Abstract
Arabinogalactan-proteins (AGPs) are highly diverse plant proteoglycans found on the plant cell surface. AGPs have large arabinogalactan (AG) moieties attached to a core-protein rich in hydroxyproline (Hyp). The AG undergoes hydrolysis by various glycoside hydrolases, most of which have been identified, whereas the core-proteins is presumably degraded by unknown proteases/peptidases secreted from fungi and bacteria in nature. Although several enzymes hydrolyzing other Hyp-rich proteins are known, the enzymes acting on the core-proteins of AGPs remain to be identified. The present study describes the detection of protease/peptidase activity toward AGP core-proteins in the culture medium of winter mushroom (Flammulina velutipes) and partial purification of the enzyme by several conventional chromatography steps. The enzyme showed higher activity toward Hyp residues than toward proline and alanine residues and acted on core-proteins prepared from gum arabic. Since the activity was inhibited in the presence of Pefabloc SC, the enzyme is probably a serine protease.
Graphical Abstract
The degradation of the core-protein of AGPs by a protease/peptidase from winter mushroom.
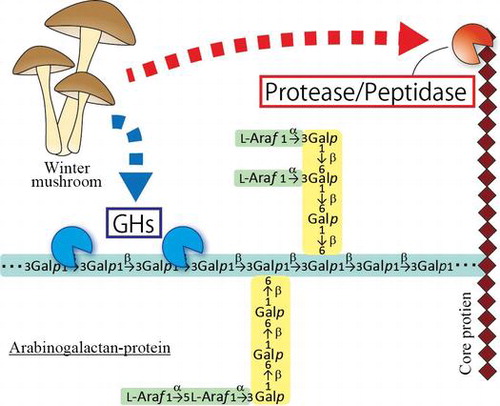
Proteoglycans are glycoproteins with particularly large sugar moieties. Arabinogalactan-proteins (AGPs) are plant proteoglycans present on plasma membranes and in cell walls. AGPs have large arabinogalactan (AG) moieties attached to a core-protein that is rich in hydroxyproline (Hyp), alanine (Ala), serine (Ser), and threonine (Thr)Citation1,2). It has been proposed that the AG chains are attached to the Hyp residues of the core-protein, in particular, Ala-Hyp, Ser-Hyp, and Thr-Hyp.Citation3) AG comprises a β-1,3-galactan main chain and β-1,6-galactan side chains, to which various monosaccharides such as L-arabinose, 4-O-methyl-glucuronic acid, L-fucose and L-rhamnose are attached.Citation4–7) AGPs are implicated in various physiological events including cell differentiation, pollen tube growth, and stress resistance.Citation8,9) This is, for instance, indicated by experiments with β-glycosyl Yariv reagent, which selectively binds to the β-1,3-galactan main chains of AG moieties, and disturbs the differentiation of tracheary elements in Zinnia elegans.Citation10,11) Recently, the terminal structure of AG which includes 4-O-methyl-glucuronosyl-β-1,6-galactosyl sequences, called AMOR, has apparently been shown to afford pollen tubes the capacity to respond to an ovular attractant peptide called LURE.Citation12)
The AG moieties of these glycoproteins are hydrolyzed by various glycoside hydrolases. In plants, AGs undergo degradation by exo-type enzymes such as β-galactosidase, α-L-arabinofuranosidase, and β-glucuronidase.Citation13–15) AGs are also degraded by galactanases and various exo-type enzymes secreted by bacteria and fungi. Endo-β-1,6-galactanase (EC 3.2.1.164) isolated from Trichoderma viride specifically acts on β-1,6-galactan side chains.Citation16) Exo-β-1,3-galactanase (EC 3.2.1.145) and endo-β-1,3-galactanase (EC 3.2.1.181) isolated from Irpex lacteus and Flammulina velutipes, respectively, employ different modes of action to hydrolyze β-1,3-galactan main chains.Citation17,18) These specific enzymes can be used as meet tools to analyze the structure and molecular function of AGPs.Citation3–6) However, no protease/peptidase acting on the core-proteins of AGPs has been discovered so far.
Several proteases/peptidases acting on non-AGP Hyp-rich proteins are known. Collagenases specifically hydrolyze collagens in which they recognize Gly-Hyp-X motifs, whereas other proteases cannot degrade collagen molecules because of the rigid, Hyp residues including, triple helix structure of the substrate.Citation19) Extensins are Hyp-rich plant glycoproteins containing Ser-Hyp-Hyp-Hyp-Hyp repeat units.Citation20) Two enzymes acting on these proteins have been reported. A KDEL-tailed cysteine endopeptidase, CysEP, liberates peptides from extensin prepared from tobacco BY-2 cell suspension cultures by heterologous expression.Citation21) As CysEP does not degrade synthetic peptides in which extensin motifs are replaced with AGP motifs, CysEP does not act on the core-proteins of AGPs. A metalloprotease partially purified from Xanthomonas campestris also has shown activity to degrade extensin prepared from a tomato suspension culture. However, the enzyme has neither been isolated nor characterized.Citation22) In addition, several peptidases specific to proline (Pro) and Hyp residues have been found in animals, fungi, and plants. These peptidases can be categorized into three groups, prolyl aminopeptidase (PAP, EC 3.4.115) acting on Pro and Hyp residues of the amino terminus of proteins, prolyl oligopeptidase (POP, EC 3.4.21.26) acting on the carboxyl side of Pro residues in an endo manner, and dipeptidyl aminopeptidase IV (DDPIV, EC3.4.14.5) liberating X-Pro dipeptides from amino termini.Citation23) Although there are a great many types of proteases/peptidases, it is still unknown which proteases/peptidases degrade the core-protein of AGPs.
For this study, we tried to find an AGP-active protease/peptidase in the culture medium of winter mushroom F. velutipes, which is known to digest gum arabic, a commercial AGP prepared from the acacia (Acacia senegal) tree. Using core-proteins prepared from gum arabic by chemical and enzymatic treatments as the substrate, we detected hydrolytic activity in the culture medium and partially purified the enzyme by conventional chromatography. The properties of the enzyme, including substrate specificity and sensitivity to inhibitors, were analyzed.
Materials and methods
Materials
F. velutipes (NBRC7663) was obtained from the NITE Biological Resource Center (Kisarazu, Japan). The recombinant α-L-arabinofuranosidase from Neurospora crassa (rNcAraf1),Citation24) recombinant β-glucuronidase from Aspergillus niger (rAnGlcAase),Citation25) recombinant endo-β-1,6-galactanase from Trichoderma viride (rTv6GAL),Citation26) recombinant exo-β-1,3-galactanase from Irpex lacteus (rIl3GAL),Citation27) recombinant endo-β-1,3-galactanase from F. velutipes (rFvEn3GAL),Citation18) and recombinant β-L-arabinopyranosidase from Streptomyces avermitilis (rArapase)Citation28) were prepared as described previously. Gum Arabic, L-alanine β-naphthylamide (Ala-β-NA), L-proline β-naphthylamide (Pro- β-NA), and trans-4-hydroxy-L-proline β-naphthylamide (Hyp- β-NA) were purchased from Sigma (St. Louis, MO, USA). The Gel Filtration Standard was from Bio-Rad (Hercules, CA, USA). The Protease Inhibitors Set including antipain, bestatin, chymostatin E-64, leupeptin, pestatin, phosphoramidon, Pefabloc SC, EDTA-Na2, and aprotinin was from Roche (Basel, Switzerland). Phenylmethylsulfonyl fluoride (PMSF) was from Wako (Osaka, Japan). N-ethylmaleimide was from Tokyo Chemical Industry Co. (Tokyo, Japan).
Culture of F. velutipes
Mycelia of F. velutipes (NBRC7663) were aerobically cultured in liquid medium containing 2% (w/v) gum arabic as the carbon source, 0.6% (w/v) Tryptone, 0.3% (w/v) yeast extract, 1% (w/v) potassium phosphate, and 0.05% (w/v) magnesium sulfate at 28 °C with shaking at 100 rpm for 21 days.
Preparation of AGP core-proteins
Core-protein fractions of AGP were prepared from gum arabic by Smith degradation followed by enzymatic degradation to remove the carbohydrate moieties. Details of the procedure have been described previously.Citation29) The Smith degradation products were digested with rNcAraf1, rArapase, rAnGlcAase, rTv6GAL, rIl3GAL, and rFvEn3GAL in 50 mM acetate buffer (pH 5.0) at 37 °C for 24 h. To separate the core-protein from mono- and oligosaccharides, the degradation products were applied to a Sepharose 6B column (4.2 × 100 cm; GE Healthcare, Tokyo, Japan). The concentration of the sugar and the core-protein in the eluted fractions was determined by the phenol-sulfuric acid methodCitation30) and the alkaline hydrolysis methodCitation31) using galactose (Gal) and bovine serum albumin (BSA) as standards, respectively.
Enzyme assay
Activity toward Ala- β-NA, Pro- β-NA, and Hyp- β-NA was determined by measuring the amount of β-naphthylamine using the diazotization and coupling method.Citation32) The reaction was performed at 37 °C using a mixture (150 μL) containing the enzyme, 50 mM Tris-HCl buffer (pH 7.0), and 1 mM substrate. Fifty μL of 20% perchloric acid was added to terminate the reaction and the mixture was kept at 4 °C for 15 min. For diazotization of β-naphthylamine liberated from the substrates, 200 μL of pre-cooled 0.2% sodium nitrite was added and the mixture was kept at 4 °C for 10 min. To remove excess nitrite, 200 μL of 0.5% ammonium sulfamate was added, and then 500 μL of 0.1% N-(1-naphthyl)ethylenediamine dihydrochloride (methanol solution) was added as chromogen. The mixture was incubated at 37 °C for 30 min to generate azo dye and centrifuged at 15,000 rpm for 5 min, and the supernatant was collected. The absorbance of the azo dye at 578 nm was measured using β-naphthylamine as the standard. One unit of enzyme activity releases 1 μmol of β-naphthylamine from substrate per min. The activity toward core-protein of AGP was determined by the ninhydrin method using Ala as the standard.Citation33) In this assay, the reaction was performed at 37 °C using a mixture (200 μL) containing the enzyme, 50 mM Tris-HCl buffer (pH 7.0), and 50 μg/mL of core-protein fraction. After the reaction was terminated by boiling, 100 μL of 0.2 M citrate buffer (pH 5.0), 200 μL of 0.2 mM potassium cyanide (2-methoxyethanol solution), and 40 μL of 5% ninhydrin (2-methoxyethanol solution) were added and mixed well. The mixture was heated at 100 °C for 15 min, and 500 μL of 60% ethanol was added. The absorbance of the solution at 570 nm was measured. One unit of enzyme activity is capable of producing 1 μmol of amino acids per min. In the inhibitors assay, each inhibitor in the Protease Inhibitors Set was used at the concentration recommended by the supplier.
Purification of enzyme
Native enzyme was purified from the culture medium of F. velutipes mycelia. The culture medium was filtered through nylon mesh to remove cell debris, and collected as a crude enzyme fraction. The crude enzyme was brought to 80% saturation with (NH4)2SO4, left for 30 min, and centrifuged. The pellet was dissolved in a small volume of 10 mM Tris-HCl buffer (pH 7.0). In order to lower the salt concentration, the enzyme solution was diluted to one-fifth concentration with 10 mM Tris-HCl buffer (pH 8.0), and applied to a diethylaminoethyl (DEAE)-Sepharose Fast Flow (F. F.) column (3.6 × 40 cm, GE Healthcare) that had been equilibrated with 10 mM Tris-HCl buffer (pH 8.0). The column was washed with the buffer, and eluted with a linear gradient of 0-0.5 M NaCl in the same buffer. The active fractions were collected, and absorbed onto a Bio-Gel HTP (hydroxyapatite) column (2.8 × 27 cm, Bio-Rad) equilibrated with 10 mM phosphate buffer (pH 7.2). After washing the column with the buffer, the column was eluted with a linear gradient of 10-400 mM of the phosphate buffer. The active fractions were collected, and diluted to five-fold volume with 10 mM Tris-HCl buffer (pH 8.0). The enzyme fraction was concentrated using a small Q-Sepharose F. F. column (1.6 × 4.5 cm, GE Healthcare). Bound proteins were eluted with a linear gradient of 0-0.5 M NaCl in the buffer. The enzyme fraction was then subjected to gel-permeation chromatography on a Sephacryl S-200 column (2.1 × 51 cm, GE Healthcare) equilibrated with 10 mM Tris-HCl buffer (pH 7.0). The active fractions were collected and an equal volume of 3.4 M (NH4)2SO4 was added. The enzyme fraction was applied to a Phenyl-Sepharose CL-4B column (0.5 × 8.6 cm, GE Healthcare) equilibrated with 10 mM Tris-HCl buffer (pH 7.0) containing 1.7 M (NH4)2SO4. The column was washed, and eluted with a linear gradient of 1.7-0 M (NH4)2SO4 in the buffer. The fractions were collected and used for analysis of enzyme properties. The purity of the enzyme was determined on SDS-polyacrylamide gel electrophoresis (PAGE).Citation34) Proteins in the gel were stained with Coomassie Brilliant Blue R-250. The concentration of protein was determined by the method of BradfordCitation35) using BSA as the standard.
Results
Hydrolytic activity toward the core-proteins of AGPs and enzyme purification
For the present study, we sought to identify a protease/peptidase in the culture medium of F. velutipes acting on the core-proteins of AGP. The substrate, AGP core-proteins, was prepared from gum arabic by Smith degradation followed by enzyme treatment to remove the carbohydrate moieties. The core-proteins were subjected to gel-permeation chromatography on a Sepharose 6B column (Supplemental Information, Sup. Fig. S1) and fractionated into three fractions, designated as high-, middle-, and low-Mr. When AGPs are classified on the basis of their core-protein, there are many different types. For example, the so-called AG peptides have short sequences as their core-protein but fasciclin-like AGPs consist of more than 250 amino acids and contain a large fasciclin domain.Citation36,37) The three fractions we obtained from gum arabic may thus derive from different types of core-proteins. We note that most of the carbohydrate moieties of gum arabic appear to have been removed, as almost no sugar was detected in these fractions.
The culture medium of F. velutipes exhibited protease/peptidase activity toward the high-Mr core-protein fraction (Fig. ). Since the amino acids detected by ninhydrin increased in the time-course reaction and the activity was lost by heat treatment, we concluded that the culture medium of F. velutipes contained a protease/peptidase acting on the core-protein of the AGP.
Fig. 1. Protease/peptidase activity toward core-proteins of AGP in the culture medium of F. velutipes.
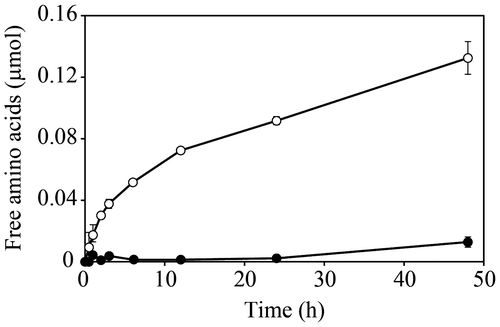
The protease/peptidase was purified from the culture medium of F. velutipes using Hyp-β-NA as the substrate because we assumed that the enzyme would prefer Hyp residues, which occur in great numbers in the core-proteins of AGPs.Citation2) The enzyme was first purified by ammonium sulfate fractionation, subsequently purified by five conventional chromatographies on a DEAE-Sepharose F. F. column, hydroxyapatite, Q-Sepharose F. F. (anion-exchange), Sephacryl S-200 (gel-permeation), and Phenyl-Sepharose CL-4B (hydrophobic) (Sup. Fig. S2). In the gel-permeation chromatography, the enzyme was eluted in fractions between relative molecular masses 44 and 17 kDa on a Sephacryl S-200 column (Sup. Fig. S2 D). The enzyme was finally purified 8682-fold in a yield of 0.7% (Table ). The purified enzyme was subjected to SDS-PAGE, and appeared as two bands with apparent molecular masses of 60 and 35 kDa (Fig. ), indicating that the purification was only partial.
Table 1. Purification of a protease/peptidase from F. velutipes acting on AGP core-proteins.
Properties of the enzyme
The maximum activity of the partially purified enzyme toward Hyp-β-NA was observed at pH 6.0–8.0 (Fig. (A)). The enzyme was stable in a broad pH range from 6.0 to 9.0 and lost most of its activity below pH 4.5 (Fig. (B)). Although the optimum temperature for the activity was 60 °C (Fig. (C)), the enzyme was not stable at 45 °C or higher temperatures for 12 h (Fig. (D)). The effects of several metal ions on the activity were examined (Table ). Ca2+ and Mn2+ slightly increased the activity, but obvious activation was not observed.
Fig. 3. Effects of pH and temperature on the activity of the protease/peptidase.
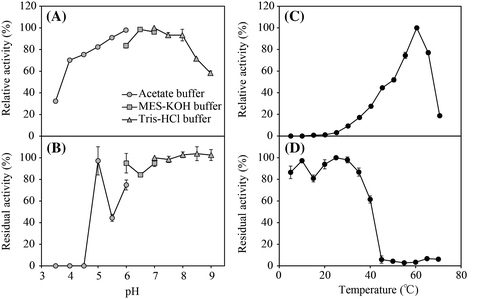
Table 2. Effect of metal ions and inhibitors on the activity of the protease/peptidase.
Effect of protease inhibitors
Proteases can be classified on the basis of their catalytic residues and thus show different sensitivity to specific inhibitors. To obtain a clue as to the type of the protease we had found, we treated the purified enzyme with various protease inhibitors (Table ). PMSF and Pefabloc SC partially inhibited the activity toward Hyp-β-NA, whereas E-64, phosphoramidon and ethylenediaminetetraacetic acid (EDTA)-Na2 did not have any effect. These observations suggest that the purified enzyme is a type of serine protease but not a cysteine protease, aspartic protease, or metalloprotease.
Substrate specificity of the enzyme
To determine the substrate specificity of the partially purified enzyme toward amino acid residues, the activity toward the synthetic β-NA substrates, Hyp-β-NA, Pro-β-NA, and Ala-β-NA, was compared (Table ). Hyp-β-NA was a better substrate for the purified enzyme than Pro-β-NA and Ala-β-NA, suggesting that the enzyme prefers Hyp residues to other amino acids in its substrates. The substrate specificity toward the core-protein fractions was also determined (Table ). The high-Mr fraction of core-protein was the best substrate among the protein substrates tested here. Native gum arabic with the carbohydrate moieties still attached was a poor substrate for the enzyme, suggesting that large numbers of AG chains affect the access of the enzyme to the core-proteins.
Table 3. Substrate specificity of the enzyme.
Discussion
Fungi and bacteria secrete various glycoside hydrolases to degrade the carbohydrate moieties of AGPs. Although glycoside hydrolases acting on the carbohydrate moieties of AGPs, such as exo-β-1,3-galactanase and endo-β-1,6-galactanase, have been isolated, no protease/peptidase acting on the core-proteins of AGPs has so far been found. In the present study, protease activity hydrolyzing the core-protein fraction prepared from gum arabic was detected in the culture medium of F. velutipes. Since AGPs including gum arabic from A. senegal have core-proteins rich in Hyp residues,Citation2) the activity of the enzyme was assayed using Hyp-β-NA in the purification by conventional chromatography and for the characterization of the enzyme.
Although the enzyme was significantly purified by conventional chromatography, the purified enzyme fraction still contained at least two proteins with apparent molecular masses of 60 and 35 kDa (Fig. ), indicating that the purification was not quite complete. On the basis of the elution position of the activity on gel-permeation chromatography (Sup. Fig. S2 D), the smaller protein is presumed to be the enzyme, although further purification is necessary to identify it with certainty. Addition of calcium and manganese ions slightly increased activity (Table ). We note that collagenases require zinc ion for their activity and calcium ion for enzyme stability.Citation38,39) It is possible that the presence of metal ions such as calcium ion increases the stability of the enzyme.
The activity of the partially purified enzyme was inhibited by the serine protease-specific inhibitors Pefabloc SC and PMSF. To date, an enormous number of proteases and peptidases have been reported. Some serine proteases among them act on Pro residues of proteins and these are classified into three types, prolyl oligopeptidase (POP), dipeptidyl aminopeptidase IV (DPPIV), and prolyl aminopeptidase (PAP).Citation23,40,41) According to the MEROPS database (http://merops.sanger.ac.uk), POP and DPPIV belong to the S9 family, whereas PAP belongs to the S33 family containing mainly exopeptidases. The properties of the enzyme purified in the present study made it appear similar to the enzymes in the S9 family. However, the proteins in the purified enzyme fraction were detected as bands with Mr of 60 and 35 kDa, setting them apart from the known S9 family enzymes with the β-propeller domain, which are heavier (~80 kDa).Citation42,43)
The purified enzyme hydrolyzed the artificial substrates, Hyp-β-NA, Pro-β-NA, and Ala-β-NA, suggesting that it is acting in an exo-manner. On the other hand, the enzyme also degraded the core-proteins of AGPs, and preferred the high-Mr fraction of the core-proteins to middle- and low-Mr fractions (Table ). It has been reported that several endo-peptidases act on artificial substrates releasing monomeric amino acids.Citation40,41) Therefore, we cannot exclude the possibility that the purified enzyme hydrolyzes the core-protein in an endo-manner.
The enzyme showed rather low activity toward native gum arabic compared with the core-protein fractions. This suggests that AG chains attached to the core-proteins interfere with the action of the enzyme, possibly because of steric hindrance. It also suggests that the advance degradation of the carbohydrate moieties by glycoside hydrolases is important for the effective degradation of the core-protein by the enzyme in nature.
Since the rate of turnover of AGPs is very rapid,Citation44) it seems conceivable that an enzyme degrading the core-proteins of AGPs also exists in plants. Cleland et al. (1967)Citation45) demonstrated that extracellular free Hyp was incorporated into a protein fraction after being converted to Pro in Avena sativa. This suggests the presence of a system to degrade Hyp-rich proteins, such as AGP and extensin, and recycle Hyp. The enzyme CysEP, which hydrolyses extensin, another cell wall glycoprotein rich in Hyp, is widely distributed in land plants and has been found in Arabidopsis thaliana.Citation21) However, the enzyme recognizes an extensin motif, Ser-Hyp-Hyp-Hyp-Hyp, that is absent or rare in AGP core-proteins. Besides CysEP, proteases recognizing Pro residues of proteins have been identified in plants, for example, a proline iminopeptidase (S33 family) from cabbage (Brassica oleraceae) and a prolyl endopeptidase (S9 family) from carrot (Daucus carota).Citation46,47) At this point, 321 and 416 genes encoding serine proteases have been found in the genomes of A. thaliana and Populus trichocarpa, respectively.Citation48) No serine protease with activity toward the core-proteins of AGPs has as yet been identified, but there may well be such among these presumptive enzymes in A. thaliana and P. trichocarpa. Although we have determined some properties of the protease/peptidase extracted from the culture medium of F. velutipes, it is necessary to determine the amino acid sequence of a purified specimen to identify the enzyme. Further studies on this enzyme should give new insights into the mechanisms that accomplish the degradation of AGPs.
Author contribution
Yoshihisa Yoshimi, Yoichi Tsumuraya, and Toshihisa Kotake designed the experiments. Yoshihisa Yoshimi, Yumi Sugawara, and Satoshi Kaneko performed the purification of the enzyme. Yoshihisa Yoshimi, Chiaki Hori, and Kiyohiko Igarashi characterized the enzyme. Yoshihisa Yoshimi, Yoichi Tsumuraya, and Toshihisa Kotake wrote the paper.
Funding
This work was supported in part by a grant-in-aid for JSPS fellows to Y. Yoshimi. [Grant-in-Aid for JSPS Fellows no. 16J05924] from the Japan Society for the Promotion of Science; Ministry of Education, Culture, Sports, Science, and Technology [grant number 24114006].
Disclosure statement
No potential conflict of interest was reported by the authors.
Supplemental material
The supplemental material for this paper is available at http://dx.doi.org/10.1080/09168451.2016.1258985.
TBBB_1258985_Supplemental_Data.zip
Download Zip (4.2 MB)Notes
Abbreviations: AG, arabinogalactan; AGP, arabinogalactan-protein; BSA, bovine serum albumin; DEAE, diethylaminoethyl; DPP-IV, dipeptidyl peptidase IV; EDTA, ethylenediaminetetraacetic acid; Hyp, hydroxyproline; β-NA, β-naphthylamide; PAP, prolyl aminopeptidase; PMSF, Phenylmethylsulfonyl fluoride; POP, prolyl oligopeptidase; rAnGlcAase, recombinant β-glucuronidase from Aspergillus niger; rArapase, recombinant β-L-arabinopyranosidase from Streptomyces avermitilis; rFvEn3GAL, recombinant endo-β-1,3-galactanase from Flammulina velutipes; rIl3GAL, recombinant exo-β-1,3-galactanase from Irpex lacteus; rNcArafase, recombinant α-L-arabinofuranosidase from Neurospora crassa; rTv6GAL, recombinant endo-β-1,6-galactanase from Trichoderma viride; V0, void volume; Vi, inner volume.
References
- Akiyama Y, Eda S, Kato K. An arabinogalactan-protein from the leaves of Nicotiana tabacum. Agric Biol Chem. 1982;46:1395–1397.
- Akiyama Y, Eda S, Kato K. Gum arabic is a kind of arabinogalactan-protein. Agric Biol Chem. 1984;48:235–237.
- Gleeson PA, McNamara M, Wettenhall REH, et al. Characterization of the hydroxyproline-rich protein core of an arabinogalactan-protein secreted from suspension-cultured Lolium multiflorum (Italian ryegrass) endosperm cells. Biochem J. 1989;264:857–862.10.1042/bj2640857
- Tryfona T, Liang HC, Kotake T, et al. Carbohydrate structural analysis of wheat flour arabinogalactan protein. Carbohydr. Res. 2010;345:2648–2656.10.1016/j.carres.2010.09.018
- Tryfona T, Liang HC, Kotake T, et al. Structural characterization of Arabidopsis leaf arabinogalactan polysaccharides. Plant Physiol. 2012;160:653–666.10.1104/pp.112.202309
- Shimoda R, Okabe K, Kotake T, et al. Enzymatic fragmentation of carbohydrate moieties of radish arabinogalactan-protein and elucidation of the structures. Biosci Biotechnol Biochem. 2014;78:818–831.10.1080/09168451.2014.910100
- Inaba M, Maruyama T, Yoshimi Y, et al. L-Fucose-containing arabinogalactan-protein in radish leaves. Carbohydr Res. 2015;415:1–11.10.1016/j.carres.2015.07.002
- Ellis M, Egelund J, Schultz CJ, et al. Arabinogalactan-proteins: key regulators at the cell surface? Plant Physiol. 2010;153:403–419.10.1104/pp.110.156000
- Shi H, Kim YS, Guo Y, et al. The Arabidopsis SOS5 locus encodes a putative cell surface adhesion protein and is required for normal cell expansion. Plant Cell. 2003;15:19–32.10.1105/tpc.007872
- Kitazawa K, Tryfona T, Yoshimi Y, et al. β-Galactosyl Yariv reagent binds to the β-1,3-galactan of arabinogalactan proteins. Plant Physiol. 2013;161:1117–1126.10.1104/pp.112.211722
- Motose H, Sugiyama M, Fukuda H. A proteoglycan mediates inductive interaction during plant vascular development. Nature. 2004;429:873–878.10.1038/nature02613
- Mizukami AG, Inatsugi R, Jiao J, et al. The AMOR arabinogalactan sugar chain induced pollen-tube competency to respond to ovular guidance. Curr Biol. 2016;26:1091–1097.10.1016/j.cub.2016.02.040
- Sekimata M, Ogura K, Tsumuraya Y, et al. A β-galactosidase from radish (Raphanus sativus L.) seeds. Plant Physiol. 1989;90:567–574.10.1104/pp.90.2.567
- Hata K, Tanaka M, Tsumuraya Y, et al. α-L-Arabinofuranosidase from radish (Raphanus sativus L.) seeds. Plant Physiol. 1992;100:388–396.10.1104/pp.100.1.388
- Eudes A, Mouille G, Thévenin J, et al. Purification, cloning and functional characterization of an endogenous beta-glucuronidase in Arabidopsis thaliana. Plant Cell Physiol. 2008;49:1331–1341.10.1093/pcp/pcn108
- Okemoto K, Uekita T, Tsumuraya Y, et al. Purification and characterization of an endo-β-(1→6)-galactanase from Trichoderma viride. Carbohydr Res. 2003;338:219–230.10.1016/S0008-6215(02)00405-6
- Tsumuraya Y, Mochizuki N, Hashimoto Y, et al. Purification of an exo-β-(1→3)-galactanase of Irpex lacteus (Polyporus tulipiferae) and its action on arabinogalactan-proteins. J Biol Chem. 1990;265:7207–7215.
- Kotake T, Hirata N, Degi Y, et al. Endo-β-1,3-galactanase from winter mushroom Flammulina velutipes. J Biol Chem. 2011;286:27848–27854.10.1074/jbc.M111.251736
- Fields GB, Van Wart HE, Birkedal-Hansen H. Sequence specificity of human skin fibroblast collagenase. J Biol Chem. 1987;262:6221–6226.
- Showalter AM. Structure and function of plant cell wall proteins. Plant Cell. 1993;5:9–23.10.1105/tpc.5.1.9
- Helm M, Schmid M, Hierl G, et al. KDEL-tailed cysteine endopeptidases involved in programmed cell death, interaction of new cells, and dismantling of extensin scaffolds. Am J Bot. 2008;95:1049–1062.10.3732/ajb.2007404
- Dow JM, Davies HA, Daniels MJ. A metalloprotease from Xanthomonas campestris that specifically degrades proline/hydroxyproline-rich glycoproteins of the plant extracellular matrix. Mol Plant-Microbe Interact. 1998;11:1085–1093.10.1094/MPMI.1998.11.11.1085
- Walter R, Simmons WH, Yoshimoto T. Proline specific endo- and exopeptidases. Mol Cell Biochem. 1980;30:111–127.
- Takata R, Tokita K, Mori S, et al. Degradation of carbohydrate moieties of arabinogalactan-proteins by glycoside hydrolase from Neurospora crassa. Carbohydr Res. 2010;345:2516–2522.10.1016/j.carres.2010.09.006
- Konishi T, Kotake T, Soraya D, et al. Properties of family 79 β-glucuronidases that hydrolyze β-glucuronosyl and 4-O-methyl-β-glucuronosyl residues of arabinogalactan-protein. Carbohydr Res. 2008;343:1191–1201.10.1016/j.carres.2008.03.004
- Kotake T, Kaneko S, Kubomoto A, et al. Molecular cloning and expression in Escherichia coli of Trichoderma viride endo-β-(1→6)-galactanase gene. Biochem J. 2004;377:749–755.10.1042/bj20031145
- Kotake T, Kitazawa K, Takata R, et al. Molecular cloning and expression in Pichia pastoris of a Irpex lacteus Exo-β-(1→3)-galactanase Gene. Biosci Biotechnol Biochem. 2009;73:2303–2309.10.1271/bbb.90433
- Ichinose H, Fujimoto Z, Honda M, et al. A β-L-arabinopyranosidase from sStreptomyces avermitilis is a novel member of glycoside hydrolase family 27. J Biol Chem. 2009;284:25097–25106.10.1074/jbc.M109.022723
- Goldstein IJ, Hay GW, Lewis BS, et al. Controlled degradation of polysaccharides by periodate oxidation, reduction, and hydrolysis. Methods Carbohydr Chem. 1956;5:361–370.
- Dubois M, Gilles KA, Hamilton JK, et al. Colorimetric method for determination of sugars and related substances. Anal Chem. 1956;28:350–356.10.1021/ac60111a017
- McGrath R. Protein measurement by ninhydrin determination of amino acids released by alkaline hydrolysis. Anal Biochem. 1971;49:95–102.
- Bergmeyer HU. Methods in enzymatic analysis. 2nd ed. Vol. 2. New York and London: Academic Press; 1974. p. 967–970.
- Yemm EW, Cocking EC. The determination of amino-acids with ninhydrin. Analyst. 1955;80:209–213.10.1039/an9558000209
- Laemmli UK. Cleavage of structural proteins during the assembly of the head of bacteriophage T4. Nature. 1970;227:680–685.10.1038/227680a0
- Bradford MM. A rapid and sensitive method for the quantitation of microgram quantities of protein utilizing the principle of protein-dye binding. Anal Biochem. 1976;72:248–254.10.1016/0003-2697(76)90527-3
- Schultz CJ, Johnson KL, Currie G, et al. The classical arabinogalactan protein gene family of Arabidopsis. Plant Cell. 2000;12:1751–1767.10.1105/tpc.12.9.1751
- Schultz CJ, Rumsewicz MP, Johnson KL, et al. Using genomic resources to guide research direction. The Arabinogalactan protein gene family as a test case. Plant Physiol. 2002;129:1448–1463.10.1104/pp.003459
- Bond MD, Van Wart HE. Characterization of the individual collagenases from Clostridium histolyticum. Biochemistry. 1984;23:3085–3091.10.1021/bi00308a036
- Ohbayashi N, Yamagata N, Goto M, et al. Enhancement of the structural stability of full-length clostridial collagenase by calcium ions. Appl Environ Microbiol. 2012;78:5839–5844.10.1128/AEM.00808-12
- Sattar AKMA, Yamamoto N, Yoshimoto T, et al. Purification and characterization of an extracellular prolyl endopeptidase from Agaricus bisporus. J Biochem. 1990;107:256–261.
- Yoshimoto T, Satter AKMA, Hirose W, et al. Studies on prolyl endopeptidase from shakashimeji (Lyophyllum cinerascens): purification and enzymatic properties. J Biochem. 1988;104:622–627.
- Fülöp V, Böcskei Z, Polgár L. Prolyl oligopeptides: an unusual β-propeller domain regulates proteolysis. Cell. 1998;94:161–170.10.1016/S0092-8674(00)81416-6
- Juhász T, Szeltner Z, Fülöp V, et al. Unclosed β-propellers display stable structures: implications for substrate access to the active site of prolyl oligopeptidase. J Mol Biol. 2005;346:907–917.10.1016/j.jmb.2004.12.014
- Gibeaut DM, Carpita NC. Tracing cell wall biogenesis in intact cells and plants. Plant Physiol. 1991;97:551–561.10.1104/pp.97.2.551
- Cleland R, Olson AC. Metabolism of free hydroxyproline in Avena coleoptiles. Biochemistry. 1967;6:32–36.10.1021/bi00853a007
- Marinova M, Dolashki A, Altenberend F, et al. Characterization of an aminopeptidase and a proline iminopeptidase from cabbage leaves. Z Naturforsch C. 2008;63:105–112.
- Yoshimoto T, Satter AKMA, Hirose W, et al. Studies on prolyl endopeptidase from carrot (Daucus carota): purification and enzymatic properties. Biochim Biophys Acta. 1987;916:29–37.10.1016/0167-4838(87)90207-X
- García-Lorenzo M, Sjödin A, Jansson S, et al. Protease gene families in Populus and Arabidopsis. BMC Plant Biol. 2006;6:30.10.1186/1471-2229-6-30