Abstract
Of the FET (fused in sarcoma [FUS]/Ewing sarcoma protein [EWS]/TATA binding protein-associated factor 15 [TAF15]) family of heterogeneous nuclear ribonucleoprotein particle proteins, FUS and TAF15 are consistently and EWS variably found in inclusion bodies in neurodegenerative diseases such as frontotemporal lobar degeneration associated with FUS. It is speculated that dysregulation of FET proteins at the post-translational level is involved in their cytoplasmic deposition. Here, the O-linked β-N-acetylglucosamine (O-GlcNAc) glycosylation stoichiometry of the FET proteins was chemoenzymatically analyzed, and it was found that only EWS is dynamically glycosylated with a high stoichiometry in the neural cell lines tested and in mouse brain. It was also confirmed that EWS, but not FUS and TAF15, is glycosylated with a high stoichiometry not only in the neural cells but also in the non-neural cell lines tested. These results indicate that O-GlcNAc glycosylation imparts a physicochemical property on EWS that is distinct from that of the other FET proteins in most of cell lineages or tissues.
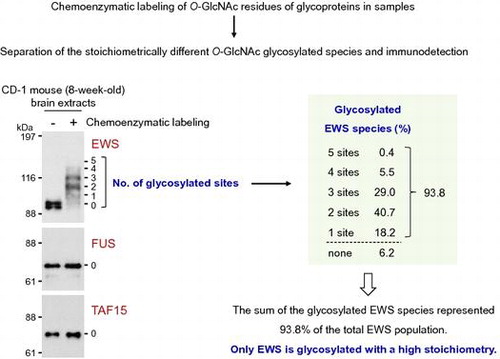
The mammalian FET protein family includes fused in sarcoma (FUS), Ewing sarcoma protein (EWS), and TATA binding protein-associated factor 15 (TAF15). These proteins have a common domain organization, including a low-complexity (LC) domain, RGG domains, zinc finger domain, and an RNA recognition motif.Citation1) All three FET proteins are ubiquitously expressed in most of tissues, bind DNA/RNA, and have been suggested to play multiple roles in the control of transcription, RNA processing and transport, and DNA damage responses.Citation1) FET proteins are of clinical interest because they are co-deposited in cytoplasmic inclusions in neural cells in a subset of patients with neurodegenerative disorders such as frontotemporal lobar degeneration associated with FUS (FTLD-FUS).Citation2) Because FTLD-FUS is not usually associated with mutations in the genes encoding FET proteins,Citation3,4) dysregulation of FET proteins at the post-translational level is speculated to be involved in their cytoplasmic deposition in FTLD-FUS.Citation2) Therefore, determination of the post-translational modification(s) of FET proteins is important to clarify the etiology of FTLD-FUS. Although similarities in the post-translational modifications of FET proteins, including arginine methylation and tyrosine phosphorylation, have been explored,Citation5–11) it is unknown whether they share O-linked β-N-acetylglucosamine (O-GlcNAc) glycosylation status. O-GlcNAc glycosylation is a reversible and dynamic post-translational modification of the Ser/Thr residues of nuclear, cytosolic, and mitochondrial proteins found in multicellular organisms, and is an important modulator of a wide range of cellular functions throughout life.Citation12,13) We and others have reported that EWS is an O-GlcNAc glycosylated proteinCitation14–18) but there are no reports on the glycosylation status of either FUS or TAF15. In the present study, the first comparative analysis of the glycosylation stoichiometry of FET proteins was performed.
Materials and methods
Materials
Anti-EWS (G-5) antibody was purchased from Santa Cruz Biotechnology. Anti-FUS (SAB4200455) and anti-TAF15 (SAB2102361) antibodies were purchased from Sigma–Aldrich. Horseradish peroxidase (HRP)-conjugated anti-mouse IgG and HRP-conjugated anti-rabbit IgG antibodies were obtained from GE Healthcare. All-trans-retinoic acid (RA) was obtained from Wako Pure Chemicals. Thiamet G was obtained from Cayman Chemical.
Cell culture
Mouse embryonic carcinoma P19 cells were grown in Dulbecco’s modified Eagle’s medium (DMEM; Wako Pure Chemicals) supplemented with MEM non-essential amino acids (Wako Pure Chemicals). Mouse neuroblastoma Neuro2a cells and human neuroblastoma SH-SY5Y cells were grown in DMEM/Ham’s F-12 medium (Wako Pure Chemicals). Human glioblastoma A172 cells were obtained from Riken Bio Resource Center and were grown in RPMI1640 medium (Wako Pure Chemicals). Human embryonic kidney 293T cells and human cervical carcinoma HeLa cells were grown in DMEM. All of the cells were cultured in the indicated medium supplemented with 10% heat-inactivated fetal bovine serum, 100 U/mL penicillin, and 100 μg/mL streptomycin at 37 °C under humidified air with 5% CO2.
Protein extraction and western blotting
Whole-cell lysates were prepared in 10 mM Tris–HCl (pH 7.4) containing 0.5% Nonidet P-40, 150 mM NaCl, and protease inhibitor cocktail (Nacalai Tesque), incubated for 5 min, and centrifuged at 13,200 rpm for 15 min. The clarified supernatants were used for western blotting. Total protein concentration of the sample was measured by a Bio-Rad Protein Assay (Bio-Rad). For protein detection in western blots, 2–30 μg of each sample was run on sodium dodecyl sulfate-polyacrylamide gel, electroblotted onto a polyvinylidene difluoride membrane (Millipore), and subjected to immunodetection using an appropriate primary antibody. Proteins were visualized using HRP-conjugated secondary antibodies and enhanced chemiluminescence.
Chemoenzymatic labeling of O-GlcNAc glycosylated proteins
Chemoenzymatic labeling of proteins containing terminal GlcNAc residues with a 5 kDa polyethylene glycol (PEG) mass tag (mPEG-Alkyne, 5340 Da, Creative PEGWorks) was used to quantify the O-GlcNAc glycosylation stoichiometry of proteins.Citation19–21) Briefly, azide-modified galactose (GalNAz) was enzymatically transferred to terminal GlcNAc residues by a mutant β-1,4-galactosyltransferase (Y289L) using a Click-iT O-GlcNAc Enzymatic Labeling System (Thermo Fisher Scientific) according to the manufacturer’s protocol. The PEG mass tag was then reacted with GalNAz by click chemistry using a Click-iT Protein Analysis Detection kit (Thermo Fisher Scientific) according to the manufacturer’s protocol with the exception that the alkyne-labeled biotin was replaced with mPEG-Alkyne at 6 mM final concentration. GalNAz–PEG-labeled proteins were resolved by SDS-PAGE and immunoblotted with an appropriate antibody. The O-GlcNAc glycosylation stoichiometry of proteins was quantified by densitometric analysis of the relative intensities of the bands.
Results
Monitoring of O-GlcNAc glycosylated species of FET proteins before and after the induction of neuronal differentiation
Recently, we reported a dynamic change in the O-GlcNAc glycosylation stoichiometry of EWS species during neuronal differentiation of mouse embryonic carcinoma P19 cells.Citation21) Based on this observation, the glycosylation stoichiometry of FET proteins was examined before and after the induction of the neuronal differentiation of P19 cells using chemoenzymatic labeling of O-GlcNAc residues. Briefly, an engineered mutant, β-1,4-galactosyltransferase, catalyzes the transfer of GalNAz from UDP–GalNAz to terminal GlcNAc residues of glycoproteins. A 5-kDa PEG alkyne tag is then reacted with the transferred GalNAz through Huisgen azide-alkyne cycloaddition. This approach enables separation of the stoichiometrically different glycosylated species upon SDS-PAGE and immunoblotting with antibodies against proteins of interest and quantification of the glycosylation stoichiometry of proteins by densitometry.
Whole-cell lysates were prepared before and after the induction of neuronal differentiation and chemoenzymatically labeled glycoproteins were separated by SDS-PAGE and probed for each member of the FET family by immunoblotting. As shown in Fig. , two antibody-reactive bands were detected above the unmodified EWS band in undifferentiated cells, although the upper band was faint (lane – RA treatment, +GalNAz–PEG reaction). The molecular weights of these bands were approximately 5 and 10 kDa higher than the unmodified EWS band, corresponding to EWS species O-GlcNAc glycosylated at one and two sites, respectively. After the induction of neuronal differentiation, the O-GlcNAc glycosylation stoichiometry of EWS changed greatly and EWS species O-GlcNAc glycosylated at three sites were observed, as previously reported.Citation21) In contrast, O-GlcNAc glycosylated species were undetectable in either FUS or TAF15, irrespective of neuronal differentiation induction (Fig. ). Consistent with these findings, the glycosylation of immunoprecipitated FUS or TAF15 was also undetectable using an O-GlcNAc–specific antibody (CTD110.6) in P19 cells during neuronal differentiation until day 3 (data not shown).
Fig. 1. Monitoring of O-GlcNAc glycosylated species of FET proteins before and after the induction of neuronal differentiation in mouse embryonic carcinoma P19 cells.
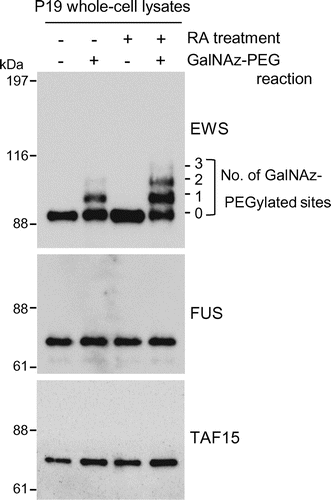
O-GlcNAc glycosylation stoichiometry of FET proteins in neural cell lines
Next, the glycosylation stoichiometry of FET proteins in neural cell lines – such as mouse neuroblastoma Neuro2a, human neuroblastoma SH-SY5Y, and human glioblastoma A172 cells – was examined. In all three cell lines, four antibody-reactive bands were detected above the unmodified EWS band, corresponding to EWS species O-GlcNAc glycosylated at one to four sites, respectively (Fig. (A), lane – thiamet G and +GalNAz–PEG). Densitometric analysis showed that the sum of the glycosylated EWS species in the Neuro2a, SH-SY5Y, and A172 cells represented 83.8, 91.2, and 88.7% of the total EWS population, respectively (Fig. (B)). As shown in Fig. (A) and (B), treatment of the cells with thiamet G, a potent O-GlcNAcase inhibitorCitation22) greatly changed the glycosylation stoichiometry of EWS in all three cell lines, with EWS species O-GlcNAc glycosylated at five and six sites observed. This result indicates that EWS is dynamically glycosylated with a high stoichiometry in these neural cell lines. In contrast, O-GlcNAc glycosylated species were undetectable in either FUS or TAF15 in all three cell lines, even in the presence of thiamet G (Fig. (A)). Incidentally, the molecular weights of FUS and TAF15 in Neuro2a cells were smaller than those in SH-SY5Y and A172 cells, respectively (Fig. (A)). This might be mainly due to the difference in amino acid chain length of the same protein between human and mouse.
Fig. 2. O-GlcNAc glycosylation stoichiometry of FET proteins in neural cell lines.
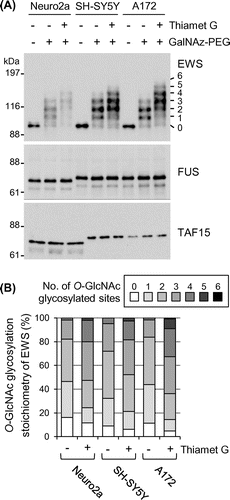
The glycosylation stoichiometry of FET proteins was further examined before and after the induction of neurite outgrowth in the neural cell lines. Neurite outgrowth of SH-SY5Y and A172 cells was induced by treatment with RACitation23) and dibutyryl cyclic AMP/theophylline,Citation24) respectively. The O-GlcNAc glycosylation stoichiometry of EWS appeared to be little affected by neurite outgrowth induction in either SH-SY5Y or A172 cells (Supplementary Fig. ). Furthermore, O-GlcNAc glycosylated species were undetectable in either FUS or TAF15, irrespective of neurite outgrowth induction (data not shown).
A great majority of EWS is normally O-GlcNAc glycosylated in the brain
To examine the glycosylation stoichiometry of FET proteins in the neural cells in vivo, brain extracts from an 8-week-old normal CD-1 mouse underwent the chemoenzymatic labeling approach. As shown in Fig. (A), doublet bands were detected in the EWS blots of the mouse brain without chemoenzymatic labeling (lane – GalNAz–PEG). It has been reported that brain-specific EWS, which is a splicing isoform containing the exon 4A (6 amino acids), is specifically expressed in the central nervous system.Citation25) It is therefore probable that the upper and lower bands correspond to the brain-specific and ubiquitous EWS isoforms, respectively. Five antibody-reactive bands were detected above the unmodified EWS band, corresponding to EWS species O-GlcNAc glycosylated at one to five sites, respectively (Fig. (A), lane +GalNAz–PEG). O-GlcNAc glycosylation of both the brain-specific and ubiquitous EWS isoforms was confirmed but could not distinguish the glycosylation stoichiometry between the two isoforms. The glycosylation stoichiometry was thus evaluated for the mixture of the two isoforms. Densitometric analysis showed that the sum of the glycosylated EWS species represented 93.8% of the total EWS population, with EWS species O-GlcNAc glycosylated at two and three sites dominant among the glycosylated EWS species (Fig. (B)). Similar to the results of the neural cell lines, O-GlcNAc glycosylated species were undetectable in either FUS or TAF15 in the mouse brain extracts (Fig. (A)). These results indicate that, of the FET proteins, only EWS is usually O-GlcNAc glycosylated with a high stoichiometry in neural cells in vivo.
Fig. 3. O-GlcNAc glycosylation stoichiometry of FET proteins in mouse brain.
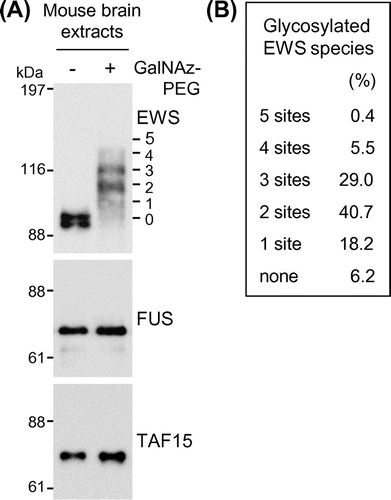
O-GlcNAc glycosylation stoichiometry of FET proteins in non-neural cell lines
Because FET proteins are ubiquitous proteins expressed in most of tissues, the glycosylation stoichiometry of FET proteins in non-neural cell lines – such as human cervical carcinoma HeLa and human embryonic kidney 293T cells – was examined. In the both cell lines, four antibody-reactive bands were detected above the unmodified EWS band, corresponding to EWS species O-GlcNAc glycosylated at one to four sites, respectively (Fig. (A), lane – thiamet G and +GalNAz–PEG). Densitometric analysis showed that the sum of the glycosylated EWS species in the HeLa and 293T cells represented 89.0 and 89.3% of the total EWS population, respectively (Fig. (B)). As shown in Fig. (A) and (B), treatment of the cells with thiamet G greatly changed the glycosylation stoichiometry of EWS in the both cell lines. In contrast, O-GlcNAc glycosylated species were undetectable in either FUS or TAF15 in the both cell lines, even in the presence of thiamet G (Fig. (A)). Similar results with these cell lines were obtained in mouse MC3T3-E1 preosteoblasts, mouse C2C12 myoblasts, and mouse 3T3-L1 preadipocytes (Supplementary Fig. ). These results indicate that EWS, but not FUS and TAF15, is normally O-GlcNAc glycosylated with a high stoichiometry in both neural and non-neural cells.
Fig. 4. O-GlcNAc glycosylation stoichiometry of FET proteins in non-neural cell lines.
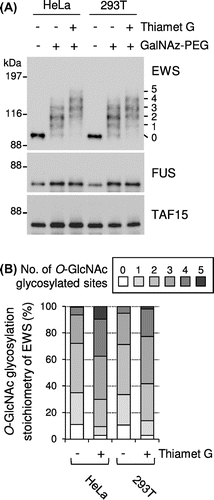
Discussion
Although all three FET proteins bind DNA/RNA and have been suggested to play multiple roles in the control of transcription, RNA processing and transport, and DNA damage responses, the precise control of their multiple functions remains to be elucidated. Here it was found that, of the FET proteins, only EWS is dynamically O-GlcNAc glycosylated with a high stoichiometry. O-GlcNAc glycosylation may therefore selectively regulate EWS functions among the FET proteins.
Given that EWS is found in both the nucleus and cytosol,Citation17,26–31) the physiological functions of EWS may be accomplished in part by its dynamic subcellular distribution. Although the regulatory mechanism for the subcellular localization of EWS is less clear, the N-terminal LC domain and three independent C-terminal domains, including the third RGG motif, the zinc-finger motif, and the PY-nuclear localization signal, are important for its efficient nuclear localization.Citation27,28,31) We have previously shown that O-GlcNAc glycosylation may play a regulatory role in the adipogenesis-dependent nuclear distribution of EWS.Citation17) The cell cycle-dependent dynamic subcellular distribution of EWS has also been reportedCitation30) and Lanza et al. have recently proposed that O-GlcNAc glycosylation controls EWS spindle localization.Citation18) Interestingly, in silico O-GlcNAc glycosylation site analysis using the YinOYang 1.2 server (http://www.cbs.dtu.dk/services/YinOYang/)Citation32) predicted that 55 and 54 Ser/Thr residues are possible modification sites of human EWS (Supplementary Fig. ) and mouse one,Citation17) respectively, and these putative sites are unevenly distributed in the N-terminal LC domain. Therefore, the nuclear localization potency of the LC domain might be regulated by the glycosylation, and identification of the glycosylation sites of EWS will be important to confirm it. In addition, because FET proteins have a common domain organization, elucidation of the molecular mechanism for the EWS-selective glycosylation among the FET proteins may lead to defining the consensus sequence(s) or structural unit(s) for O-GlcNAc glycosylation.
The oncogene EWS-FLI1 encodes a chimeric transcription factor expressed in more than 90% of Ewing’s sarcoma family tumor.Citation33) EWS-FLI1 consists of the EWS N-terminal LC domain and the FLI1 C-terminal DNA-binding domain, and possesses a new transcriptional activity due to the replacement of the inactive transcriptional domain of FLI1 by the EWS LC domain.Citation33) It has been reported that EWS-FLI1 is O-GlcNAc glycosylated in the EWS LC domain and the transcriptional activity of EWS-FLI1 is regulated by the glycosylation.Citation34) In contrast, the functional significance of EWS glycosylation remains unanswered. Interestingly, serine 266 was predicted one of the possible glycosylation sites of human EWS (Supplementary Fig. ) and the same site has been reported as the potent phosphorylation site of human EWS by protein kinase C.Citation35) Phosphorylation of Ser266 has also been identified in the fusion oncoproteins such as EWS-FLI1 and EWS-ATF1.Citation36) It will therefore be important to clarify the involvement of the interplay between O-GlcNAc glycosylation and phosphorylation in multiple roles of EWS in the control of transcription, RNA processing and transport, and DNA damage responses, in addition to the regulation of EWS subcellular localization.
O-GlcNAc glycosylation may also impart a physicochemical property on EWS that is distinct from that of the other FET proteins. FET proteins undergo a reversible phase transition from the soluble to the aggregate state under physiological conditions and the LC domains of FET proteins have the ability to form fibrous assemblies.Citation1,37–40) This ability of the LC domains to form fibrous assemblies is thought to be regulated by post-translational modifications such as phosphorylation.Citation1,40) An attractive model has been proposed that the controlled formation of fibrous assemblies is critical to the physiological functions of FET proteins, but uncontrolled aggregations of FET proteins are pathogenic in neurodegenerative diseases including FTLD-FUS.Citation1) Because FTLD-FUS is not usually associated with mutations in the genes encoding FET proteins,Citation3,4) dysregulation of FET proteins at the post-translational level is speculated to be involved in their cytoplasmic deposition in FTLD-FUS.Citation2) Remarkably, FUS and TAF15 are consistently and EWS variably found in inclusion bodies in neural cells in patients with neurodegenerative disorders such as FTLD-FUS.Citation3,4) Based on this circumstantial evidence, the regulatory mechanism of EWS to form fibrous assemblies seems to be distinct from that of the other FET proteins. O-GlcNAc glycosylation may help to prevent EWS from forming uncontrolled aggregates and accumulating in pathological inclusion bodies such as those found in patients with FTLD-FUS. Further studies are needed to identify O-GlcNAc glycosylation sites of EWS and to prove the pathophysiological effects of the glycosylation on EWS functions.
Author contribution
Kazuo Kamemura conducted experiments and wrote the paper.
Disclosure statement
No potential conflict of interest was reported by the author.
Funding
This work was supported by the Japan Society for the Promotion of Science (JSPS) KAKENHI No. [15K00412], [16K07700] to K.K.
Supplemental materials
The supplemental material for this paper is available at http://dx.doi.org/10.1080/09168451.2016.1263148.
Supplementary_Fig._1__Kamemura_.pptx
Download MS Power Point (131.7 KB)Supplementary_Fig._2__Kamemura_.pptx
Download MS Power Point (119.7 KB)Supplementary_Fig._3__Kamemura_2.pptx
Download MS Power Point (148.9 KB)Notes
Abbreviations: EWS, Ewing sarcoma protein; FTLD, frontotemporal lobar degeneration; FUS, fused in sarcoma; GalNAz, azide-modified galactose; LC, low-complexity; O-GlcNAc, O-linked β-N-acetylglucosamine; PEG, polyethylene glycol; TAF15, TATA binding protein-associated factor 15.
References
- Schwartz JC, Cech TR, Parker RR. Biochemical properties and biological functions of FET proteins. Annu Rev Biochem. 2015;84:355–379.10.1146/annurev-biochem-060614-034325
- Dormann D, Haass C. Fused in sarcoma (FUS): an oncogene goes awry in neurodegeneration. Mol Cell Neurosci. 2013;56:475–486.10.1016/j.mcn.2013.03.006
- Neumann M, Bentmann E, Dormann D, et al. FET proteins TAF15 and EWS are selective markers that distinguish FTLD with FUS pathology from amyotrophic lateral sclerosis with FUS mutations. Brain. 2011;134:2595–2609.10.1093/brain/awr201
- Davidson YS, Robinson AC, Hu Q, et al. Nuclear carrier and RNA binding proteins in frontotemporal lobar degeneration associated with fused in sarcoma (FUS) pathological changes. Neuropathol Appl Neurobiol. 2013;39:157–165.10.1111/nan.2013.39.issue-2
- Klevernic IV, Morton S, Davis RJ, et al. Phosphorylation of Ewing’s sarcoma protein (EWS) and EWS-Fli1 in response to DNA damage. Biochem J. 2009;418:625–634.10.1042/BJ20082097
- Pahlich S, Quero L, Roschitzki B, et al. Analysis of Ewing sarcoma (EWS)-binding proteins: interaction with hnRNP M, U, and RNA-helicase p68/72 within protein-RNA complexes. J Proteome Res. 2009;8:4455–4465.10.1021/pr900235t
- Leemann-Zakaryan RP, Pahlich S, Grossenbacher D, et al. Tyrosine phosphorylation in the C-terminal nuclear localization and retention signal (C-NLS) of the EWS protein. Sarcoma. 2011;2011:218483.
- Dormann D, Madl T, Valori CF, et al. Arginine methylation next to the PY-NLS modulates Transportin binding and nuclear import of FUS. EMBO J. 2012;31:4258–4275.10.1038/emboj.2012.261
- Deng Q, Holler CJ, Taylor G, et al. FUS is phosphorylated by DNA-PK and accumulates in the cytoplasm after DNA damage. J Neurosci. 2014;34:7802–7813.10.1523/JNEUROSCI.0172-14.2014
- Darovic S, Mihevc SP, Župunski V, et al. Phosphorylation of C-terminal tyrosine residue 526 in FUS impairs its nuclear import. J Cell Sci. 2015;128:4151–4159.10.1242/jcs.176602
- Suárez-Calvet M, Neumann M, Arzberger T, et al. Monomethylated and unmethylated FUS exhibit increased binding to Transportin and distinguish FTLD-FUS from ALS-FUS. Acta Neuropathol. 2016;131:587–604.10.1007/s00401-016-1544-2
- Hart GW, Slawson C, Ramirez-Correa G, et al. Cross talk between O-GlcNAcylation and phosphorylation: roles in signaling, transcription, and chronic disease. Annu Rev Biochem. 2011;80:825–858.10.1146/annurev-biochem-060608-102511
- Bond MR, Hanover JA. A little sugar goes a long way: the cell biology of O-GlcNAc. J Cell Biol. 2015;208:869–880.10.1083/jcb.201501101
- Matsuoka Y, Matsuoka Y, Shibata S, et al. Identification of Ewing's sarcoma gene product as a glycoprotein using a monoclonal antibody that recognizes an immunodeterminant containing O-linked N-acetylglucosamine moiety. Hybrid Hybrydomics. 2002;21:233–236.10.1089/153685902760213831
- Wells L, Vosseller K, Cole RN, et al. Mapping sites of O-GlcNAc modification using affinity tags for serine and threonine post-translational modifications. Mol Cell Proteomics. 2002;1:791–804.10.1074/mcp.M200048-MCP200
- Ishihara K, Takahashi I, Tsuchiya Y, et al. Characteristic increase in nucleocytoplasmic protein glycosylation by O-GlcNAc in 3T3-L1 adipocyte differentiation. Biochem Biophys Res Commun. 2010;398:489–494.10.1016/j.bbrc.2010.06.105
- Li Q, Kamemura K. Adipogenesis stimulates the nuclear localization of EWS with an increase in its O-GlcNAc glycosylation in 3T3-L1 cells. Biochem Biophys Res Commun. 2014;450:588–592.10.1016/j.bbrc.2014.06.013
- Lanza C, Tan EP, Zhang Z, et al. Reduced O-GlcNAcase expression promotes mitotic errors and spindle defects. Cell Cycle. 2016;15:1363–1375.10.1080/15384101.2016.1167297
- Rexach JE, Rogers CJ, Yu S-H, et al. Quantification of O-glycosylation stoichiometry and dynamics using resolvable mass tags. Nat Chem Biol. 2010;6:645–651.10.1038/nchembio.412
- Nagel AK, Ball LE. O -GlcNAc modification of the runt- related transcription factor 2 (Runx2) links osteogenesis and nutrient metabolism in bone marrow mesenchymal stem cells. Mol Cell Proteomics. 2014;13:3381–3395.10.1074/mcp.M114.040691
- Kamemura K, Abe H. The glycosylation stoichiometry of EWS species in neuronal cells. Biosci Biotechnol Biochem. 2017;81:165–167. doi:10.1080/09168451.2016.1230004
- Yuzwa SA, Macauley MS, Heinonen JE, et al. A potent mechanism-inspired O-GlcNAcase inhibitor that blocks phosphorylation of tau in vivo. Nat Chem Biol. 2008;4:483–490.10.1038/nchembio.96
- Jensen LM. Phenotypic differentiation of aphidicolin-selected human neuroblastoma cultures after long-term exposure to nerve growth factor. Dev Biol. 1987;120:56–64.10.1016/0012-1606(87)90103-5
- Sakai H, Nakashima S, Yoshimura S, et al. Suppressed expression of CD44 variant isoforms during human glioma A172 cell differentiation induced by cyclic AMP. Neurosci Lett. 1996;210:189–192.10.1016/0304-3940(96)12683-5
- Melot T, Dauphinot L, Sévenet N, et al. Characterization of a new brain-specific isoform of the EWS oncoprotein. Eur J Biochem. 2001;268:3483–3489.10.1046/j.1432-1327.2001.02251.x
- Felsch JS, Lane WS, Peralta EG. Tyrosine kinase Pyk2 mediates G-protein-coupled receptor regulation of the Ewing sarcoma RNA-binding protein EWS. Curr Biol. 1999;9:485–490.10.1016/S0960-9822(99)80214-0
- Rossow KL, Janknecht R. The Ewing’s sarcoma gene product functions as a transcription activator. Cancer Res. 2001;61:2690–2695.
- Zakaryan RP, Gehring H. Identification and characterization of the nuclear localization/retention signal in the EWS proto-oncoprotein. J Mol Biol. 2006;363:27–38.10.1016/j.jmb.2006.08.018
- Andersson MK, Ståhlberg A, Arvidsson Y, et al. The multifunctional FUS, EWS, and TAF15 proto-oncoproteins show cell type-specific expression patterns and involvement in cell spreading and stress response. BMC Cell Biol. 2008;9:37.10.1186/1471-2121-9-37
- Leemann-Zakaryan RP, Pahlich S, Sedda MJ, et al. Dynamic subcellular localization of the ewing sarcoma proto-oncoprotein and its association with and stabilization of microtubules. J Mol Biol. 2009;386:1–13.10.1016/j.jmb.2008.12.039
- Shaw DJ, Morse R, Todd AG, et al. Identification of a tripartite import signal in the Ewing Sarcoma protein (EWS). Biochem Biophys Res Commun. 2009;390:1197–1201.10.1016/j.bbrc.2009.10.120
- Gupta R, Brunak S. Prediction of glycosylation across the human proteome and the correlation of protein function. Pac Symp Biocomput. 2002;7:310–322.
- Ordòñez JL, Osuna D, Herrero D, et al. Advances in Ewing's sarcoma research: where are we now and what lies ahead? Cancer Res. 2009;69:7140–7150.10.1158/0008-5472.CAN-08-4041
- Bachmaier R, Aryee DNT, Jug G, et al. O-GlcNAcylation is involved in the transcriptional activity of EWS-FLI1 in Ewing’s sarcoma. Oncogene. 2009;28:1280–1284.10.1038/onc.2008.484
- Deloulme JC, Prichard L, Delattre O, et al. The prooncoprotein EWS binds calmodulin and is phosphorylated by protein kinase C through an IQ domain. J Biol Chem. 1997;272:27369–27377.10.1074/jbc.272.43.27369
- Olsen RJ, Hinrichs SH. Phosphorylation of the EWS IQ domain regulates transcriptional activity of the EWS/ATF1 and EWS/FLI1 fusion proteins. Oncogene. 2001;20:1756–1764.10.1038/sj.onc.1204268
- Kwon I, Kato M, Xiang S, et al. Phosphorylation-regulated binding of RNA polymerase II to fibrous polymers of low-complexity domains. Cell. 2013;155:1049–1060.10.1016/j.cell.2013.10.033
- Yang L, Gal J, Chen J, et al. Self-assembled FUS binds active chromatin and regulates gene transcription. Proc Nat Acad Sci USA. 2014;111:17809–17814.10.1073/pnas.1414004111
- Patel A, Lee HO, Jawerth L, et al. A liquid-to-solid phase transition of the ALS protein FUS accelerated by disease mutation. Cell. 2015;162:1066–1077.10.1016/j.cell.2015.07.047
- Schüller R, Eick D. Getting access to low-complexity domain modifications. Trends Biochem Sci. 2016;41:894–897.10.1016/j.tibs.2016.05.010