Abstract
l-Xylulose reductase (LXR) catalyzes the reduction of l-xylulose to xylitol in the fungal l-arabinose catabolic pathway. LXR (RpLXR) was purified from the pentose-fermenting zygomycetous fungus Rhizomucor pusillus NBRC 4578. The native RpLXR is a homotetramer composed of 29 kDa subunits and preferred NADPH as a coenzyme. The Km values were 8.71 mM for l-xylulose and 3.89 mM for dihydroxyacetone. The lxr3 (Rplxr3) gene encoding RpLXR consists of 792 bp and encodes a putative 263 amino acid protein (Mr = 28,341). The amino acid sequence of RpLXR showed high similarity to 3-oxoacyl-(acyl-carrier-protein) reductase. The Rplxr3 gene was expressed in Escherichia coli and the recombinant RpLXR exhibited properties similar to those of native RpLXR. Transcription of the Rplxr3 gene in R. pusillus NBRC 4578 was induced in the presence of l-arabinose and inhibited in the presence of d-glucose, d-xylose, and d-mannitol, indicating that RpLXR is involved in the l-arabinose catabolic pathway.
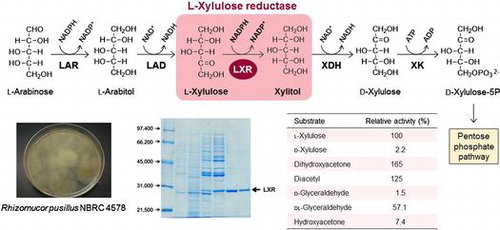
Lignocellulosic biomasses such as rice straw, wheat straw, and corn cob are used as sources of bioethanol because they are not food sources. They are mainly composed of cellulose, hemicellulose, and lignin, and l-arabinose is the most abundant pentose after d-xylose in hemicellulose. Thus, novel ethanol fermentation systems using microorganisms fermenting l-arabinose in addition to d-glucose and d-xylose need to be developed for the efficient use of lignocellulosic biomasses.
The first reaction in l-arabinose catabolic pathway in eukaryotic microorganisms is the reduction of l-arabinose to l-arabitol. This reductive reaction of l-arabinose differs depending on the microorganism. In Hypocrea jecorina (Trichoderma reesei), the d-xylose reductase (XR; EC 1.1.1.307) involved in d-xylose catabolic pathway is also responsible for this reaction.Citation1) In Aspergillus nigerCitation2,3) and Penicillium chrysogenum,Citation4) two specific pentose reductases, XR and l-arabinose reductase (LAR; EC 1.1.1.21), are present, and it was revealed that XR and LAR catalyzed the reduction of d-xylose and l-arabinose, respectively, in A. niger.Citation3) After that, l-arabitol is serially converted into l-xylulose, xylitol, and d-xylulose by the actions of l-arabitol dehydrogenase (LAD; EC 1.1.1.12), l-xylulose reductase (LXR; EC 1.1.1.10), and xylitol dehydrogenase (XDH; EC 1.1.1.9), respectively (Fig. ). In the final step, d-xylulose is phosphorylated to l-xylulose-5-phosphate, an intermediate of the pentose phosphate pathway, by d-xylulokinase (XK; EC 2.7.1.17).
Fig. 1. l-Arabinose and d-xylose catabolism pathways in fungi and yeasts. LAR; l-arabinose reductase, LAD; l-arabinitol dehydrogenase, LXR; l-xylulose reductase, XR; d-xylose reductase, XDH; xylitol dehydrogenase.
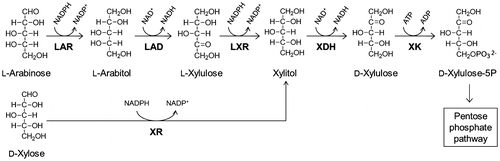
LXR which catalyzes the third reaction belongs to the short-chain dehydrogenase/reductase (SDR) superfamily. However, studies on LXR have been limited and its properties were partially identified on A. nigerCitation5,6) and H. jecorinaCitation7–Citation9) only. Particularly, the few attempts to identify the LXR gene have been associated with some difficulties. Firstly, the lxr1 gene (GenBank accession No. AF375616) encoding NADPH-dependent LXR was cloned from H. jecorina,Citation7) after a while, it was reported that the gene coded for d-mannitol dehydrogenase (MDH).Citation8) The lxrA gene (JGI177736) encoding LXR was cloned from A. niger, and a strain lacking the gene lost NADPH-dependent LXR activity.Citation6) Recently, a novel LXR and the corresponding lxr3 gene (GenBank accession No. BK008567) were also identified in H. jecorina.Citation9) However, the enzymological properties of other fungal LXRs have not been fully understood and need to be examined in order to obtain a more comprehensive understanding of L-arabinose catabolism.
Several strains of zygomycetous fungi,Citation10,11) including Rhizomucor pusillus NBRC 4578Citation12,13) and Mucor circinelloides NBRC 45721,Citation14,15) produce ethanol from d-xylose as well as d-glucose. We previously reported the enzymological and physicochemical properties of XDH (RpXDH)Citation12) and XR (RpXR)Citation13) from R. pusillus NBRC 4578, and XK from M. circinelloides NBRC 4572.Citation15) These strains utilized l-arabinose as well as d-xylose as a carbon source, but did not produce ethanol from l-arabinose.Citation13,15) In order to further clarify the l-arabinose catabolic pathway of R. pusillus NBRC 4578, we purified LXR (RpLXR) from this strain and determined its biochemical and physicochemical characteristics. Furthermore, the lxr3 (Rplxr3) gene encoding RpLXR was cloned based on its internal amino acid sequences and expressed in Escherichia coli cells. The expression of Rplxr3 in R. pusillus NBRC 4578 was also analyzed on various sugars in order to elucidate the induction conditions of the gene.
Materials and methods
Strains
R. pusillus NBRC 4578 was cultured in XY medium (1% yeast extract, 1.5% (NH4)2SO4, 0.7% KH2PO4, 0.1% MgSO4·7H2O, pH 5.5) containing 20 g/L of a carbon source. E. coli JM109 and BL21 (DE3) cells harboring pT7Blue T-vector or pET-15b vector (Takara Bio Inc., Shiga, Japan) were cultured in Luria-Bertani (LB) medium containing 50 μg/mL of ampicillin.
Purification of native RpLXR
R. pusillus NBRC 4578 was cultured at 30 °C for 72 h on a potato dextrose agar plate (Nihon Seiyaku, Tokyo, Japan). A part of the mycelia was inoculated into XY medium containing 20 g/L l-arabinose and then aerobically cultured at 28 °C for 48 h shaking at 150 rpm. The activities of LXR were measured by the oxidation of xylitol with NADP+ as a cofactor. Mycelia (43.8 g wet weight) from 4 L of the culture were suspended in 50 mM potassium phosphate buffer (KPB, pH 7.0) containing 5 mM 2-mercaptoethanol (2-ME) and 2 mM MgCl2 (referred to as Buffer A), and a protease inhibitor (EDTA free; Roche, Basel, Switzerland). Cell-free extract was prepared by sonication as described previouslyCitation12) and fractionated with 45–90% (NH4)2SO4 saturation. The redissolved protein solution was dialyzed against 20 mM KPB (pH 7.0) containing 5 mM 2-ME and 2 mM MgCl2 (referred to as Buffer B). The enzyme solution was brought up to 40% (NH4)2SO4 saturation, and the supernatant was applied to a Butyl-Toyopearl column (40 mL; Tosoh Corp., Tokyo, Japan) which had been equilibrated with Buffer B containing 40% saturated (NH4)2SO4. The enzyme was eluted with a linear gradient of 40–0% (NH4)2SO4 saturation in the buffer. The active fractions were dialyzed against Buffer B for 24 h. It was applied to a Gigapite hydroxyapatite column (10 mL; Seikagaku Kogyo, Tokyo, Japan) which had been equilibrated with the same buffer, and then eluted with a linear gradient of 20–300 mM potassium phosphate. The active fractions were dialyzed against Buffer B and then applied to a MonoQ HR 5/5 column (GE Healthcare, Uppsala, Sweden) which had been equilibrated with the same buffer. The enzyme was eluted with a linear gradient of 0–0.15 M NaCl, and then concentrated with ultrafiltration using Centricon YM-10 (Millipore, Darmstadt, Germany). The enzyme solution was applied to a Superdex 200 HR 10/30 column (GE Healthcare) which had been equilibrated with Buffer B containing 0.15 M NaCl, and the active fractions were dialyzed against Buffer B. Amino acid sequences were analyzed by APRO Life Science Institute Inc. (Tokushima, Japan). The molecular masses of the subunits and native enzyme were determined by SDS polyacrylamide gel electrophoresis (PAGE) and gel filtration as described previously.Citation12)
Enzyme assay
The activity of LXR was measured at 25 °C by monitoring the oxidation of NADPH or the reduction of NADP+ at 340 nm. In the reductive reaction, the reaction mixture (1 mL) contained 50 mM KPB (pH 7.0), 50 mM l-xylulose, 0.2 mM NADPH, and an appropriate amount of enzyme. In the oxidative reaction, the reaction mixture (1 mL) contained 50 mM glycine/NaOH buffer, pH 9.6, 100 mM xylitol, 2 mM NADP+, and an appropriate amount of enzyme. One unit of enzyme activity was defined as the amount of enzyme that oxidized 1 μmol of NADPH or reduced 1 μmol of NADP+ per minute under the above conditions. Protein concentrations were determined as described previouslyCitation12) and specific activities are expressed as units of enzyme activity per milligram of protein.
The kinetic parameters were determined by measuring the initial velocities at various concentrations of one substrate at fixed concentrations of the other substrates under standard assay conditions. The maximum velocity (Vmax) and the Michaelis constant (Km) values were calculated using Lineweaver–Burk plots.
Cloning of the l-xylulose reductase gene encoding RpLXR
Genomic DNA and total RNA were prepared from R. pusillus NBRC 4578 as described previously.Citation12) The primers used in this study are listed in Table S1. The partial genomic sequence of the RpLXR gene, Rplxr3, was amplified by PCR using genomic DNA as a template with SP1 and AP1 primers. The nucleotide sequence of the 5′-region of Rplxr3 was determined using the 5′ RACE system for rapid amplification of cDNA ends (Invitrogen, Warrington, UK). PCR was performed using cDNA previously synthesized from RNA with gene-specific primer (GSP) 1 as a template, the abridged anchor primer, and GSP2. Nested PCR was then performed using the PCR product with the abridged universal amplification primer (AUAP) and GSP3. The nucleotide sequences of the 3′-region of Rplxr3 were obtained using the 3′ RACE system for rapid amplification of cDNA Ends (Invitrogen). After amplification of DNA fragments using cDNA as a template with the AUAP and GSP4, nested PCR was performed with AUAP and GSP5. The whole nucleotide sequence of mRNA for Rplxr3 was amplified using cDNA as a template with AUAP, GSP6, and nested GSP7, and then ligated to the pT7Blue vector to yield pTRpLXR. The whole nucleotide sequence of the Rplxr3 gene was amplified with SP2, and AP2 using genomic DNA as a template.
Expression of the l-xylulose reductase gene in E. coli
The whole open reading frame of the Rplxr3 gene encoding RpLXR was amplified using the plasmid pTRpLXR as the template, SP3, and AP3. The PCR product was digested with NdeI and BamHI (792 bp) and was inserted downstream of the T7 promoter in the pET-15b vector to yield pRpLXR, which was then used to transform E. coli BL21 (DE3) cells.
E. coli BL21 (DE3) cells harboring pRpLXR was cultured in LB medium with 50 μg/mL of ampicillin at 30 °C for 15 h at 150 rpm. After the addition of IPTG at a concentration of 0.5 mM, cells were cultured at 30 °C for 5 h. Harvested cells were suspended in five volumes of Buffer A and then disrupted by sonication at 4 °C for 20 min. After centrifugation, the resulting supernatant was prepared as a cell-free extract. The recombinant RpLXR (rRpLXR) with a His6-tag at the N-terminus was purified from the cell-free extract by immobilized metal ion affinity chromatography with the Profinia protein purification system (Bio-Rad, CA, USA) and then dialyzed against Buffer B.
Quantitative real-time PCR
Culture samples for real-time PCR analysis were collected from 500 mL of XY medium containing 20 g/L of l-arabinose, l-xylose, glycerol, d-mannitol, or d-glucose as a carbon source after being incubated at 28 °C while shaking at 150 rpm. Total RNA and cDNA were prepared as described previously.Citation10) Primers SP4 and AP4 were designed from the Rplxr3 mRNA sequence. The β-actin gene, act1, from R. pusillus NBRC 4578 was used as an internal control. Real-time PCR was performed as described previously.Citation12)
Results and discussion
Purification and characterization of RpLXR
The native RpLXR protein was purified to homogeneity (560-fold) with an overall yield of 5.5% from the cell-free extract of the strain by (NH4)2SO4 fractionation followed by hydrophobic, hydroxyapatite, ion-exchange, and gel-filtration column chromatographies (Table ). The purified RpLXR protein catalyzed the oxidation of xylitol with NADP+ as a cofactor at 57.8 U/mg and the reduction of l-xylulose with NADPH as a cofactor at 53.9 U/mg under standard assay conditions. The enzyme displayed a single band on SDS-PAGE with a Mr of 29,000 (Fig. ) and gel-filtration chromatography of the native enzyme revealed an apparent Mr of 98,000, indicating that RpLXR forms a homotetramer.
Table 1. Purification steps of native RpLXR.
Fig. 2. SDS-PAGE of RpLXR during purification. Lanes. 1, Molecular-weight standards; 2, Cell-free extract; 3, Ammonium sulfate fractionation (50–90%); 4, Butyl-Toyopearl column chromatography; 5, Gigapite column chromatography; 6, MonoQ HR 5/5 column chromatography; 7, Superdex 200 HR 10/30 column chromatography.
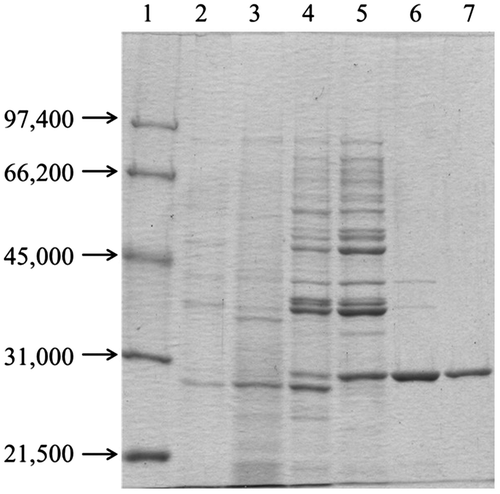
Substrate specificity of the purified RpLXR is summarized in Table . Enzyme activity was clearly observed with l-xylulose, DHA, diacetyl, and dl-glyceraldehyde in the reductive reaction. RpLXR catalyzed the oxidative reaction of xylitol and glycerol, but did not that of d-mannitol or d-sorbitol. In the reductive reaction, Km and Vmax values for l-xylulose were 8.71 mM and 106 U/mg, and that for DHA were 3.89 mM and 224 U/mg, respectively. Enzyme activity toward l-xylulose with NADH was 3.1% of that with NADPH. No enzyme activity was detected when using d-xylose or d-fructose as the substrate. In the oxidative reaction, Km and Vmax values for xylitol were 253 mM and 301 U/mg, and that for glycerol were 423 mM and 99.9 U/mg, respectively. The maximum activities toward xylitol in the oxidative and l-xylulose in the reductive reaction were observed at pH 9.0 and 6.0, respectively (data not shown). Throughout the purification, MgCl2 was added to the buffer to maintain the LXR activity. The activity was decreased to 8% by dialysis with 20 mM KPB (pH 7.0) containing 5 mM 2-ME, and was fully restored following incubation with MgCl2, ZnCl2, or MnCl2, indicating that divalent cations were required for the enzyme activity (data not shown).
Table 2. Substrate specificity of the native RpLXR.
The properties of RpLXR, native LXR from A. niger (AnLXR),Citation5) recombinant LXRs from A. niger (rAnLXR)Citation7) and H. jecorina (rHjLXR)Citation9) expressed in E. coli and from the yeast Ambrosiozyma monospora (rAmLXR) expressed in Saccharomyces cerevisiaeCitation16) are summarized in Table . Native RpLXR exhibited a strict preference for NADPH as a cofactor as well as other LXR except for rAmLXR. The Mr of the subunit, and the Vmax and Km values in catalyzing the reduction of l-xylulose for RpLXR were similar to those for the other LXRs from other fungi.
Table 3. Comparison of l-xylulose reductase from several fungi.
Interestingly, RpLXR exhibited not only a l-xylulose reductase activity but also a strong dicarbonyl reductase activity (Km = 3.89 mM for DHA) like dicarbonyl/l-xylulose reductases (DCXRs) (Km = 1.6–60 mM for DHA) from mammals, e.g. mice, rats, hamsters, guinea pigs, and humans, which also belong to the SDR superfamily.Citation17) On the other hand, it was reported that dihydroxyacetone reductase (NADP+-dependent glycerol 2-dehydrogenase; GLD; 1.1.1.156) from M. javanicus (syn. M. circinelloides; MjGLD) has been characterized,Citation18,19) and the enzymological properties were similar to those of RpLXR (data not shown). It is unclear whether MjGLD were identical to RpLXR, however, GLDs from A. niger and A. nidulansCitation20) did not catalyze the reductive reactions of l-xylulose to xylitol, and so we hypothesized that RpLXR differed from the general GLDs.
Cloning and characterization of the LXR gene
In order to obtain the sequence of the Rplxr3 gene encoding RpLXR, the amino acid sequences of the purified native RpLXR were analyzed. Two internal amino acid sequences were determined to be TELLDITLK and EAVDQIYKDFGA. The sequence of the Rplxr3 gene were identified using PCR with primers designed from the internal amino acid sequences and that conserved among LXRs from fungi, yeast, and mammals (Table S1). The sequence of the Rplxr3 gene and mRNA are available in the GenBank databases as accession numbers LC081188 and LC081189, respectively. The Rplxr3 gene cDNA consists of 792 bp and codes for a protein of 263 amino acids. Its molecular weight was estimated to be 28,341, which agrees with the subunit Mr of 29,000 obtained from the SDS-PAGE analysis of the purified native RpLXR. The coding region of the Rplxr3 gene was interrupted by three introns of 67, 54, and 62 bp. All of these introns started with GT at the 5’-end and ended with AG at the 3’-end, which correspond to consensus sequences for known eukaryotic introns.
The expressed rRpLXR in E. coli BL21 (DE3) cells was purified with a recovery of 26% using an Ni-chelating affinity column. The rRpLXR protein exhibited NADPH-dependent reductase activity as well as native RpLXR. The Km and Vmax values for l-xylulose with NADPH were 13.2 mM and 83.2 U/mg, and that for xylitol with NADP+ of rRpLXR were 225 mM and 109 U/mg, respectively. The Km values for l-xylulose and xylitol were similar between native RpLXR and rRpLXR, indicating that the Rplxr3 gene encoded RpLXR.
According to the BLAST program (www.ncbi.nlm.nih.gov), the primary structure of the 263 amino acid protein deduced from the ORF of the Rplxr3 gene exhibits 99% identity to the hypothetical protein HMPREF1544_00552 from M. circinelloides f. circinelloides 1006PhL (GenBank accession No. EPB92539) and putative 3-oxoacyl-(acyl-carrier-protein (ACP) reductase from M. ambiguus NBRC 6742 (GenBank accession No. GAN06435). The amino acid sequence of RpLXR also showed similarity to LXRs from H. jecorina (41% identity) and A. niger (27% identity), and MDH from H. jecorina (41% identity) (Table ). The phylogenetic tree constructed for RpLXR, and other fungal LXRs, GLDs, and MDHs, and mammalian DCXRs revealed three distinct clades (Fig. ). RpLXR shares the clade with other LXRs except for AnLXR, and clearly differs from DCXRs and GLDs. These results show that RpLXR is distinct from fungal GLDs and support the differences observed in substrate specificities and kinetic parameters between RpLXR and GLDs.
l-Arabinose catabolism in R. pusillus NBRC 4578
R. pusillus NBRC 4578 cannot produce ethanol from l-arabinose, but can utilize l-arabinose.Citation13) Since this strain also utilizes d-mannitol and glycerol as carbon sources, the expression level of the Rplxr3 gene in this strain was analyzed using cultures with l-arabinose, d-xylose, d-mannitol, glycerol, and d-glucose as carbon sources. The Rplxr3 gene was strongly expressed only on l-arabinose, was slightly expressed on d-xylose, d-mannitol, and glycerol, and was completely inhibited on d-glucose (Fig. ). These findings clearly indicate that RpLXR is an l-arabinose-inducible enzyme and involved in the l-arabinose catabolism pathway in this strain.
Fig. 4. Expression of the Rplxr3 gene in R. pusillus NBRC 4578 cultured on d-glucose (▼), l-xylose (●), l-arabinose (○), d-mannose (□), and glycerol (◊). The expression level of mycelia cultivated for 24 h on L-arabinose set as 1.0.
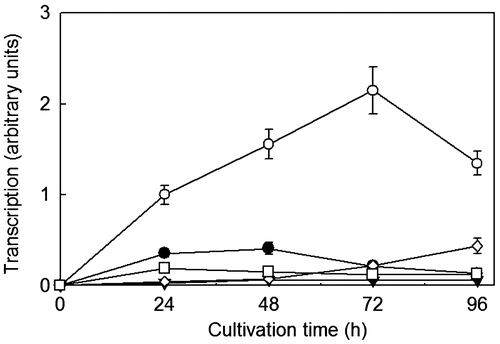
We have already revealed that R. pusillus NBRC 4578 possesses enzymes related to l-arabinose catabolism, NADPH-dependent RpLXR and NAD+-dependent RpXDH.Citation12) In addition, NADPH-dependent RpXR catalyzed not only l-xylose (100%) but also l-arabinose (78.6%) and the expression of xyl1 encoding RpXR was up-regulated on both d-xylose and l-arabinose, indicating that RpXR might also catalyze l-arabinose reduction in the l-arabinose catabolism pathway.Citation13) In addition, RpXDH did not act on l-arabitol, suggesting that this pathway also requires LAD.
Notably, we previously reported that l-arabinose was exhausted after 120 h in cultivations containing 20 g/L l-arabinose, while l-arabitol accumulated at a high concentration after 96 h.Citation13) If this strain possesses NAD+-dependent LAD as well as other fungi, this catabolic pathway results in an imbalance in redox cofactor arising from different coenzyme specificities and an inefficient catabolism of l-arabinose. It is also possible that LAD activity is not sufficient for the rapid l-arabinose catabolism and ethanol fermentation. In order to determine why this strain ferments d-xylose but not l-arabinose, further studies on LAD properties and the redox cofactor balance in l-arabinose catabolism are needed.
Conclusions
In the present study, we purified and characterized NADPH-dependent l-xylulose reductase from the zygomycetous fungus R. pusillus NBRC 4578 (RpLXR). This enzyme exhibited broad substrate specificity toward substrates including l-xylulose and diacetyl, similar to mammalian DCXRs. The expression of Rplxr3, the gene encoding RpLXR, was induced by culture on l-arabinose, but not on d-glucose, d-xylose, d-mannitol, or glycerol, indicating that the enzyme is involved in the l-arabinose catabolic pathway.
The low rate of l-arabinose catabolism and deficiency in ethanol production from l-arabinose of this strain may be caused by an imbalance in the coenzymes involved in the l-arabinose catabolic pathway or low activity of LAD. Recombinant yeast expressing enzymes with altered coenzyme preferences involved in the d-xylose catabolic pathway have been created for efficient ethanol production from lignocellulosic biomasses.Citation21,22) Future studies to identify the other enzymes involved in the l-arabinose catabolic pathway and to develop novel l-arabinose-fermenting strains with altered coenzyme preferences will be of great value.
Author contributions
S.Y.Y. and H.K. designed the research. S.Y.Y. performed the experiments, analyzed the data, and wrote the manuscript. K.H. found the pentose-fermenting ability of this strain. H.K., K.H, and Y.A. reviewed the data and manuscript.
Disclosure statement
No potential conflict of interest was reported by the authors.
Supplemental materials
Supplemental material for this article can be accessed at https://doi.org/10.1080/09168451.2017.1320518.
TBBB_1320518_Table_S1.doc
Download MS Word (79.5 KB)Acknowledgments
We gratefully acknowledge support for this study by a grant from NEDO (New Energy and Industrial Technology Development Organization of Japan).
Additional information
Funding
References
- Akel E, Metz B, Seiboth B, et al. Molecular regulation of arabinan and L-arabinose metabolism in Hypocrea jecorina (Trichoderma reesei). Eukaryot Cell. 2009;8:1837–1844.10.1128/EC.00162-09
- Witteveen CFB, Busink R, van de Vondervoort P, et al. L-Arabinose and L-xylose catabolism in Aspergillus niger. J Gen Microbiol. 1989;135:2163–2171.
- Mojzita D, Penttilä M, Richard R. Identification of an L-arabinose reductase gene in Aspergillus niger and its role in L-arabinose catabolism. J Biol Chem. 2010;285:23622–23628.10.1074/jbc.M110.113399
- Chiang C, Knight SG. L-arabinose metabolism by cell-free extracts of Penicillium chrysogenum. Biochim Biophys Acta. 1961;46:271–278.10.1016/0006-3002(61)90750-8
- Witteveen CFB, Weber F, Busink R, et al. Isolation and characterization of two xylitol dehydrogenases from Aspergillus niger. Microbiology. 1994;140:1679–1685.10.1099/13500872-140-7-1679
- Mojzita D, Vuoristo K, Koivistoinen OM, et al. The ‘true’ L-xylulose reductase of filamentous fungi identified in Aspergillus niger. FEBS Lett. 2010;584:3540–3544.10.1016/j.febslet.2010.06.037
- Richard P, Putkonen M, Väänänen R, , et al. The missing link in the fungal L-arabinose catabolic pathway: identification of the L-xylulose reductase gene. Biochemistry. 2002;41:6432–6437.10.1021/bi025529i
- Metz B, de Vries RP, Polak S, et al. The Hypocrea jecorina (syn. Trichoderma reesei) lxr1 gene encodes a L-mannitol dehydrogenase and is not involved in L-arabinose catabolism. FEBS Lett. 2009;583:1309–1313.10.1016/j.febslet.2009.03.027
- Metz B, Mojzita D, Herold S, et al. A novel L-xylulose reductase essential for L-arabinose catabolism in Trichoderma reesei. Biochemistry. 2013;52:2453–2460.10.1021/bi301583u
- Millati R, Edebo L, Taherzadeh MJ. Performance of Rhizopus, Rhizomucor, and Mucor in ethanol production from glucose, xylose, and wood hydrolysates. Enzyme Microbiol Technol. 2005;36:294–300.10.1016/j.enzmictec.2004.09.007
- Abedinifara S, Karimia K, Khanahmadic M, et al. Ethanol production by Mucor indicus and Rhizopus oryzae from rice straw by separate hydrolysis and fermentation. Biomass Bioenergy. 2009;33:828–833.10.1016/j.biombioe.2009.01.003
- Yamasaki-Yashiki S, Komeda H, Hoshino K, et al. Molecular analysis of NAD+-dependent xylitol dehydrogenase from zygomycetous fungus Rhizomucor pusillus and reversal of the coenzyme preference. Biosci Biotechnol Biochem. 2014;78:1943–1953.10.1080/09168451.2014.943646
- Komeda H, Yamasaki-Yashiki S, Hoshino K, et al. Identification and characterization of L-xylose reductase involved in pentose catabolism of the zygomycetous fungus Rhizomucor pusillus. J Biosci Bioeng. 2015;119:57–64.10.1016/j.jbiosc.2014.06.012
- Takano M, Hoshino K. Direct ethanol production from rice straw by coculture with two high-performing fungi. Front Chem Sci Eng. 2012;6:139–145.10.1007/s11705-012-1281-6
- Komeda H, Yamasaki-Yashiki S, Hoshino K, et al. Identification and characterization of L-xylulokinase from the L-xylose-fermenting fungus, Mucor circinelloides. FEMS Microbiol Lett. 2014;360:51–61.10.1111/fml.2014.360.issue-1
- Verho R, Putkonen M, Londesborough J, et al. A novel NADH-linked L-xylulose reductase in the L-arabinose catabolic pathway of yeast. J Biol Chem. 2004;279:14746–14751.10.1074/jbc.M312533200
- Nakagawa J, Ishikura S, Asami J, et al. Molecular characterization of mammalian dicarbonyl/L-xylulose reductase and its localization in kidney. J Biol Chem. 2002;277:17883–17891.10.1074/jbc.M110703200
- Dutler H, Van der Baan JL, Hochuli E, et al. Dihydroxyacetone reductase from Mucor javanicus. 1. Isolation and properties. Eur J Biochem. 1977;75:423–432.10.1111/ejb.1977.75.issue-2
- Hochuli E, Taylor KE, Dutler H. Dihydroxyacetone reductase from Mucor javanicus. 2. Identification of the physiological substrate and reactivity towards related compounds. Eur J Biochem. 1977;75:433–439.10.1111/ejb.1977.75.issue-2
- Schuurink R, Busink R, Hondmann DH, et al. Purification and properties of NADP+-dependent glycerol dehydrogenases from Aspergillus nidulans and A. niger. J Gen Microbiol. 1990;136:1043–1050.10.1099/00221287-136-6-1043
- Watanabe S, Abu Saleh A, Pack SP, et al. Ethanol production from xylose by recombinant Saccharomyces cerevisiae expressing protein-engineered NADH-preferring xylose reductase from Pichia stipitis. Microbiology. 2007;153:3044–3054.10.1099/mic.0.2007/007856-0
- Bengtsson O, Hahn-Hägerdal B, Gorwa-Grauslund MF. Xylose reductase from Pichia stipitis with altered coenzyme preference improves ethanolic xylose fermentation by recombinant Saccharomyces cerevisiae. Biotechnol Biofuels. 2009;2:9. DOI:10.1186/1754-6834-2-9.