Abstract
Flavobacterium psychrophilum (F. psychrophilum) is the causative agent of bacterial cold-water disease (BCWD) that occurs in ayu Plecoglossus altivelis. Formalin-killed cell of F. psychrophilum has long been studied as an immersion vaccine for BCWD. In this study, we explored the possibility of F. psychrophilum collagenase (fpcol) for use as the immersion vaccine. BCWD convalescent ayu sera contained specific IgM antibodies against somatic F. psychrophilum and fpcol, meaning that fpcol is a promising antigen for the vaccine development. The recombinant fpcol was successfully expressed in Escherichia coli and Brevibacillus chosinensis (B. chosinensis). The culture supernatant of the B. chosinensis was used as an immersion vaccine solution. The vaccinated ayu were then challenged by soaking into F. psychrophilum culture. In two experimental groups, the relative percentages of survivals were 63 and 38%, respectively, suggesting that fpcol is promising as the immersion vaccine for ayu-BCWD.
The Gram-negative bacterium Flavobacterium psychrophilum (F. psychrophilum) is the causative agent of bacterial cold-water disease (BCWD) that occurs in ayu Plecoglossus altivelis and salmonids including rainbow trout Oncorhynchus masou and coho salmon O. kisutch. The isolates obtained from ayu and salmonid fish differ in serologic and genetic types.Citation1,2) The disease symptoms are also known to differ between ayu and salmonid fish. BCWD-affected ayu primarily show symptoms such as anemia (pale gills), hemorrhagic lesions, ulcers on the lower jaw, and ulcerative lesions on the body trunk surface.Citation3) BCWD-affected salmonids primarily show symptoms such as erosion of fins, tail loss, and dermal ulceration.Citation4) Thus, the pathogenic mechanism of ayu isolates is suggested to differ from those of salmonid isolates.
Ayu is one of the most important fish species for freshwater fishery in Japan. It is raised in fish farms as well as stocked in rivers for game fishing. BCWD epidemic has often occurred in both environments with a high mortality rate over the past quarter century; thus, it is one of the most severe problems for freshwater fisheries. In fish farms, the epidemic can be partially controlled by medication with sulfonamide; however, mass mortality sometimes occurs even upon medication. To make matters worse, there is no established approach for treating stocked fish in rivers when BCWD is prevalent. Therefore, a vaccine for ayu-BCWD has been aspired for a long time.
Hitherto, ayu-BCWD vaccine mainly consisting of formalin-killed cell (FKC) of F. psychrophilum, has been studied for a long time. In an experimental environment, the injection-typeCitation5–7) and oral administration-typeCitation8) vaccines have been developed successfully. However, these vaccines are considered impractical because juvenile fish (with an average body weight of less than 1.5 g) cannot withstand the injection, though the disease does occur among fish of this size. With oral administration, the formalin contained within the vaccine sometimes triggers immune tolerance among the vaccinated fish.Citation8, 9) Thus, an immersion-type vaccine is one of the most practical dosage form for ayu-BCWD.
Previous studies have revealed that development of an immersion-type BCWD vaccine is very difficult.Citation10,Citation11) On the other hand, a vaccine for ayu vibriosis, caused by Vibrio anguillarum, had already been developed and approved in Japan. Although the antigen incorporation mechanisms are still unclear, antigens can be presented to the ayu immune system by immersion into the FKC vaccine in case of vibriosis.Citation12, 13) Thus, the development of an immersion-type vaccine for ayu-BCWD should be possible, at least in principle.
Some antigens including glycoprotein P60 (OmpA),Citation14) Flavobacterium-specific protein antigen (FspA),Citation15) 3-hydroxyacyl-CoA dehydrogenase (HCD), ATP synthase beta subunit (atpD), and glutamate dehydrogenase (gdhA)Citation16) have been identified from the F. psychrophilum cell fraction. Thus, it is apparent that the cell fraction contains important potential candidates for a BCWD vaccine. Additionally, a collagenase expressed in ayu isolates is considered promising as one of the potential vaccine candidates.Citation17) The collagenase belonging to M13 metalloprotease family is one of the extracellular proteases, known to be secreted into culture supernatant.Citation17) It is considered to play an important role in the infection process and/or developing the characteristic symptoms of ayu-BCWD, because collagenase cleaves the fibrillar collagen, which is a component of skin, vessels and bones. However, it is difficult to use the native collagenase as a vaccine, because the expression level in F. psychrophilum is very low (unpublished results).
In this study, we explored F. psychrophilum collagenase (fpcol) for use in ayu-BCWD vaccine. The convalescent ayu sera contained the specific IgM antibody for fpcol. Recombinant fpcol was expressed in Brevibacillus chosinensis, and the ayu that received the immersion of the culture medium of fpcol-expressing B. chosinensis exhibited higher immortality toward the challenge of F. psychrophilum. Our results suggest that fpcol is a novel candidate as ayu-BCWD vaccine.
Materials and methods
Fish
Ayu juveniles were reared in Kanagawa Prefectural Fisheries Technology Center Freshwater Experiment Station (Sagamihara, Japan). Fish were confirmed to be F. psychrophilum-free by PCR as described by Yoshiura et al.Citation18) The fish were maintained in 500-L polycarbonate tank flowing fresh water and were fed commercial pellets on a daily basis.
Cultivation of F. psychrophilum
Ayu isolates of F. psychrophilum WA-1 and SG-1 were obtained from NITE Biological Resource Center (NBRC). The accession numbers were NBRC108951 and NBRC111643, respectively. F. psychrophilum cells were cultured in 1/2 CGY medium (2.5 g bacto casitone, 1.5 g gelatin from bovine, and 0.5 g yeast extract in 1 L of distilled water) at 18 °C for 3 days. The cells were collected by centrifugation at 3,500×g for 30 min.
Construction of expression vectors
The genomic DNA of F. psychrophilum WA-1 was prepared from the collected cells as described above, by DNA extraction kit (Sigma–Aldrich, St. Louis, MO). For the expression in Brevibacillus, the 2680-bp fragment (AB921326) was amplified from the genomic DNA using the following nucleotide primers 5′-TTTCGCTGCAGGATCCtatactttgccagtagtatttc-3′ (The small capital sequence is the fpcol-specific sequence) (Y76for) and 5′-CCGGGGTACCGAATTCttattttttaattatttttttag-3′ (K960rev) and KOD Dash DNA polymerase (Toyobo, Osaka, Japan). The PCR fragment was digested with the restriction enzymes BamHI and EcoRI and inserted in pNY326 to produce pNY326-fpcol by DNA ligation kit Mighty Mix (Takarabio, Otsu, Japan) (Fig. (a)).
Fig. 1. Plasmids for the expression of fpcol.
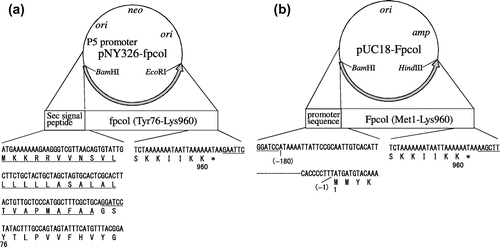
Additionally, for the expression in Escherichia coli (E. coli), the 3,162-bp fragment was amplified from the genomic DNA of F. psychrophilum WA-1 using the following nucleotide primers 5′-CGGTACCCGGGGATCCATTAAATTATTCCGC-3′ (Promoter-for) and 5′-GGCCAGTGCCAAGCTTAAGGCACCTTTTTTG-3′ (Terminator-rev) and KOD Dash DNA polymerase. The PCR fragment was inserted into linearized pUC18 with BamHI and HindIII to produce pUC18-Fpcol by In-Fusion HD cloning kit (Takarabio) as following the instruction manual (Fig. (b)).
Transfection of expression plasmids and selection of transformants
pNY326-fpcol was transfected into Brevibacillus cells according to the manufacturer’s instructions except for the following: the culture was incubated with shaking at 18 °C for 2 h and was transferred into MTNm plates containing 50 μg/mL neomycin and stored at 18 °C for 48 h. Each colony was cultured in 2SY medium (5 mL) containing 50 μg/mL neomycin and 5 mM CaCl2 at 18 °C for five days. The gelatinase activity of the supernatant was assessed by gelatin zymography as described below, and one clone that exhibited the activity was selected.
pUC18-Fpcol was transfected into E. coli JM109 cells according to the conventional calcium chloride method except for the followings: the culture was incubated at 18 °C for 1 h and transferred into LB plates containing 50 μg/mL ampicillin and stored at 18 °C for 48 h. Each colony was cultured in LB medium (5 mL) containing 50 μg/mL ampicillin and 5 mM CaCl2 at 18 °C for five days. The gelatinase activity of the supernatant was assessed by gelatin zymography as described below, and one clone that exhibited the activity was selected.
Expression of fpcol
In the expression in Brevibacillus, the overnight culture (20 mL at 37 °C) of transformed cells was added to 200 mL of 2SYNm medium containing 50 μg/mL neomycin and 5 mM CaCl2 and incubated at predetermined temperature with shaking.
In the expression in E. coli, the overnight culture (20 mL at 25 °C) of the transformed cells was added to 200 mL of LB medium containing 50 μg/mL ampicillin and 5 mM CaCl2 and incubated at predetermined temperature with shaking.
Gelatin zymography
Ten microliters of each samples was applied to gelatin zymography. A gelatin zymography gel containing 10% acrylamide, 0.1% sodium dodecyl sulfate (SDS), and 0.1% gelatin (bovine, Sigma–Aldrich) was prepared. The sample was mixed with an equal volume of 2 × loading dye (62.5 mM Tris–HCl pH 6.8, 25% glycerol, 2% SDS, 0.01% bromophenol blue) and was loaded to the gel. Electrophoresis was performed with a constant voltage in ice-cold water. Then, the gel was washed twice with a washing buffer (50 mM Tris–HCl pH 8.0 and 0.1% polyoxyethylene sorbitan monolaurate (Tween 20)) to remove SDS and was incubated in an incubation buffer (50 mM Tris–HCl pH 8.0, 0.1% Tween 20, and 5 mM calcium chloride) at 24 °C for 16 h. The gel was stained with PhastGel Blue R (GE Healthcare, Little Chalfont, GBR).
Preparation of vaccine solution
Brevibacillus chosinensis SP3 harboring the expression plasmid pNY326-fpcol carrying mature collagenase gene (fpcol; Tyr76-Lys960) was cultured in 2SY medium containing 50 μg/mL neomycin and 5 mM calcium chloride at 37 °C for 1 day and at 15 °C for 8 days (Fig. ). The culture supernatant was collected by centrifugation at 3,500×g for 30 min. The culture supernatant containing a mature fpcol was diluted by 20 times with well water and was used as a vaccine solution. 10 μL of the vaccine solution was applied to gelatin zymography to assay the gelatinolytic activity, as described above.
Fig. 2. Preparation of the vaccine-I solution.
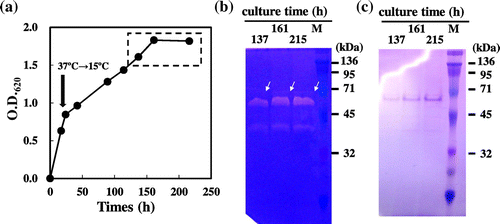
Collagenolytic activity
The collagenolytic activity in the culture supernatant of the recombinant B. chosinensis SP3 and F. psychrophilum WA-1 were measured using fluorescein isothiocyanate (FITC)-labeled type-I collagen, as previously described.Citation17,19,20) Briefly, the supernatant was mixed with 50 mM Tris–HCl buffer (pH 7.5) containing 0.05% FITC-labeled type-I collagen, 5 mM CaCl2 and 200 mM NaCl and incubated at 25 °C for 1 h. After adding EDTA to stop the enzymatic reaction, degraded FITC-labeled collagen fragments were extracted with 50 mM Tris–HCl buffer (pH 9.5) containing 70% ethanol. The fluorescence intensity of the supernatant was measured by fluorescence spectrophotometry (530 nm [emission], 485 nm [excitation]). The results of the collagenase assay were shown as the average of triplicate assays.
Enzyme-linked immunosorbent assay (ELISA) using convalescent ayu sera
Convalescent sera and naïve sera were collected from 10 fish as described below. Blood was withdrawn from the caudal vein using a syringe and sera was separated by centrifugation at 1,500×g for 10 min following the incubation at 4 °C for overnight. The antibody levels against somatic F. psychrophilum and fpcol were determined by ELISA. PBS buffer and the culture supernatant of B. chosinensis SP3 harboring pNY326 mock plasmid, were used as negative controls, respectively. Briefly, 10 mg (wet weight) of F. psychrophilum SG-1 cells was suspended by 50 mL of carbonate buffer (pH 9.6) and was used as a bacterial somatic antigen solution. Instead of the bacterial somatic antigen, the culture supernatant of the recombinant B. chosinensis SP3 was diluted 1:10 in carbonate buffer (pH 9.6) and was also used as a collagenase antigen solution. Each well of ELISA plate (Thermo Scientific, Waltham, MA) was coated with 100 μL of the bacterial somatic antigen solution or the collagenase antigen solution, and the plate was then incubated at 25 °C for 1 h. The plate was blocked with 200 μL of 1% Block Ace (DS Pharma Biomedical, Suita, Japan) in distilled water. Then, 50 μL ayu sera (diluted 1:10 in TBST (20 mM Tris–HCl buffer (pH 7.4) containing 136 mM NaCl, 2 mM KCl, 3 mM sodium azide, 0.05% Tween 20)) were added to each well and incubated for 1 h at 25 °C. The wells were prepared in triplicates. Fifty microliters of an anti-ayu IgM monoclonal antibody (Aquatic Diagnostics Ltd, Stirling, Scotland, GBR) was diluted 1:200 in TBST and added to each well and incubated at 25 °C for 1 h. Then, 50 μL of an anti-mouse IgG AP (Vector Laboratories Inc., Burlingame, CA) was diluted 1:2,000 in TBST and added to each well and incubated for 1 h at 25 °C. The reaction was finally developed by adding 100 μL of disodium 4-nitrophenylphosphate (Wako, Osaka, Japan) and stopped with 100 μL of 2 M NaOH. The plated was read at 405 nm using microplate reader Immuno Mini NJ-2300 (Nalge Nunc). Wells were washed three times after each incubation step with 200 μL of TBST. Results were calculated as the average of three individual readings. The values (Abs.405) of negative controls were used as the base lines.
Vaccination
Experiment-I: 200 g of ayu (approx. 80 tails) were immersed in 1 L of vaccine solution for 5 min, with supplemental aeration provided by air stones.
Control group was prepared by the similar process, except that the vaccine solution was replaced by the same volume of well water. Each 200 g of vaccinated and control group fish were reared by the same condition as described above.
Experiment-II: The vaccinated and control group fish were prepared as experiment-I.
Challenge test of vaccinated fish
Challenge-I: Each 40 fish of vaccinated group and control group from experiment-I were soaked in F. psychrophilum SG-1 culture (4.2 × 107 CFU/mL) for 3 h. The challenged fish were then distributed into 54-L tanks containing 20 fish each and were reared for 14 days. The significant difference in vaccine efficacy was calculated by Fisher’s exact test. The relative percent survival (RPS) was calculated according to the formula: RPS (%) = (1 − mortality of vaccinated fish / mortality of control fish) × 100.
Challenge-II: Each 40 fish of vaccinated group and control group from experiment-II, were challenged by the same procedure as challenge-I.
Results
Expression of fpcol
Active-type fpcol was successfully expressed in both B. chosinensis SP3/pNY326-fpcol and E. coli JM109/pUC18-Fpcol (Fig. and S_Fig. ). Based on the result from gelatin zymography, the molecular masses of fpcol expressed in B. chosinensis (Tyr76-Lys960) were estimated to be 65.4, 54.0, and 42.7 kDa, which was smaller than the predicted molecular mass of mature fpcol (approximately 95 kDa) (Fig. ). The molecular masses of fpcol expressed in E. coli (Met1-Lys960) were estimated to be 110, 100, and 95 kDa, which was almost equal to the predicted molecular mass (approximately 103 kDa) (S_Fig. ). SDS-PAGE showed many extra bands in the E. coli supernatant compared to the B. chosinensis supernatant (Fig. and S_Fig. ). Therefore, the supernatant from B. chosinensis was used for the subsequent vaccination study. The relative collagenolytic activity of this culture supernatant was 254 times higher than that of F. psychrophilum (data not shown). The 20-fold diluted supernatant which showed similar gelatinolytic pattern as Fig. (b) (data not shown) was used as vaccine solution.
Antigenicity of F. psychrophilum cells and recombinant fpcol
To surmise the effect of recombinant fpcol as ayu-BCWD, we examined if convalescent ayu has immunity to fpcol. For this purpose, we established an ELISA for ayu IgM against fpcol, as described in the Materials and methods. We also established an ELISA for ayu IgM against somatic F. psychrophilum as a control. Neither anti-somatic IgM nor anti-fpcol IgM were detected in the sera from naïve ayu, while both were done in the sera from convalescent ayu (Fig. ). These results suggest that fpcol is a promising antigen for the vaccine development.
Immunoprotective efficacy of vaccine
To explore the effect of recombinant fpcol as ayu-BCWD, we examined whether the vaccination of fpcol leads to the survival of ayu challenged by F. psychrophilum. In challenge-I, the mortality rate of the vaccinated group and the control group was 17.5% and 47.5%, respectively, and the relative percent survival (RPS) was 63% (Fig. (a)). In challenge-II, the mortality rate of the vaccinated group and the control group were 12.5% and 20.0%, respectively, and the RPS was 38% (Fig. (b)). On basis of the low mortality of the vaccinated groups in challenge-I and -II, fpcol is considered to be promising as the immersion vaccine for ayu-BCWD. However, the IgM values of vaccinated fish were similar to those of the control fish before the challenges (data not shown).
Discussion
Expression of fpcol
Active-type fpcols were successfully expressed in both B. chosinensis SP3/pNY326-fpcol and E. coli JM109/pUC18-Fpcol. However, their molecular weights were different (Fig. , S_Figs. and ). Although the maturation and secretion mechanisms are still unclear, recombinant fpcol expressed in B. chosinensis was self-digested with possessing the typical catalytic HExxH residuesCitation21,22) and the epitope (Figs. and ).
Antigenicity of F. psychrophilum cells and recombinant fpcol
According to the results of ELISA (Fig. ), both somatic F. psychrophilum and the recombinant fpcol are considered to be important protective antigens. However, because some fish showed low immune reactivity against both antigens (data not shown), it seemed that the immune reactivities depend on individuals even among the convalescent ayu. On the other hand, the naïve sera showed no immune reactivity, indicating that the specificity of ELISA is reliable enough.
F. psychrophilum has been known to secrete not only fpcolCitation17) but also membrane vesicle, metalloproteases Fpp (Flavobacterium psychrophilum protease) 1 and 2Citation23–25) to the culture supernatant. Prior to this study, the vaccine effect of the native culture supernatant had been tested. Regrettably, the native culture supernatant had shown very low vaccine efficacy against ayu-BCWD (our unpublished results). The low vaccine efficacy is considered to be caused by the low expression level of native antigens including fpcol and Fpps. Thus, in this study, the culture supernatant of B. chosinensis SP3 containing the recombinant fpcol was used as vaccine solution.
Immunoprotective efficacy of vaccine
In this study, immersion vaccine showed high and moderate effects in two challenges, respectively (Fig. ). In both challenges, the vaccinated groups showed lower mortality than those of the control group, meaning that the immersion vaccine is moderately effective.
It is considered to be reliable to evaluate a vaccine effect on basis of immersion challenge, because the invasion route is similar to that of the native infection route. However, an immersion challenge of BCWD is considered to be difficult to reproduce a similar mortality.Citation11) Actually, in this study, the accumulated mortality of vaccinated and control group in challenge-II (Fig. (b)) showed lower than those in challenge-I (Fig. (a)). To increase the reproducibility of BCWD vaccine experiment, an evaluation system using i.p. injection may be better.
The IgM values for somatic F. psychrophilum and fpcol before the challenge were similar between control and vaccinated group (data not shown). This suggests that the vaccine may introduce some defensive mechanism against BCWD infection in addition to produce specific antibody against the recombinant fpcol. The mechanisms have left to be revealed.
In this study, the immersion vaccine using recombinant fpcol was shown to be promising. However, it is difficult to be applied into practical use, because of the low reproducibility. Further trials will be needed to develop an immersion BCWD vaccine, such as (1) increasing the efficiency of incorporation of fpcol antigen by longer time immersion and/or an addition of an appropriate adjuvant; (2) increasing the number of booster to two or more; and (3) combining inoculations of fpcol and one or more other antigens.
Supplemental material
Supplemental material for this article can be accessed at http://dx.doi.org/10.1080/09168451.2016.1268041
Author contributions
H.N. designed research; H.N., M.M., K.T., K.Y., H.A., T.H., T.O., K.T., S.M., M.K., and K.A. performed research; H.N., M.M., K.Y., K.T., S.H., T.T., and K.Y. analyzed data; H.N., M.M., and K.Y. wrote the manuscript.
Disclosure statement
No potential conflict of interest was reported by the authors.
Funding
This work was supported by an Adaptable and Seamless Technology Transfer Program grant through Target-driven R&D (A-STEP); the Japan Science and Technology Agency [AS2531213N].
TBBB_1268041_Supplementary_Material.pptx
Download MS Power Point (991 KB)Notes
Abbreviations: BCWD, bacterial cold-water disease; fpcol, Flavobacterium psychrophilum collagenase; FKC, formalin-killed cell; i.p., intraperitoneal; SDS, sodium dodecyl sulfate; ELISA, Enzyme-linked immunosorbent assay; RPS, relative percentage survival.
References
- Izumi S, Wakabayashi H. Further study on serotyping of Flavobacterium psychrophilum. Fish Pathol. 1999;34:89–90.10.3147/jsfp.34.89
- Izumi S, Aranishi F, Wakabayashi H. Genotyping of Flavobacterium psychrophilum using PCR-RFLP analysis. Dis Aquat Organ. 2003;56:207–214.10.3354/dao056207
- Miwa S, Nakayasu C. Pathogenesis of experimentally induced bacterial cold water disease in ayu Plecoglossus altivelis. Dis Aquat Organ. 2005;67:93–104.10.3354/dao067093
- Dalsgaard I. Virulence mechanisms in Cytophaga psychrophila and other Cytophaga-like bacteria pathogenic for fish. Annu Rev Fish Dis. 1993;3:127–144.10.1016/0959-8030(93)90032-7
- Rahman MH, Ototake M, Iida Y, et al. Efficacy of oil-adjuvanted vaccine for coldwater disease in ayu, Plecoglossus altivelis. Fish Pathol. 2000;35:199–203.10.3147/jsfp.35.199
- Rahman MH, Kuroda A, Dijkstra JM, et al. The outer membrane fraction of Flavobacterium psychrophilum induces protective immunity in rainbow trout and ayu. Fish Shellfish Immunol. 2002;12:169–179.10.1006/fsim.2001.0362
- Rahman MH, Ototake M, Nakanishi T. Water-soluble adjuvants enhance the protective effect of Flavobacterium psychrophilum vaccines in ayu Plecoglossus altivelis. Fish Pathol. 2003;38:171–176.10.3147/jsfp.38.171
- Kondo M, Kawai K, Okabe M, et al. Efficacy of oral vaccine against bacterial coldwater disease in ayu Plecoglossus altivelis. Dis Aquat Organ. 2003;55:261–264.10.3354/dao055261
- Joosten PH, Avilés-Trigueros M, Sorgeloos P, et al. Oral vaccination of juvenile carp (Cyprinus carpio) and gilthead seabream (Sparus aurata) with bioencapsulated Vibrio anguillarum bacterin. Fish Shellfish Immunol. 1995;5:289–299.10.1006/fsim.1995.0028
- Nagai T, Sakamoto T. Susceptibility and immune response to Flavobacterium psychrophilum between different stocks of ayu Plecoglossus altivelis. Fish Pathol. 2006;41:99–104.10.3147/jsfp.41.99
- Gómez E, Méndez J, Cascales D, et al. Flavobacterium psychrophilum vaccine development: a difficult task. Microb Biotechnol. 2014;7:414–423.10.1111/1751-7915.12099
- Kawano K, Aoki T, Kitao T. Immersion vaccination and water-borne challenge of ayu (Plecoglossus altivelis) against vibriosis. Fish Pathol. 1983;18:143–149.10.3147/jsfp.18.143
- Kawano K, Aoki T, Kitao T. Efficacy of direct immersion vaccination of ayu Plecoglossus altivelis with Vibrio anguillarum bacterin. Fish Pathol. 1984;18:185–190.10.3147/jsfp.18.185
- Dumetz F, LaPatra SE, Duchaud E, et al. The Flavobacterium psychrophilum OmpA, an outer membrane glycoprotein, induces a humoral response in rainbow trout. J Appl Microbiol. 2007;103:1461–1470.10.1111/j.1365-2672.2007.03359.x
- Crump EM, Burian J, Allen PD, et al. Identification and expression of a host-recognized antigen, FspA, from Flavobacterium psychrophilum. Microbiology. 2005;151:3127–3135.10.1099/mic.0.28140-0
- Kato G, Sakai T, Suzuki K, et al. Antigenic proteins of Flavobacterium psychrophilum recognized by ayu Plecoglossus altivelis antisera. Dis Aquat Organ. 2014;108:103–112.10.3354/dao02679
- Nakayama H, Tanaka K, Teramura N, et al. Expression of collagenase in Flavobacterium psychrophilum isolated from cold-water disease-affected ayu (Plecoglossus altivelis). Biosci Biotechnol Biochem. 2015;80:135–144.
- Yoshiura Y, Kamaishi T, Nakayasu C, et al. Detection and genotyping of Flavobacterium psychrophilum by PCR targeted to peptidyl-prolyl cis-trans isomerase C gene. Fish Pathol. 2006;41:67–71. In Japanese with English abstract.
- Nagai Y, Hori H, Hattori S, et al. A micro-assay method for collagenase activity and its application in the study of collagen metabolism in pathological tissues. Jpn J Inflam. 1984;4:121–130.
- Teramura N, Tanaka K, Iijima K, et al. Cloning of a novel collagenase gene from the gram-negative bacterium Grimontia (vibrio) hollisae 1706B and its efficient expression in Brevibacillus choshinensis. J Bacteriol. 2011;193:3049–3056.10.1128/JB.01528-10
- Watanabe K. Collagenolytic proteases from bacteria. Appl Microbiol Biotechnol. 2004;63:520–526.10.1007/s00253-003-1442-0
- Rawlings ND, Barrett AJ, Finn R. Twenty years of the MEROPS database of proteolytic enzymes, their substrates and inhibitors. Nucleic Acid Res. 2016;44:D343–D350.10.1093/nar/gkv1118
- Secades P, Alvarez B, Guijarro JA. Purification and characterization of a psychrophilic, calcium-induced, growth-phase-dependent metalloprotease from the fish pathogen Flavobacterium psychrophilum. Appl Environ Microbiol. 2001;67:2436–2444.10.1128/AEM.67.6.2436-2444.2001
- Secades P, Alvarez B, Guijarro JA. Purification and properties of a new psychrophilic metalloprotease (Fpp2) in the fish pathogen Flavobacterium psychrophilum. FEMS Microbiol Lett. 2003;226:273–279.10.1016/S0378-1097(03)00599-8
- Aoki M, Kondo M, Nakatsuka Y, et al. Stationary phase culture supernatant containing membrane vesicles induced immunity to rainbow trout Oncorhynchus mykiss fry syndrome. Vaccine. 2007;25:561–569.10.1016/j.vaccine.2006.07.047