Abstract
Ninety samples from the extracts of plants from traditional Chinese medicines were screened for antitumor activity. Paeoniflorigenone (PFG) was isolated as an active ingredient from the root of moutan cortex, which showed the strongest activity. In addition, our data indicated that PFG was cytotoxic and induced apoptosis selectively in the cancer cell lines. These effects were cancelled by the addition of caspase inhibitor Z-VAD-FMK, suggesting that it was mediated by caspase-3 activation.
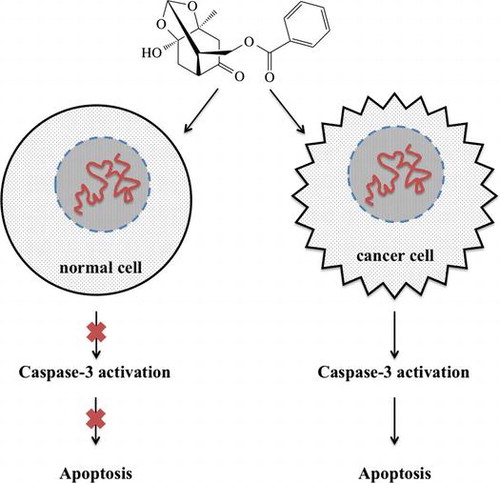
Paeoniflorigenone (PFG), isolated from the root of moutan cortex, was cytotoxic and induced apoptosis selectively in the cancer cell lines mediated by caspase-3 activation.
Terrestrial plants have been used as medicines in China, India, Egypt, and Greece from ancient time, and an impressive number of modern drugs have been developed from them.Citation1) Increasing numbers of consumers are turning to plant medicines for many reasons; low cost and pursuing natural alternatives with fewer side effects, such as toxicity, are commonly cited.Citation2) Plants have been used in the treatment of cancer for a long time.Citation3) Plant-derived compounds have been a significant source of several clinically useful anticancer agents. These include vinblastine and vincristine, the first agents to advance into clinical use.Citation4)
However, these anticancer agents induce DNA damage indiscriminately killing both normal and rapidly proliferating cancer cells. Since these agents are not specifically selective, cancer patients suffer from adverse side effects including infections, fatigue, anemia, hair loss, and nausea.Citation5) Such cytotoxic anticancer treatments are currently being supplemented by targeted therapies to improve the QOL and immunological status of weak patients.Citation6) For this reason, there has lately been an active move toward identifying biologically active substances with anticancer activity from medicine plants, as these are more likely to be innocuous than typical anticancer agents.Citation7)
Apoptosis-inducing ability is known to be dysregulated in many kinds of cancers, and thus, the apoptotic level of cancer cells has been considered a principal indicator of the anticancer activity of potentially therapeutic substances.Citation8,9) Modulation of apoptotic pathways becomes an attractive strategy for the development of anticancer agents particularly if the process is cancer-specific.Citation10,11)
Moutan Cortex (“Mudanpi” in Chinese), the root bark of Paeonia suffruticosa Andrew, is an analgesic, sedative, and anti-inflammatory agent that has been used as a remedy for cardiovascular disorders, extravagated blood, and female genital diseases.Citation12–15) Monoterpenes, such as paeoniflorin, oxypaeoniflorin, and benzoylpaeoniflorin, are the major active substances of Paeonia species.Citation16) Paeoniflorigenone (PFG) isolated from a number of Paeonia species,Citation17–19) was reported to be a depolarizing neuromuscular blocking agent,Citation20) and cytotoxic to N9 microglial cells.Citation21) However, it was reported to be noncytotoxic to melanoma M-14 cells.Citation19)
In this study, 90 samples from the extracts derived from traditional Chinese medicines were screened for apoptosis-inducing activity by MTT assayCitation22) and trypan blue dye exclusion.Citation23) The extract of moutan cortex was identified to be most active. Based on the analyses of biological activities and structural determination, the component of the extract, PFG was isolated and identified to be an active ingredient. Moreover, the apoptosis-inducing effect of PFG toward human cancer cell lines (promyelocytic leukemia HL60, cervical cancer HeLa, T-cell leukemia Jurkat) was evaluated by the MTT assay, trypan blue dye exclusion, Hoechst 33342/PI staining, and DNA ladder analysis, respectively, in comparison with those activities toward normal cell lines (human diploid fibroblast TIG-1 and murine adipocyte 3T3-L1).
Materials and methods
General
Chemicals and solvents were the best grade available and were used as received from commercial sources. NMR spectra were recorded with JEOL JNM-ECA500 (500 MHz for 1H, 125 MHz for 13C) spectrometers. ESI mass spectra were recorded on a LCT premier EX spectrometer (Waters, Milford, MA). Column chromatography was performed with silica gel FL-60D (Fuji Silysia Chem. Ltd., Aichi, Japan). Preparative TLC was performed with glass TLC plates (Merck, 0.5 mm coated silica gel, 60 F254) and PLC plates (Merck, 2.0 mm coated silica gel, 60 F254).
Materials
Natural products of traditional Chinese medicine were purchased from Tong Ren Tang Group Co., Ltd. (Beijing, China). Z-VAD-FMK was obtained from Promega (Madison, USA) and 100 bp DNA ladder marker was procured from Nacalai Tesque Inc. (Kyoto, Japan) and Hoechst 33342/PI from GenScript Inc. (Tokyo, Japan).
Preparation of herbal extracts and isolation of PFG
All natural extracts were prepared from dry herbal products. Briefly, the natural products were ground into a fine powder and then ultrasonically extracted with 70% (v/v) ethanol, respectively. After filtration and removal of the solvent by rotary evaporation, the natural compound extracts were obtained.
PFG was isolated from the traditional Chinese medicine, moutan cortex (Peaonia suffruticosa Andrews) as described previously. The root of moutan cortex (Paeonia suffruticosa Andrews, 2.0 kg) was extracted with 70% (v/v) ethanol. The resulting ethanolic extract was concentrated in vacuo, suspended in H2O, and then successively partitioned with cyclohexane, ethyl acetate, and MeOH to give the corresponding extracts. The MeOH layer (70.4 g) was subjected to silica gel column chromatography with CHCl3, 0, 20, 30, 50, and 95% MeOH to obtain six fractions (A–F). Fraction A (1.75 g) was rechromatographed on silica gel column chromatography and eluted with CHCl3, 0, 2, 10, 25, and 95% MeOH to obtain six fractions (A1–A6). Fraction A1 (1.01 g) was further chromatographed on PLC and eluted with CHCl3–MeOH (98:2) to afford PFG (10 mg) and paeonol (450 mg).
Cell culture
HL60 cells and Jurkat cells were cultured at 37 °C with 5% CO2 in RPMI 1640 Medium (Thermo Scientific, Tokyo, Japan), supplemented with 10% heat-inactivated fetal bovine serum (FBS; Cell Culture Bioscience, Tokyo, Japan), 100 units/mL penicillin, 100 mg/mL streptomycin, 300 mg/mL l-glutamine. HeLa cells, 3T3-L1 cells and TIG-1 cells were cultured at 37 °C with 5% CO2 in MEM (Thermo Scientific) supplemented with 10% heat-inactivated FBS, 100 units/mL penicillin, 100 mg/mL streptomycin, 300 mg/mL l-glutamine.
Cell proliferation and cell viability
HL60 cells and Jurkat cells were seeded at 1 × 104 cells/well in 96-well plates (Iwaki, Tokyo, Japan). HeLa cells, 3T3-L1 cells and TIG-1 cells were seeded at 4 × 103 cells/well in 96-well plates and cultured overnight. Various concentrations of extracts or PFG were then added, and cells were incubated for 24, 48 or 72 h.
After treatment, cell proliferation was determined using the MTT assay. Twenty microliter of MTT (5 mg/mL in PBS) was added to each well and incubated for an additional 4 h at 37 °C. The purple-blue MTT formazan precipitate was dissolved in 200 μL of DMSO and the absorbance values at 550 nm were determined on a multiwell-plate reader. Cell viability was measured using trypan blue dye exclusion, by TC10TM automated cell counter (Bio-Rad Laboratories, Inc.), according to the manufacturer’s protocol.
Since extracts and PFG were dissolved in MeOH, we therefore used 1.5% MeOH as vehicle control. In our pilot study, we found that this concentration of MeOH had no effect on the cell viability.
Inhibition of apoptosis by the caspase inhibitor Z-VAD-FMK
HL60 cells and Jurkat cells were seeded at 6 × 104 cells/well in 24-well plates. HeLa, 3T3-L1 cells and TIG-1 cells were seeded at 8 × 104 cells/well in 24-well plates and cultured overnight. Cells were preincubated with or without 25 mM of Z-VAD-FMK for 30 min. The cells were then treated with or without PFG for 24 h (48 h for TIG-1). They were then stained with trypan blue, and the cell viability was measured.
Double-stain apoptosis detection
Cells were treated with various concentrations of PFG for 24 h. After being washed with PBS and collected, the cell pellet was resuspended in PBS. The cells were washed with PBS and stained with Double Stain Apoptosis Detection Kit (Hoechst 33342/PI) for 30 min, according to the manufacturer’s protocol. After being washed with PBS, the cells were analyzed by fluorescence microscopy (Eclipse E600; Nikon, Tokyo, Japan). The cells were photographed through UV filters at a magnification of ×200 or 100.
DNA ladder analysis
Cells were treated with various concentrations of PFG for 24 or 72 h, and then, washed with phosphate-buffered saline (PBS: 8 g/L NaCl, 200 mg/L KCl, 1.15 g/L Na2HPO4·2H2O, 200 mg/L KH2PO4). The cells were then resuspended in lysis buffer (10 mM Tris-HCl [pH 7.4], 10 mM EDTA, 0.5% Triton X-100,). After centrifugation at 17,700 g, the supernatant was then treated with 0.2 mg/mL RNase A. The lysates were treated with 0.2 mg/mL proteinase K, and 5 M NaCl (0.5 M of total) and the same volume of 2-propanol were added. After the mixtures were stored overnight at −20 °C, DNA was collected by centrifugation at 17,700 g. The pellet was finally dissolved in TE buffer (10 mM Tris-HCl [pH 7.4], 1 mM EDTA). The DNA was then electrophoresed on 2% agarose gel and stained with 1 mg/mL ethidium bromide. The gel was visualized and photographed under UV light.
Caspase 3 activity assay
Caspase 3 (DEVDase) activity of the living cell was analyzed using the NucViewTM 488 Caspase-3 Assay Kit (Biotium, Inc.). NucViewTM 488 Caspase-3 substrate is a novel cell membrane-permeable fluorogenic caspase substrate designed for detecting caspase-3 activity within living cells in real time. Cells treated with various concentrations of PFG for 24 h, were washed with PBS and than collected. The cell pellet was resuspended in PBS. After the addition of NucViewTM 488 Caspase-3 substrate stock solution directly into cell suspension, the cells were incubated at room temperature for 30 min. After being washed with PBS, the cells were analyzed by fluorescence microscopy (Eclipse E600; Nikon, Tokyo, Japan), and the percentage of caspase3-activated cells was determined. The cells were photographed through UV filters at a magnification of 100×.
Statistical analysis
Data are presented in the statistics of four independent experiments in the form of mean ± SD Statistical comparison between groups was made using ANOVA followed by the Bonferroni parametric t-test. Differences were considered significant if p < 0.05.
Results
Screening of cell proliferation inhibitors in TCM extracts
To evaluate the effects of 90 TCM extracts on cell proliferation, human promyelocytic leukemia cells (HL60) were cultured with varying concentrations of 90 extracts for 72 h, and then determined using MTT assay, respectively. Exposure to 19 of 90 extracts produced a dose-dependent suppression of cell proliferation in HL60 cells, with the cytostatic IC50 under 50 μg/mL (Tables and ). In addition, the cell viability was determined by trypan blue dye exclusion in order to discover whether the cytostatic effects on HL60 cells were due to the cytotoxicity of the 19 extracts. Both IC50 (cytostatic IC50 and cytotoxic IC50) of 19 extracts on HL60 cells, and their ratio (cytotoxic IC50/cytostatic IC50) were shown in Table . The extract from moutan cortex, was identified to be the most active one, which had the highest ratio among the 19 extracts.
Table 1. Antitumor activity of screened TCMs.
Table 2. TCMs with (left) or without (right) low antiproliferative effect.
Subsequently, we successfully isolated an anti-tumor fraction (10 mg) from extract of moutan cortex (2 kg), showing a single spot Rf 0.31 on TLC (hexane–ethyl acetate, 1:1). The molecular formula of this compound, C17H18O6, was established by HRESIMS at m/z 319.2178 [M + H]+(calcd for C17H19O6 319.3292). The 1H-NMR(500 MHz, CDCl3) spectrum displayed a methyl proton at δH 1.31 (s, 3H), four methylene protons at δH 2.21, 2.38 (each dd, J = 13.6, 2.4 Hz, 1H) and 2.68 (q, J = 14.5 Hz, 2H), two methine protons in the region of δH 2.38–2.41 (m, 1H) and 2.90–2.91 (m, 1H), and two methyleneoxy protons at δH 4.05 and 4.38 (each dd, J = 11.6, 5.6 Hz, 1H), an acetal proton at δH 5.47 (s, 1H), and five aromatic protons in the region of δH 7.41–8.19. The 13C-NMR (125 MHz, CDCl3) spectrum showed signals assignable to a methyl (δC 21.27), three methylenes (δC 34.32, 46.93, 62.70), three methines (δC 43.15, 46.39, 99.82), two quaternary carbons (δC 78.69, 101.77), six aromatic carbons (δC 128.56, 129.76, 129.85, 133.33), an ester carbonyl (δC 166.25) and a ketonic carbonyl (δC 209.65). [α]D + 6.3°(c = 4.5, MeOH). This viscous oily fraction was identified as PFG by comparison of its spectroscopic data with Shimizu et al.Citation17) Also according to the data of Shimizu et al., [α]D + 4.3°(c = 0.69, MeOH), our data indicated a concurrence of absolute configuration.
Effects on cell proliferation of PFG
Cell viability and cell proliferation were measured to evaluate the effects of PFG on normal cell lines (TIG-1 and 3T3-L1) and tumor cells (HL60, Jurkat and HeLa). As shown in Fig. (A), exposure of the cells to PFG (30 μM) significantly decreased the cell proliferation of tumor cell lines and inhibited the proliferation of normal cell lines. After 72 h of incubation, PFG showed strong cytotoxicity toward tumor cells in a dose-dependent manner; however, weak cytotoxicity toward normal cell lines (Fig. (B)). In addition, only for tumor cells, PFG-induced cell death was suppressed in the presence of Z-VAD-FMK, an irreversible and cell-permeable inhibitor of caspases 3, 8, and 9 (Fig. (C)). Thus, PFG was likely to induce apoptotic cell death in tumor cell lines.
Fig. 1. Antiproliferative effect of PFG against normal cells and cytotoxicity of PFG against tumor cells.
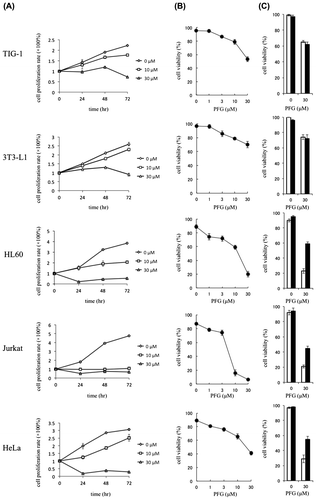
Induction of apoptosis by PFG in tumor cells
To investigate whether PFG can induce apoptosis, tumor cells (HL60, Jurkat, HeLa) and normal cells (TIG-1 and 3T3-L1) were incubated with various concentrations of PFG for 24 and 72 h, respectively, and DNA fragmentation was detected using a DNA ladder analysis. As shown in Fig. , PFG significantly induced apoptotic DNA fragmentation selectively in tumor cells. Moreover, Hoechst 33342/PI staining showed that most nuclei in PFG-treated TIG-1 cells displayed uniform blue chromatin with organized structure as same as the control, while cells dead of 30 μM PFG did not display condensed nuclei and chromatin fragmentation (Fig. (B)). However, PFG treatment resulted in apoptotic morphological changes against Jurkat cells (i.e., a bright blue fluorescent condensed nuclei and chromatin fragmentation, by fluorescence microscope Fig. (A)), with increased frequency compared to control. Hoechst 33342/PI staining of PFG-treated 3T3-L1, HL60 and HeLa showed the similar results with TIG-1 and Jurkat, respectively (data not shown). These results indicated that PFG induced tumor-selective apoptosis.
Fig. 2. DNA ladder analysis.
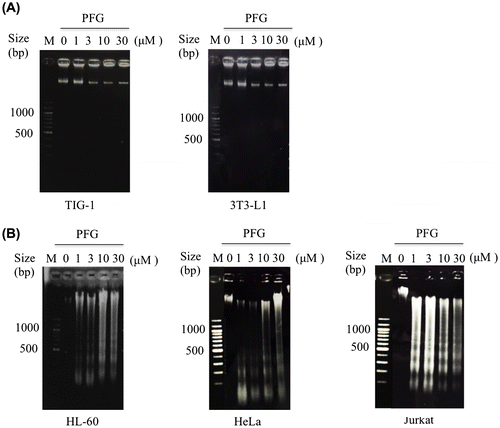
Fig. 3. Effects of PFG on apoptosis of tumor cell lines.
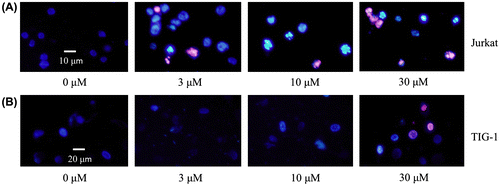
Involvement of caspase-3 in PFG-induction apoptosis of tumor cells
It was found that PFG induced a marked increase in expression and activity of caspase-3 in tumor cells after 24-h treatment in comparison with normal cells treated with PFG (Fig. (A) and (C)). Higher concentration of PFG was more effective than the lower concentration, while PFG had no significant effect on caspase-3 against TIG-1 cells (Fig. (B)).
Fig. 4. Effects on activation of caspase-3 in 4 human cell lines.
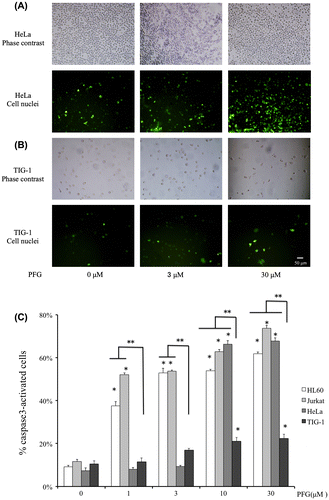
Discussion
The present study demonstrated that (1) moutan cortex delivered from traditional Chinese medicine showed high cytosatic and low cytotoxic activity against HL60 cells; (2) PFG isolated from moutan cortex was verified to be an active ingredient; (3) PFG-induced tumor-selective apoptosis in dose-dependent manner; (4) Caspase-3 participated in the apoptotic effects of PFG.
The anti-tumor potential of components from traditional Chinese medicines has been of great interest. According to our study, moutan cortex and Rubia cordifolia showed high cytostatic effect and low cytotoxicity against HL60 cells. Both of these two plants are well known as important crude drugs having antidiabetic action, anti-inflammatory activity, and antioxidant activity has been used as a remedy for extravasated blood and female genital diseases in Chinese traditional medicine.Citation24,25) Although the major antitumor active substances of these herbal plants are well investigated, such as peaonol from moutan cortexCitation26) and RA-700 from Rubia cordifolia,Citation27) these plants are still rich resource of antitumor agents using the screening method of our study.
PFG, known as a monoterpene, has already been isolated from Peaony root in 1983, but its capacity to induce apoptosis in tumor cell lines has not yet been elucidated. Induction of apoptosis in tumor cells, determined by various methods such as DNA fragmentation, nuclear condensation, and caspase activation, has been recognized as a possible property of monoterpenes.Citation28,29) The apoptotic effects of PFG isolated from moutan cortex have not been previously investigated on HL60, Jurkat or HeLa. Our present study demonstrated that PFG can promote apoptosis of these three tumor cell lines. Interestingly, PFG did not induce apoptosis toward normal cells. The results suggested that cell cycle arrest might contribute to the antiproliferation effects of PFG on normal cell lines.
Apoptosis is induced through two major pathways, Receptor-mediated pathway and cell stress pathway. The two pathways converge at caspase-3 activation,Citation30) and thus, caspase-3 is considered as a key enzyme in the pathogenesis of cell apoptosis. Our study showed that PFG significantly increased the activity of caspase-3 significantly against tumor cell lines.
“Cagelike” monoterpenes are one of the major biologically active components existing in Paeonia species. Paeoniflorin (PF) is well known as a “Cagelike” monoterpene glycoside and has the same monoterpene unit with PFG. Tsuboi et al. reported that PF (100–500 μg/mL; (0.2–1 mM)) induced apoptosis of Jurkat cells,Citation31) while our study showed PFG (1–30 μM) significantly promoted the apoptosis of Jurkat cells. The active concentration of PFG is over 200-fold lower than that of PF against Jurkat cells. Similarly, Zhang and Zhang reported that PF (250–3000 μg/mL; (0.5–6 mM)) induced apoptosis of HeLa cells,Citation32) and its activity was over 60-fold weaker than that of PFG. Thus, the carbonyl group might be a key factor.
In addition, the antiproliferative effects of PFG were evaluated against a disease-oriented panel composed of 39 human cancer cell lines at the Japanese Foundation for Cancer ResearchCitation33) (data not shown). The average GI50 value (the concentration for inhibiting 50% proliferation of control cells) across all of the cell lines tested was 14.1 μM, and PFG exhibited differential antiproliferative effects: the caucasian ovary adenocarcinoma SK-OV-3 (GI50: 2.24 μM), the prostate cancer PU-145 (GI50: 4.68 μM), and the renal adenocarcinoma ACHM (GI50: 4.79 μM) were especially sensitive.
The effect of PFG on histone deacetylase (HDAC), epithelial–mesenchymal transition (EMT), the protein kinase, androgen receptor (AR), and hypoxia-inducible factor (HIF) were investigated by Screening Committee of Anticancer Drugs, but PFG did not show any potent activity at 10 μM.
In conclusion, the results obtained in the present study indicate PFG induced apoptosis selectively in cancer cell lines. The effects might be mediated by caspase-3 activation. Future studies will determine the mechanisms of PFG-induced apoptosis in cancer cells and anti-proliferation in normal cells. In addition, it would be relevant to investigate the quantitative structure-activity relationship from PFG.
Author contribution
HY and MK conceived and designed the study. HY performed the experiments and wrote the paper. OO helped to draft the manuscript. OO and SK participated in the design of the experiments and performed the structural analysis. All authors read and approved the manuscript.
Disclosure statement
No potential conflict of interest was reported by the authors.
Acknowledgements
We thank the Screening Committee of Anticancer Drugs supported by Grant-in-Aid for Scientific Research on Innovative Areas, Scientific Support Programs for Cancer Research, from The Ministry of Education, Culture, Sports, Science and Technology, Japan for the cancer cell line assay, the HDAC assay, the EMT assay, the protein kinase inhibition assay, the AR assay, and the HIF assay.
References
- Shoeb M. Anticancer agents from medicinal plants. Bangladesh J Pharmacol. 2006;1:35–41.
- Bown D. Encyclopaedia of herbs and their uses. London: Dorling Kindersley; 1995.
- Hartwell JL. Plants used against cancer. Lawrence (MA): Quarterman; 1982.
- Cragg GM, Newman DJ. Plants as a source of anti-cancer agents. J Ethnopharmacol. 2005;100:72–79.10.1016/j.jep.2005.05.011
- Kawabe T. G2 checkpoint abrogators as anticancer drugs. Mol Cancer Ther. 2004;3:513–519.
- Maione PA, Rossi A, Airoma G, et al. The role of targeted therapy in non-small cell lung cancer. Crit Rev Oncol Hematol. 2004;51:29–44.10.1016/j.critrevonc.2004.02.002
- Newman DJ, Cragg GM. Natural products as sources of new drugs over the last 25 years. J Nat Prod. 2007;70:461–477.10.1021/np068054v
- Nagata S. Apoptosis by death factor. Cell. 1997;88:355–365.10.1016/S0092-8674(00)81874-7
- Wyllie AH. Apoptosis: an overview. Br Med Bull. 1997;53:451–465.10.1093/oxfordjournals.bmb.a011623
- Beesoo R, Neergheen-Bhujun V, Bhagooli R, et al. Apoptosis inducing lead compounds isolated from marine organisms of potential relevance in cancer treatment. Mutation Res. 2014;768:84–97.10.1016/j.mrfmmm.2014.03.005
- Rossi D, Gaidano G. Messengers of cell death: apoptotic signaling in health and disease. Haematologica. 2003;88:212–218.
- Kubo Y, Tani T, Kosoto H, et al. Studies on moutan cortex (I) historical analysis. Shoyakugaku Zasshi. 1979;33:155–165 [in Japanese].
- Li Q. Pharmacopoeia of the People’s Republic of China [M], 2015. Chin Trad Herb Drugs. 1988;19:276–278.
- Hirai A, Terano T, Hamazaki T, et al. Studies on the mechanism of antiaggregatory effect of moutan cortex. Thromb Res. 1983;31:29–40.10.1016/0049-3848(83)90005-1
- Okubo T, Nagai F, Seto T, et al. The inhibition of phenylhydroquinone-induced oxidative DNA cleavage by constituents of moutan cortex and Paeoniae radix. Biol Pharm Bull. 2000;23:199–203.10.1248/bpb.23.199
- Tanaka T, Kataoka M, Tsuboi N, et al. New monoterpene glycoside esters and phenolic constituents of Paeoniae radix, and increase of water solubility of proanthocyanidins in the presence of paeoniflorin. Chem Pharm Bull. 2000;48:201–207.10.1248/cpb.48.201
- Shimizu M, Hayashi T, Morita N, et al. The structure of Paeoniflorigenone, a new monoterpene isolated from Paenoiae radix. Chem Pharm Bull. 1983;31:577–583.10.1248/cpb.31.577
- Papandreou V, Magiatis P, Kalpoutzakis E, et al. New salicylic glycoside from the Greek endemic species Paeonia clusii. Z Naturforsch. 2002;57c:235–238.
- Mencherini T, Picerno P, Festa M, et al. Triterpenoid constituents from the roots of Paeonia rockii sp. rockii. J Nat Prod. 2011;74:2116–2121.10.1021/np200359v
- Kimura M, Kimura I, Nojima H, et al. Blocking effects of a new component, Paeoniflorigenone, in paeony root on neuromuscular junctions of frogs and mice. Jpn J Pharmacol. 1984;35(1):61–66.10.1254/jjp.35.61
- Duan WJ, Yang JY, Chen LX, et al. Monoterpenes from Paeonia albiflora and their inhibitory activity on nitric oxide production by lipopolysaccharide-activated microglia. J Nat Prod. 2009;72(9):1579–1584.10.1021/np9001898
- Mosmann T. Rapid colorimetric assay for cellular growth and survival: application to proliferation and cytotoxicity assays. J Immunol Methods. 1983;65:55–63.10.1016/0022-1759(83)90303-4
- Strober W. Trypan blue exclusion test of cell viability. Curr Protoc Immunol. 1997; A.3B.1–A.3B.2.
- Son JK, Jung SJ, Jung JH, et al. Anticancer constituents from the roots of Rubia cordifolia L. Chem Pharm Bull. 2008;56(2):213–216.10.1248/cpb.56.213
- Ding LQ, Zhao F, Chen LX, et al. New monoterpene glycosides from Paeonia suffruticosa Andrews and their inhibition on NO production in LPS-induced RAW 264.7 cells. Bioorg Med Chem Lett. 2012;22:7243–7247.10.1016/j.bmcl.2012.09.034
- Bao MH, Zhang YW, Zhou HH. Paeonol suppresses oxidized low-density lipoprotein induced endothelial cell apoptosis via activation of LOX-1/p38MAPK/NF-κB pathway. J Ethnopharmacol. 2013;146:543–551.10.1016/j.jep.2013.01.019
- Hamanaka T, Ohgoshi M, Kawahara K, et al. A novel antitumor cyclic hexapeptide (RA-700) obtained from Rubiae radix. J Pharmacobiodyn. 1987;10(11):616–623.10.1248/bpb1978.10.616
- Mansoor TA, Ramalho RM, Mulhovo S, et al. Induction of apoptosis in HuH-7 cancer cells by monoterpene and β-carboline indole alkaloids isolated from the leaves of Tabernaemontana elegans. Bioorg Med Chem Lett. 2009;19:4255–4258.10.1016/j.bmcl.2009.05.104
- Mansoor TA, Borralho PM, Dewanjee S, et al. Monoterpene bisindole alkaloids, from the African medicinal plant Tabernaemontana elegans, induce apoptosis in HCT116 human colon carcinoma cells. J Ethnopharmacol. 2013;149(2):463–470.10.1016/j.jep.2013.06.051
- Sugawara T, Fujimura M, Noshita N, et al. Neuronal death/survival signaling pathways in cerebral ischemia. Neuro Rx. 2004;1:17–25.10.1602/neurorx.1.1.17
- Tsuboi H, Hossain K, Akhand AA, et al. Paeoniflorin induces apoptosis of lymphocytes through a redox-linked mechanism. J Cell Biochem. 2004;93:162–172.10.1002/(ISSN)1097-4644
- Zhang LL, Zhang SL. Modulating Bcl-2 family proteins and caspase-3 in induction of apoptosis by paeoniflorin in human cervical cancer cells. Phytother Res. 2011;25:1551–1557.10.1002/ptr.v25.10
- Yamori T, Matsunaga A, Sato S, et al. Potent antitumor activity of MS-247, a novel DNA minor groove binder, evaluated by an in vitro and in vivo human cancer cell line panel. Cancer Res. 1999;59:4042–4049.