous solution produced colorless needles that were identified as ammonium tris(oxalato)aluminate(III) trihydrate(III) by X-ray analysis (Fig. S1).Citation24) Ammonia was derived from the eluent, so the major substance from the shiro front would be tris(oxalato)aluminate(III) trihydrate(III). The 27Al NMR spectrum of the antibacterial material from the Oasis WAX column showed signals at 12.4 and 16.1 ppm at an intensity ratio 29:71 assignable to 27Al of bis(oxalato)aluminate and tris(oxalato)aluminate, respectively.Citation25) This indicated that only tris(oxalato)aluminate from the mixture of bis(oxalato)aluminate and tris(oxalato)aluminate crystallized. A standard (oxalato)aluminate complex that is a mixture of (oxalato)aluminate, bis(oxalato)aluminate, and tris(oxalato)aluminate at a ratio of 23:63:14 showed antimicrobial activity with the inhibitory zone diameter 20 mm at 320 μg. The extract from the No. 32 shiro front which contained 388 μg of the (oxalato)aluminate complex that had the three specifications ratio 22:71:7, as shown in Fig. , similar to that of the standard (oxalato)aluminate complex showed the inhibitory zone diameter 21 mm corresponding to that of the standard (oxalato)aluminate complex. Thus, the antimicrobial substance of the shiro front was identified as the (oxalato)aluminate complex.
The antimicrobial property of the (oxalato)aluminate complex
The minimum inhibitory dose of the (oxalato)aluminate complex was 0.1 μmol, while that of oxalic acid which had antimicrobial activity was 1 μmol (Table ).Citation26) Metal aluminum did not show antimicrobial activity.
Table 1. The antimicrobial activity of oxalic acid and the (oxalato)aluminate complex.
B. subtilis did not grow after washing the bacteria incubated in a medium containing the (oxalato)aluminate complex (data not shown). This result meant that the antimicrobial activity of the (oxalato)aluminate complex was bacteriocidal, and not bacteriostatic.
Aluminum adopts a regular octahedral complex where one to three molecules of oxalic acid can coordinate (Fig. ).Citation25) The number of oxalic acid molecules coordinating to aluminum depends on the molar ratio of aluminum to oxalic acid.Citation27) The antimicrobial activity could be different among one, two, and three molecule coordination. This possibility was examined using culture media containing mixtures of aluminum chloride and disodium oxalate with molar ratios of 1:1, 1:2, and 1:3, respectively. The ratio of (oxalato)aluminate, bis(oxalato)aluminate, and tris(oxalato)aluminate in the mixture with molar ratio of 1:1 was 80:20:0, that of 1:2 was 14:57:29, and that of 1:3 was 0:0:100. The activity of the molar ratio of 1:1 was the highest, and that of 1:2 was medium, while that of 1:3 was almost inactive (Fig. ). This result showed that the order of the antimicrobial activity was tris(oxalato)aluminate, bis(oxalato)aluminate, and (oxalato)aluminate from lowest to highest.
Fig. 3. The antimicrobial activities of the mixtures of aluminum chloride and disodium oxalate with molar ratios of 1:1 (○), 1:2 (●), and 1:3 (△).
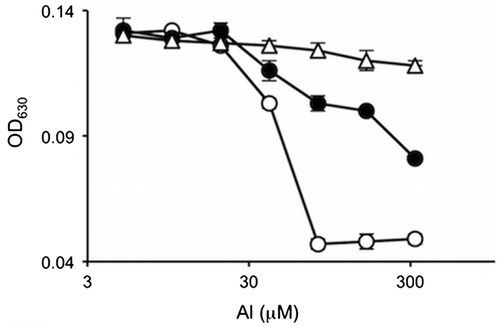
Aluminum of the (oxalato)aluminate complex would be derived from insoluble aluminum phosphate or aluminum hydroxide in soil. Oxalic acid secreted from the mycorrhizas probably reacts with aluminum phosphate or aluminum hydroxide to form a water-soluble (oxalato)aluminate complex. As a model of this process, antimicrobial activity of oxalic acid was tested using bacterial agar plates containing dispersed aluminum phosphate. Oxalic acid had a higher activity on agar plates containing aluminum phosphate than that on agar plates containing no aluminum phosphate (Fig. ), while aluminum phosphate itself did not have any activity.
Fig. 4. The antimicrobial activity of oxalic acid against B. subtilis in the absence (a) and presence (b) of aluminum phosphate (5.5 mM).
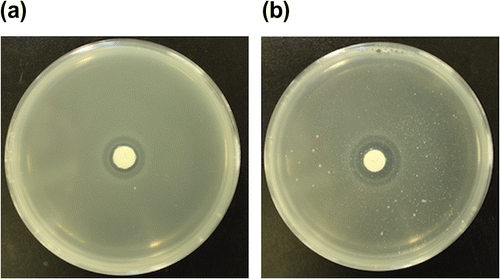
Aluminum may enhance the antimicrobial activity of other short chain organic acids. This possibility was examined with the same method as that for oxalic acid. Antimicrobial activity of lactic, malic, and succinic acid on bacterial agar plates was enhanced by impregnation of the media with aluminum phosphate, while that of malonic, and citric acid was not enhanced by it (Table ).
Table 2. The antimicrobial activity of organic acids (1 μmol) against B. subtilis in the presence or absence of aluminum phosphate (5.5 mM).
The occurrence of the (oxalato)aluminate complex in the shiro front suggested that T. matsutake was resistant to the (oxalato)aluminate complex. In fact, the effect of the (oxalato)aluminate complex at 30–300 ppm on the mycelium growth of T. matsutake was promotion rather than inhibition (Fig. , S2). The colony size of T. matsutake increased to 1.4 times on 1/2 Hamada medium containing 100 ppm of the (oxalato)aluminate complex on comparison with the control. The antimicrobial activity of the (oxalato)aluminate complex against other micro-organisms is summarized in Table . The antibacterial activity was not so high, but growth not only of Gram-positive but also of Gram-negative bacteria was inhibited at 300 ppm and pH 5. B. subtilis FKU101 used for the antimicrobial test in this experiment, and yeast were more sensitive to the (oxalato)aluminate complex than those bacteria. The growth of Penicillium citrinum and Aspergillus niger was partially inhibited at 300 ppm, and Trichoderma reesei and Rhizopus oryzae were resistant to the (oxalato)aluminate complex. The (oxalato)aluminate complex did not inhibit the growth of rice seedlings, or the germination of lettuce seeds up to 1000 ppm, indicating that it is not toxic to plants (data not shown).
Fig. 5. The effect of the (oxalato)aluminate complex on the mycelia growth of T. matsutake.
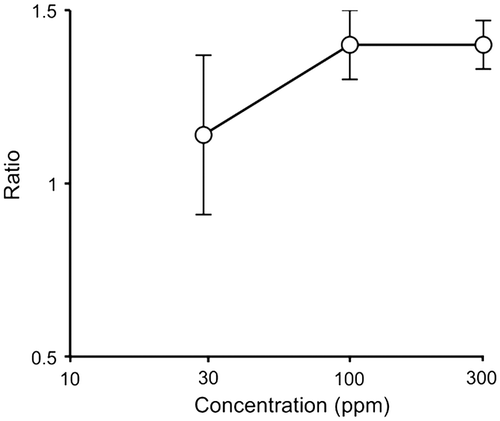
Table 3. The antimicrobial activity of the (oxalato)aluminate complex.
Distribution of the mycelium content of T. matsutake, the (oxalato)aluminate complex, oxalic acid, pH, antimicrobial activity, micro-organisms, and soluble phosphorus in shiros
If the (oxalato)aluminate complex is involved in protection of the shiro from soil micro-organisms, its distribution should correlate with that of the mycelia content of T. matsutake, and that of the antimicrobial activity of the shiro, while the distribution of bacteria and fungi should show a negative correlation with that of the (oxalato)aluminate complex. The pH of the shiro front should be lower than that outside and inside the shiro front since the shiro front would contain oxalic acid secreted from the active mycorrhizas. The contents of oxalic acid and soluble phosphorus of the shiro front should also be higher than those inside and outside the shiro front. To examine this expectation, two shiros No. 7 and No. 32 were chosen. Soil samples from 30 cm inside the shiro front, the shiro front, and 30 cm outside the shiro front at a depth of 15 cm were collected, and named as SI, SF, and SO, respectively, from shiro No. 32 in October, the season of fruiting body formation. Soil samples, SI, SF, and SO, were also collected from shiro No. 7 in November as well as shiro No. 32. The soil samples were taken from one spot in each zone, and analyzed once, since soil is highly heterogeneous and it is difficult to obtain statically significant average values from plural spots even in the same zone. We compared the result of the two shiros each other to know whether there is the same relationship among the items between the two shiros. The mycelium contents of SI and SF were 4.8 and 11.7 μg/g soil in No. 32, and 0 and 65 μg/g soil in No. 7, respectively (Fig. (a)). The mycelium was not detected in both SO. The results confirmed that SF was at the shiro front, and SI and SO were inside and outside the shiro front, respectively. The results of analyses are summarized in Fig. (b)–(h).
Fig. 6. The distribution of T. matsutake mycelium (a), the (oxalato)aluminate complex (b), oxalic acid (c), pH (d), antimicrobial activity (e), bacteria (f), fungi (g), and phosphorus (h) inside the shiro front (SI), at the shiro front (SF), and outside the shiro front (SO) of No.32 and 7.
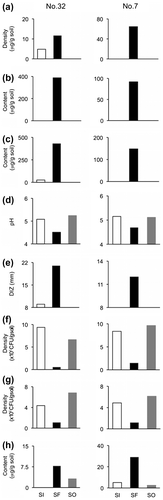
The contents and speciation of the (oxalato)aluminate complex in SI, SF, and SO were analyzed with the 27Al NMR spectra. The 27Al signals from the (oxalato)aluminate complex were detected only in SF, and the content SF was 388 μg/g soil in No. 32, and 92 μg/g soil in No. 7, respectively. The ratio of (oxalato)aluminate, bis(oxalato)aluminate, and tris(oxalato)aluminate were 22:71:7 for No. 32 and 31:65:4 for No. 7. The contents of oxalic acid in SI, SF, and SO from No. 32 were 26 μg/g soil, 433 μg/g soil, and less than the detection limit of 1.0 μg/g soil, respectively. Oxalic acid was detected at 150 μg/g soil in SF whereas not detected in SI, and SO from No. 7. The pHs of SI, SF, and SO in No. 32 were 5.0, 4.5, and 5.2, respectively, and in No. 7 were similar to those of No. 32.
For the antimicrobial activity of both shiros, SF showed high activity, SI low or no activity, and SO did not any activity. The densities of bacteria and fungi in SF were clearly lower than those of SI and SO. The distributions of antimicrobial activity and micro-organisms were closely related with those of the (oxalato)aluminate complex.
Oxalic acid secreted from the mycorrhizas can react with insoluble aluminum phosphate and/or insoluble calcium phosphate to release soluble phosphorus.Citation28) The quantitation of soluble phosphorus showed that the content of phosphorus in SF was higher than SI and SO.
Discussion
We have identified the antimicrobial substance of the shiro front that has remained unidentified 50 years as the (oxalato)aluminate complex. The (oxalato)aluminate complex is known as a byproduct of the reaction of oxalic acid and aluminum phosphate to release soluble phosphorus as an important mineral nutrient,Citation28) or as a byproduct formed through the detoxification of aluminum derived from aluminum phosphate and/or aluminum hydroxide in the acidic soil.Citation29) However, the antimicrobial activity of the (oxalato)aluminate complex has never been reported. This is the first report on its antimicrobial activity.
Aluminum appeared to enhance the antimicrobial activity of oxalic acid, since metal aluminum did not show antimicrobial activity (Table ). However, the inactivity of tris(oxalato)aluminate suggested that vacant coordination position of the (oxalato)aluminate complex was important for the antimicrobial activity, although the coordination of oxalic acid to aluminum is essential.
Oxalic acid secreted from the mycorrhizas may react with aluminum phosphate in soils to form the antimicrobial (oxalato)aluminate complex. The result of the model experiment (Fig. ) supported the above process.
Antimicrobial activity of lactic, malic, and succinic acid on bacterial agar plates was enhanced by impregnation of the media with aluminum phosphate, while that of malonic, and citric acid was not enhanced by it (Table ). This result showed that aluminum may enhance the antimicrobial activity of other short chain organic acids, and that not only a carboxyl group but also the chemical structure would be important for the antimicrobial activity. This may be coincident with the antimicrobial activity of the aluminum complex itself.
The effect of the (oxalato)aluminate complex on the mycelium growth of T. matsutake (Fig. , S2) suggested that the (oxalato)aluminate complex promoted the growth of T. matsutake under natural conditions. Antimicrobial activity of the complex may have a wider range against bacteria and yeast than against fungi (Table ), and the complex was not toxic to plants.
Distribution of the mycelium content of T. matsutake, the (oxalato)aluminate complex, and others are important to discuss the ecological function of the (oxalato)aluminate complex. As shown in Fig. (b), the 27Al signals from the (oxalato)aluminate complex were detected only in SF, and the antimicrobial complex, (oxalato)aluminate, and bis(oxalato)aluminate, were dominant. Oxalic acid was detected in SF of both shiros, and SI of No. 32 (Fig. (c)). The presence of oxalic acid in SI of No. 32 suggested that SI contained the (oxalato)aluminate complex, but its content would be too low to detect the 27Al signals. The pHs of SF in both shiros were lower than those of SI and SO (Fig. (d)), being similar to those reported previously.Citation30) The pH of the shiro is probably affected by the content of oxalic acid in the soil, since oxalic acid was detected only in SF in No. 7 and 32. Oxalic acid would be secreted from the mycorrhizas, and the producer is likely to be T. matsutake as well as wood-rotting basidomycetes,Citation31) but we cannot deny that P. densiflora produces oxalic acid. These results revealed that the shiro front contained a high level of the (oxalato)aluminate complex while the soils inside and outside of the shiro front contained a trace amount and none of the (oxalato)aluminate complex, respectively. When SI was the shiro front 1–2 years ago, it would have contained more of the (oxalato)aluminate complex than that of SI at present. The (oxalato)aluminate complex inside the shiro front may have been leached out by rain.
The distributions of antimicrobial activity and micro-organisms were closely related with those of the (oxalato)aluminate complex (Fig. (b), (e)–(g)). The low antimicrobial activity of SI from No. 32 was coincident with the suggested occurrence of a trace amount of the (oxalato)aluminate complex.
Oxalic acid secreted from the mycorrhizas can react with insoluble aluminum phosphate and/or insoluble calcium phosphate to release soluble phosphorus.Citation28) The content of phosphorus in SF was more than three times as high as that of SO (Fig. (h)). The low content of soluble phosphorus in SO suggested that most phosphorus in SO remained as the insoluble form of aluminum phosphate and/or calcium phosphate. The soluble phosphorus in SF may be utilized by T. matsutake as an important nutrient for formation of the fruiting body.
These results clearly showed a close correlation between the distribution of the (oxalato)aluminate complex and that of mycelium of T. matsutake, antimicrobial activity, and phosphorus. This correlation strongly suggests that the (oxalato)aluminate complex contributes to preservation and development of the shiro by protecting it from other micro-organisms, and through the supply of phosphorus. We propose a third role, acting as an antimicrobial agent, to the (oxalato)aluminate complex in addition to its roles as a byproduct formed by the release reaction of phosphorus, and as a byproduct of the detoxification of aluminum. For mycorrhiza, the secretion of oxalic acid is like “killing three birds with one stone” (Fig. ). The seasonal change in contents of the (oxalato)aluminate complex in the shiro, and identification and sensitivity to the (oxalato)aluminate complex of the micro-organisms isolated from SF, SI, and SO are under investigation.
Author contributions
N.H. conceived and designed the study, and initiated and coordinated collaborations. K.N., M.S., R.O., and K.O. carried out the antimicrobial assay and partially purified the active substance. K.N. carried out the antimicrobial assay, isolation, and identification of the active substance, the structure-activity analysis, and analyses of the (oxalato)aluminate complex, oxalic acid, pH, and phosphorus. T.F. and A.Y. conserved the shiro, and collected the shiro samples. T.S. and N.T. carried out X-ray experiments. C.T. analyzed the density of micro-organisms. M.Y. analyzed the density of the T. matsutake mycelium. S.H. measured the 27Al NMR spectra. K.N. and N.H. wrote the paper.
Disclosure statement
No potential conflict of interest was reported by the authors.
Funding
This work was partially supported by the JSPS Grants-in-Aid for Scientific Research [grant numbers 23380083, 16H04935].
Supplemental material
The supplemental material for this paper is available at http://dx.doi.org/10.1080/09168451.2016.1238298.
TBBB_1238298_Supplementary_Material.docx
Download MS Word (249.1 KB)Acknowledgments
We would like to thank Emeritus Professors of Kyoto University Koichi Koshimizu and Mitsuya Tsuda for giving us the opportunity to perform this research. We would like to thank Professor Shinya Funakawa and Dr. Tetsuhiro Watanabe of our Graduate School for the useful advice on soil analysis, and Professor Takefumi Hattori at Tokushima University and Professor Jian Feng Ma at Okayama University for valuable advice concerning the (oxalato)aluminum complex. We appreciate the cooperation of Dr. Masami Yoshikawa at Kyoto Prefectural Agriculture, Forestry and Fisheries Technology Center. We would also like to thank Emeritus Professor Kenji Yamamoto, Kyoto University for providing B. subtilis.
References
- Smith SE. Mycorrhizal fungi. Crit. Rev. Microbiol. 1974;3:275–313.10.3109/10408417409108753
- Donald HM. Ectomycorrhizae as biological deterrents to pathogenic root infections. Annu. Rev. Phytopathol. 1972;10:429–454.
- Ogawa M. Biology of matsutake mushroom. Tokyo: Tsukiji Shokan; 1978. (in Japanese).
- Hosford D, Pilz D, Molinia, R, et al. Ecology and management of the commercially harvested American matsutake mushrooms. Portland (OR): USDA Forest Service PNW-GTR-412; 1997. p. 1–68.
- Ogawa, M. Microbial ecology of mycorrhizal fungus, Tricholoma matsutake Ito et Imai (Sing.) in pine forest I. Fungal colony (‘Shiro’) of Tricholoma matsutake. Bull. Gov. Forest Exp. Sta. 1975;272:79–121.
- Ohara H, Hamada M. Disappearance of bacteria from the zone of active mycorrhizas in Tricholoma matsutake (S. Ito et Imai) singer. Nature. 1967;213:528–529.10.1038/213528a0
- Yoshimura, F. Competition and co-operation among soil micro-organisms. In: Horikoshi K, Futai K, editors. Soil microbial ecology. Tokyo: Asakura Publishing; 2003. p. 134–150. ( in Japanese)
- Ohara H. Antibacterial activity of mycorrhiza of Pinus densiflora formed by Tricholoma matsutake. Proc. Japan Acad. 1966;42:503–506.
- Tsuruta T, Kawai M. Studies on the artificial reproduction of Tricholoma matsutake (S. Ito et Imai) Sing. VII. Antibiotic activities of volatile substances extracted from a “Shiro” of T. matsutake. Trans. Mycol. Soc. Japan 1979;20:211–219. ( in Japanese)
- Beer EJ, Sherwood MB. The paper-disc agar-plate method for the assay of antibiotic substances. J. Bacteriol. 1945;50:459–467.
- Burla MC, Caliandro R, Camalli M, et al. SIR2004: an improved tool for crystal structure determination and refinement. J. Appl. Cryst. 2005;38:381–388.10.1107/S002188980403225X
- Sheldrick GM. A short history of SHELX. Acta Crystallogr. Sect. A. 2008;64:112–122.10.1107/S0108767307043930
- Ma JF, Hiradate S, Matsumoto H. High aluminium resistance in buckwheat. II. Oxalic acid detoxifies aluminum internally. Plant Physiol. 1998;117:753–759.10.1104/pp.117.3.753
- Cockerill FR, Hindler JA, Wikler MA, et al. M07-A9, Vol. 32, No. 2; Methods for dilution antimicrobial susceptibility tests for bacteria that grow aerobically; Approved Standard -9th ed. Wayne (PA): Clinical and Laboratory Standards Institute; 2012.
- Todoroki Y, Hirai N, Koshimizu K. 8′- and 9′-Methoxyabscisic acids as antimetabolic analogs of abscisic acid. Biosci. Biotech. Biochem. 1994;58:707–715.10.1271/bbb.58.707
- Dota KFD, Freitas AR, Cosolaro MEL, et al. A challenge for clinical laboratories: detection of antifungal resistance in Candida species causing vulvovaginal candidiasis. Labmedicine. 2011;42:87–93.
- Zhang J, Chen J, Huang HQ, et al. Comparison of a glucose consumption based method with the CLSI M38-A method for testing antifungal susceptibility of Trichophyton rubrum and Trichophyton mentagrophytes. Chin. Med. J. 2010;123:1909–1914.
- Yamaguchi M, Nakamura M, Takano M, et al. Quantification of the mycelial mass of the white-rot fungus. Pleurotus pulmonarius by real-time PCR. Bull. FFPRI 2009;8:133–141.
- Ugawa S, Yamaguchi M, Miura S, et al. A method for obtaining the relationship between the amount of DNA and the fine root weight from mixtures of fine roots and soil particles. Soil Sci. Plant Nutr. 2012;58:510–516.10.1080/00380768.2012.700462
- Yamaguchi M, Narimatsu M, Fujita T, et al. A qPCR assay that specifically quantifies Tricholoma matsutake biomass in natural soil. Mycorrhiza. Forthcoming 2016.
- Bray RH, Kurtz LT. Determination of total, organic, and available forms of phosphorus in soils. Soil Sci. 1945;59:39–46.10.1097/00010694-194501000-00006
- Murphy J, Riley JP. A modified single solution method for the determination of phosphate in natural waters. Anal. Chim. Acta. 1962;27:31–36.10.1016/S0003-2670(00)88444-5
- Kurtz LT. Elimination of fluoride interference in the molybdenum blue reaction. Ind. Eng. Chem. Anal. Ed. 1942;14:855.10.1021/i560111a008
- Bulc N, Golic L, Siftar J. Structure of ammonium tris(oxalato)gallate(III) trihydrate, (I) (NH4)3[Ga(C2O4)3].3H2O, and ammonium tris(oxalato)aluminate(III) trihydrate, (II) (NH4)3[Al(C2O4)3].3H2O. Acta Cryst. 1984;C40:1829–1831.
- Kerven GL, Larsen PL, Bell LC, et al. Quantitative 27Al NMR spectroscopic studies of Al(III) complexes with organic acid ligands and their comparison with GEOCHEM predicted values. Plant Soil. 1995;171:35–39.10.1007/BF00009561
- Yamamoto Y, Higashi K, Yoshii H. Inhibitory activity of organic acids on food spoilage bacteria. Nippon Shokuhin Kogyo Gakkaishi. 1984;31:525–530.10.3136/nskkk1962.31.8_525
- Ma JF, Hiradate S, Matsumoto H. High aluminium resistance in buckwheat. II. Oxalic acid detoxifies aluminum internally. Plant Physiol. 1998;117:753–759.10.1104/pp.117.3.753
- Graustein WC, Cromack K Jr, Sollins P. Calcium oxalate: occurrence in soils and effect on nutrient and geochemical cycles. Science. 1977;198:1252–1254.10.1126/science.198.4323.1252
- Zheng SJ, Ma JF, Matsumoto H. High aluminium resistance in buckwheat. I. Al-induced specific secretion of oxalic acid from root tips. Plant Physiol. 1998;117:745–751.10.1104/pp.117.3.745
- Islam F, Ohga S. The response of fruit body formation on Tricholoma matsutake in situ condition by applying electric pulse stimulator. Int. Sch. Res. Notices Agron. 2012;2012. Article ID 462724. Available from: http://dx.doi.org/10.5402/2012/462724
- Shimada M, Akamtsu Y, Tokimatsu T, et al. Possible biochemical roles of oxalic acid as a low molecular weight compound involved in brown-rot and white-rod wood decays. J. Biotechnol. 1997;53:103–113.10.1016/S0168-1656(97)01679-9