Abstract
In our previous study, rice bran protein (RBP) inhibited cholesterol micellar solubility in vitro and decreased serum cholesterol level in rats. In the present study, RBP was separated and purified by size-exclusion chromatography and reversed-phase chromatography. The active protein of RBP related to cholesterol micellar solubility was identified as lectin and non-specific lipid-transfer protein 1 using MALDI-TOF mass spectrometry analysis.
Hypercholesterolemia is a major risk factor for arteriosclerosis. The significance of dietary protein in the development of hypercholesterolemia and atherosclerosis has been extensively demonstrated. Cholesterol is a water-insoluble molecule and its intestinal absorption has a complexity similar to other lipids, including a previous step of micellar solubilization.Citation1) The modulation of cholesterol absorption by dietary protein and other food components is also one of the mechanisms investigated to explain the action of food on lowering serum cholesterol. Some studies have reported that dietary proteins, such as soy proteinCitation2) and sunflower protein hydrolysatesCitation3) were decreased micellar solubility of cholesterol in vitro. SesaminCitation4) and catechinCitation5) also lowered micellar solubility of cholesterol in conjunction with their serum cholesterol-lowering effects in animal.
Rice bran is produced as a by-product during the milling process in the production of white rice from brown rice and it contains 10–16% highly nutritional protein.Citation6) Rice bran protein (RBP) has been recognized as nutritionally superior to other proteins, which have hypoallergenicityCitation7) and anticancer activity.Citation8) Thus, the studies on RBP indicate that it has nutritional and pharmaceutical potential. Only a little information is available about the effects of RBP on cholesterol metabolism. Elisa et al. showed that rice bran enzymatic extract containing 38.1% protein reduced serum cholesterol level in animal experiment.Citation9) Zhang et al. showed that RBP hydrolysate by a pancreatic peptidase trypsin inhibited micellar solubility of cholesterol in vitro without animal experiments.Citation10) We reported that RBP decreased serum cholesterol level in rats. Moreover, we showed that RBP bound to bile acids and inhibited micellar solubility of cholesterol in vitro.Citation11) However, little is known about the mechanism of a hypocholesterolemic action of RBP and the active protein of RBP.
A wide range of fractionation strategies for peptides and proteins are generally available, often combined in multidimensional methods or systems. Some types of chromatographic separation can be used at the protein level, including size exclusion, reversed phase.Citation12) In this study, RBP was separated and purified by a size-exclusion chromatography and a reversed-phase chromatography. Moreover, we evaluated an inhibitory activity of cholesterol micellar solubility of RBP fractions and tried to identify the active protein responsible for cholesterol micellar solubility by MALDI-TOF mass spectrometry.
RBP was provided by Oriza Oil and Fat Chemical CO., LTD (Aichi, Japan). It was consisted of 81.5% protein, 4.9% sugar, 0.5% lipids, 5.6% dietary fiber, 4.1% ash, and 3.4% moisture.
First, RBP was separated according to the procedure described previously with some modifications.Citation13) Size-exclusion chromatography was performed using a HiLoad 26/60 Superdex 200 p.g. column (GE Healthcare) using the AKTA avant 25 system (GE Healthcare). RBP (6.13 g) was dissolved in 200 mL, 10 mM Tris–HCl buffer (pH 8.0), and centrifuged at 25,000×g for 20 min at 4 °C. After centrifugation, the supernatant was filtered and applied to this column. The elution buffer was 10 mM Tris–HCl buffer (pH 8.0). The flow rate was 2.5 mL/min. Online UV absorbance was monitored at 280 nm. The eluted fractions were collected and desalted using a MICRO ACILYZER S1 (ASTOM Corporation, Tokyo, Japan) and freeze-dried.
The typical elution profile of RBP by a HiLoad 26/60 Superdex 200 p.g. column was shown in Fig. (A). These peaks (peak 1−6) were usually detected in the separate experiments. To determine the molecular weight of each of the peaks (1–6), standard proteins were applied to this column. Based on the molecular weights of the standard proteins, the molecular weight of peaks 1, 2, 3, 4, 5, 6 was estimated to be 272, 62, 10, 6, 2, and 0.8 kDa, respectively. The fractions RBPF1~RBPF6 were collected and evaluated the effect of these fractions on cholesterol micellar solubility in vitro using a previously described method.Citation14) The [4-14C] labeled micellar solutions (1.0 mL) were prepared at the following concentrations and mixed by sonication (Ultrasonic Homogenizer, Model VP-5, Taitec, Tokyo, Japan): 0.74 kBq [4-14C]-cholesterol (2.1 Gbq/mmol, PerkinElmer Life Sciences), 0.1 mM cholesterol (Katayama Chemical, Osaka, Japan), 6.6 mM sodium taurocholate (Sigma–Aldrich, Tokyo, Japan), 1 mM oleic acid (Sigma–Aldrich), 0.6 mM phosphatidylcholine (Sigma–Aldrich), 0.5 mM monoolein (Sigma–Aldrich), 132 mM NaCl, and 15 mM sodium phosphate (pH 7.4). After incubation at 37 °C for 24 h, sample (10 g/L, respectively) was added to the micellar solution, solubilized by sonication, incubated at 37 °C for 1 h, and centrifuged at 100,000×g for 60 min at 37 °C. The supernatant was collected for the determination of [14C]-cholesterol content by a liquid scintillation counter.
Fig. 1. The typical elution profile of RBP by HPLC and micellar solubility of cholesterol in vitro.
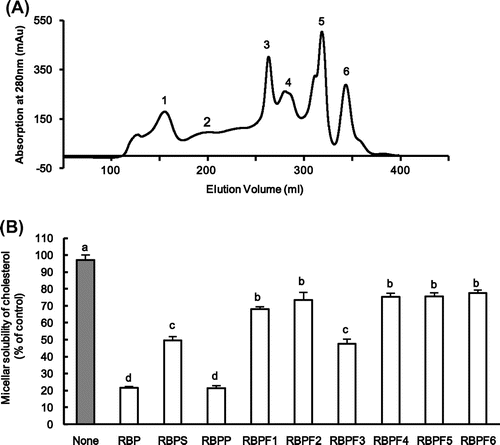
The results were expressed as mean ± SEM. The statistical significance of differences was evaluated by Tukey’s test. Differences were considered significant when p < 0.05.
To investigate whether supernatant (RBPS) or precipitate (RBPP) of RBP inhibits micellar solubility of cholesterol, micellar solubility of cholesterol with RBPS or RBPP in vitro was measured. The cholesterol micellar solubility of RBPP (21.3%) was more effective than that of RBPS (49.7%) as shown in Fig. (B). The cholesterol micellar solubility of RBPF3 (47.6%) similar to RBPS (49.7%) was more effective than other fractions like RBPF1 (68.0%), RBPF2 (73.5%), RBPF4 (75.3%), RBPF5 (75.6%) or RBPF6 (77.8%) as shown in Fig. (B). These results showed that active proteins of RBPS related to the inhibitory action of cholesterol micellar solubility might be concentrated in the RBPF3 than other fractions.
Secondly, RBPF3 (10 mg/mL) was dissolved in 0.065% trifluoroacetic acid (TFA) in 2% acetonitrile and further fractionated by reverse-phase chromatography (RPC), using a column (SOURCE 5RPC ST 4.6/150; GE Healthcare). The conditions for RPC were as follows: Solvent A was 0.065% TFA in 2% acetonitrile and solvent B was 0.05% TFA in 80% acetonitrile. The flow rate was 1 mL/min. The solvent gradient started and kept at 0% B for 20 min, then changed from 0 to 50% B over 100 min, then from 50 to 100% B over 12.5 min. Online UV absorbance was monitored at 280 nm. The eluted fractions were collected at a volume of 2.0 mL and concentrated by a rotary evaporation and freeze dried.
The typical RPC elution profile of RBPF3 was shown in Fig. (A). RBPF3 was separated into RBPF3A (0% B), RBPF3B (0–16.5% B), RBPF3C (16.5–50% B) and evaluated the effect of fractions on micellar solubility of cholesterol in vitro. The cholesterol micellar solubility of RBPF3C (10.1%) was more effective than that of RBPF3A (79.9%) or RBPF3B (81.9%) in Fig. (B).
Fig. 2. The typical elution profile of RBPF3 by reverse-phase column chromatography (RPC) and micellar solubility of cholesterol in vitro.
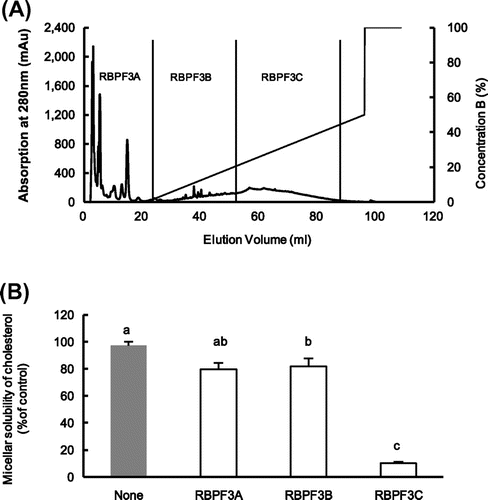
Finally, we identified the active protein from RBPF3C using MALDI-TOF mass spectrometry, RBP contained about 0.58% RBPF3C which is related to the inhibitory activity of cholesterol micellar solubility. The proteins of RBPF3C were identified as non-specific lipid-transfer protein 1 (NCBI accession No. A2ZHF1; 11.3 kDa) and lectin (NCBI accession No. Q01MB6; 22.7 kDa). The matched peptides showed a high homology sequences with the non-specific lipid-transfer protein 1 (sequences coverage, 37.93%, Fig. (A)) and lectin (sequences coverage, 31.28%, Fig. (B)). The exact content of non-specific lipid-transfer protein 1 or lectin in RBP needs to determine in future study.
Fig. 3. Amino acid sequences of non-specific lipid-transfer protein 1 (NCBI accession No. A2ZHF1; 11.3 kDa) and lectin (NCBI accession No. Q01MB6; 22.7 kDa).
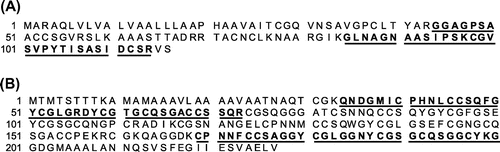
The multiple fractionation or separation methods provide additional information on the analytes, such as molecular weight, hydrophobicity or isoelectric point that can be used to improve identification.Citation12) Many researchers have used in vitro measurements of reduced cholesterol solubilization in micelles or animal studies to identify potential hypocholesterolemic agent.
We discovered that in vitro micellar solubility of cholesterol was significantly suppressed in the presence of soy protein peptic hydrolysate SPH,Citation15) β-lactoglobulin tryptic hydrolysate (LTH),Citation16) and Major Royal Jelly Protein 1 (MRJP1)Citation14) accompanying with their hypocholesterolemic activities induced by the inhibition of intestinal cholesterol absorption in vivo accompanying with the increased excretion of fecal cholesterol.
In this study, we separated and purified the RBPF3C from RBP by SEC and RPC. The cholesterol micellar solubility of RBPF3C was more effective than that of RBP. These results suggest that RBPF3C may have contributed to the hypocholesterolemic action of RBP. Future studies are needed to determine whether the purified RBPF3C improves cholesterol metabolism in vivo.
To our knowledge for the first time we identified the non-specific lipid-transfer protein 1 and lectin as an active protein from RBP related to the inhibitory activity of cholesterol micellar solubility in this study. Kayashima et al. suggested that serum total cholesterol and fecal neutral sterols excretion were significantly increased in rats fed a cholesterol-enriched diet with 0.34% lectin (concanavalin A) for 12 days.Citation17) Thus, the cholesterol-lowering effect may be partially related to the lectin derived from RBP. Carvalho et al. reported that plant non-specific lipid-transfer proteins have the ability to bind or transfer various types of hydrophobic molecules in vitro, such as fatty acids, fatty acyl-CoA, phospholipids, and glycolipids.Citation18) Therefore, non-specific lipid-transfer protein 1 derived from RBP may inhibit cholesterol micellar solubility by binding to hydrophobic components, such as fatty acids, bile acids or phospholipids of micellar components in vitro. These possibilities are currently underway. The result suggest that the hypocholesterolemic action of RBP may be related to some active proteins related to micellar solubility of cholesterol, such as lectin or non-specific lipid-transfer protein 1 or both of them by their combined action. Taken all results together indicate that RBPF3C from RBP is a good source for a functional food and a nutraceutical application to improve cholesterol metabolism.
Disclosure statement
No potential conflict of interest was reported by the authors.
References
- Ros E. Intestinal absorption of triglyceride and cholesterol. Dietary and pharmacological inhibition to reduce cardiovascular risk. Atherosclerosis. 2000;151:357–379.10.1016/S0021-9150(00)00456-1
- Zhong F, Zhang X, Ma J, et al. Fractionation and identification of a novel hypocholesterolemic peptide derived from soy protein alcalase hydrolysates. Food Res Int. 2007;40:756–762.10.1016/j.foodres.2007.01.005
- Megías C, Pedroche J, del Mar Yust M, et al. Sunflower protein hydrolysates reduce cholesterol micellar solubility. Plant Foods Hum Nutr. 2009;64:86–93.10.1007/s11130-009-0108-1
- Hirose N, Inoue T, Nishihara K, et al. Inhibition of cholesterol absorption and synthesis in rats by sesamin. J Lipid Res. 1991;32:629–638.
- Ikeda I, Imasato Y, Sasaki E, et al. Tea catechins decrease micellar solubility and intestinal absorption of cholesterol in rats. Biochim Biophys Acta. 1992;1127:141–146.10.1016/0005-2760(92)90269-2
- Saunders RM. The properties of rice bran as a food stuff. Cereal Food World. 1990;35:632–636.
- Helm RM, Burks AW. Hypoallergenicity of rice bran protein. Cereal Food World. 1996;41:839–843.
- Shoji Y, Mita T, Isemura M, et al. A fibronectin-binding protein from rice bran with cell adhesion activity for animal tumor cells. Biosci Biotechnol Biochem. 2001;65:1181–1186.10.1271/bbb.65.1181
- Revilla E, Santa Maria C, Miramontes E, et al. Nutraceutical composition, antioxidant activity and hypocholesterolemic effect of a water-soluble enzymatic extract from rice bran. Food Res Int. 2009;42:387–393.10.1016/j.foodres.2009.01.010
- Zhang H, Yokoyama WH. Concentration-dependent displacement of cholesterol in micelles by hydrophobic rice bran protein hydrolysates. J Sci Food Agric. 2012;92:1395–1401.10.1002/jsfa.v92.7
- Wang J, Shimada M, Kato Y, et al. Cholesterol-lowering effect of rice bran protein containing bile acid-binding proteins. Biosci Biotechnol Biochem. 2015;79:456–461.10.1080/09168451.2014.978260
- Mostovenko E, Hassan C, Rattke J, et al. Comparison of peptide and protein fractionation methods in proteomics. EuPA Open Proteomics. 2013;1:30–37.10.1016/j.euprot.2013.09.001
- Kashima Y, Kanematsu S, Asai S, et al. Identification of a novel hypocholesterolemic protein, major royal jelly protein 1, derived from royal jelly. PLoS One. 2014;9:e105073.10.1371/journal.pone.0105073
- Nagaoka S, Nakamura A, Shibata H, et al. Soystatin (VAWWMY), a novel bile acid-binding peptide, decreased micellar solubility and inhibited cholesterol absorption in rats. Biosci Biotechnol Biochem. 2010;74:1738–1741.10.1271/bbb.100338
- Nagaoka S, Miwa K, Eto M, et al. Soy protein peptic hydrolysate with bound phospholipids decreases micellar solubility and cholesterol absorption in rats and caco-2 cells. J Nutr. 1999;129:1725–1730.
- Nagaoka S, Futamura Y, Miwa K, et al. Identification of novel hypocholesterolemic peptides derived from bovine milk β-lactoglobulin. Biochem Biophys Res Commun. 2001;281:11–17.10.1006/bbrc.2001.4298
- Kayashima T, Okazaki Y, Katayama T, et al. Dietary lectin lowers serum cholesterol and raises fecal neutral sterols in cholesterol-fed rats. J Nutr Sci Vitaminol. 2005;51:343–348.10.3177/jnsv.51.343
- Carvalho AO, Gomes VM. Role of plant lipid transfer proteins in plant cell physiology – a concise review. Peptides. 2007;28:1144–1153.10.1016/j.peptides.2007.03.004